
Effects of additives on corrosion and wear resisitance of micro-arc oxidation coatings on TiAl alloy
WU Xiang-qing(吴向清), XIE Fa-qin(谢发勤), HU Zong-chun(胡宗纯), WANG Li(王 立)
School of Aeronautics, Northwestern Polytechnical University, Xi’an 710072, China
Received 15 July 2009; accepted 11 January 2010
Abstract: Ceramic coating was deposited on TiAl alloy substrate by micro-arc oxidation (MAO) in a silicate-aluminate electrolyte solution with additives including sodium citrate, graphite and sodium tungstate. The microstructures and compositions were analyzed by SEM, EDX and XRD.The corrosion and wear properties of the coatings were investigated by potentiodynamic polarization and ball-on-disc wear test, respectively. The results show that the MAO coatings consist of WO3, Ti2O3, graphite and Al2O3 besides Al2TiO5 and Al2SiO5. With additives in the electrolyte, the working voltage at the micro-arc discharge stage decreases, and the ceramic coating gets smoother and more compact. The corrosion current density of MAO coating is much lower than that of TiAl substrate. It can be reduced from 9.81×10-8 A/cm2 to 3.02×10-10 A/cm2. The MAO coatings composed of hard Al2O3, WO3 and Ti2O3 obviously improve the wear resistance of TiAl alloy. The wear rate is -3.27×10-7 g/(N?m).
Key words: TiAl alloy; micro-arc oxidation coating; additive; corrosion resistance; wear resistance
1 Introduction
TiAl alloys attract much attention as high temperature structural materials in the fields of aerospace and automobile, such as important parts of rocket, automobile, aeroplane and space shuttle, due to their high specific strength, high melting point and excellent corrosion resistance. However, TiAl alloys show poor oxidation resistance at high temperature, because the oxidation scale formed on the TiAl surface is not a continuous Al2O3 layer but a mixed scale of Al2O3 and TiO2 at elevated temperature, which is non-protective and exhibits a high oxidation rate[1]. Therefore, improving the oxidation resistance of TiAl alloys is the key problem. During the past decades, a series of surface modification techniques have been developed[2-7], but it is still worthy exploring more effective methods to enhance the oxidation resistance of TiAl alloys.
Micro-arc oxidation (MAO) is a distinguished technique to fabricate a ceramic coating on light alloys such as Al, Mg and Ti[8]. Under arc discharge at high voltage on the anodic surface, a dense ceramic coating grows in situ on the surface of TiAl alloys by plasma chemistry and electrochemistry reaction. Wear resistance, high temperature corrosion resistance and thermal shock resistance of TiAl alloys have been greatly improved [9-11]. Thus, this technique shows a wide prospect for its application. Up to now, most researches have focused on the properties of micro-arc oxidation coatings and the effects of the processing parameters, such as composition of electrolyte, voltage, current density and time. However, few data can be available concerning on the effect of additives on the micro-arc oxidation of TiAl alloys. In this work, a new electrolyte based on a silicate-aluminate electrolyte solution[12] with additives composed of sodium citrate, graphite and sodium tungstate was studied. Structure and composition of MAO coatings on TiAl alloy were analyzed, the corrosion and wear resistance of MAO coating were assessed by the electrochemical tests and wear tests.
2 Experimental
2.1 Materials and coating preparation
A γ-TiAl alloy with nominal composition (mole fraction) of 48%Ti, 48%Al, 2%Cr, 2%Nb was used as the raw material. The uncoated specimens with a size of 30 mm×30 mm×1 mm were polished with 800# SiC abrasive paper. Prior to coating preparation, the specimens were ultrasonically cleaned in acetone and thoroughly dried. The MAO coating was deposited by using an alternating-current system with the maximum power of 20 kW. The TiAl alloy specimen and the wall of the stainless steel container were used as the anode and the cathode, respectively. A solution composed of Na2SiO3, NaAlO2, Na3PO4 and NaF was used as the basic electrolyte solution with pH value of 11-13, and the current density was varied in the range of 5.6-6.7 A/dm2. The MAO coating deposition process was run for 30 min. The electrolyte temperature was controlled within 20-30 ℃. The additives composed of sodium citrate (C6H5Na3O7 4 g/L), graphite (C 3 g/L) and sodium tungstate (Na2WO4·2H2O 3.5 g/L) were added into the basic electrolyte solution to evaluate their effects on the MAO coating. The TiAl alloy substrate, the specimens coated in the basic electrolyte and in the electrolyte with additives were designated as specimens 1#, 2# and 3#, respectively.
2.2 MAO coating characterization and properties
Potentiodynamic polarization curves in 3.5% NaCl solution were measured using a CHI660B electrochemical workstation to evaluate corrosion behaviors of the bare and coated TiAl alloys. A three- electrode cell, with specimens as working electrode, saturated calomel electrode (SCE) as reference electrode and platinum coil as counter electrode, was employed in this test. After 5 min initial delay, scan was conducted from -750 to 2 000 mV at a rate of 1 mV/s.
An AISI 52100 bearing ball with a hardness of HRC 60 and a diameter of 4.75 mm was used as couple materials against MAO coating using a HT-1000 ball-on-disc friction and wear tester under dry sliding. During wear test, a 5 N load and a 0.13 m/s sliding speed at ambient temperature were chosen. The sliding distance was 260 m. The wear rate (Dm) per unit load was calculated by
(1)
where F is the load (N); s is the distance of wear (m); m1 and m2 are mass of specimen before and after abrasion (g), respectively.
The surface morphologies of MAO coatings were observed by a JSM-6360LV scanning electronic microscope (SEM) equipped with energy dispersive spectrometry (EDS). An X-ray diffractometer (XRD, Philips-1700X) with Cu Kα radiation at 30 kV, 20 mA and 4 (?)/min (2θ) scanning rate was used to identify the phases of MAO coatings made in the basic electrolyte solution without and with the additives.
3 Results and discussion
3.1 Characterization of MAO coatings
Fig.1 shows the morphologies of MAO coatings prepared in the solutions with and without additives, respectively. It is found that the micro-arc oxidation coating is porous and loose (Fig.1(a)). By adding additives, the number of pores is obviously reduced, and the surface becomes compact (Fig.1(b)). During the MAO process, the breakdown voltage is reduced from 390 V to 330 V significantly after adding additives into basic electrolyte solution. According to the MAO theory [13], the thickness of coating (d) can be calculated by
d=Aexpk(V-Vb) (2)
where V is the coating-forming voltage, Vb is the breakdown voltage, A and k are two constants. From Eq.(2), to a certain coating-forming voltage, the lower the breakdown voltage is, the thicker the coating is. As the breakdown voltage reduces, the coating-forming voltage and the discharge intensity reduce consequently, which is advantageous to refine the grains and close up the micropores, and makes the number of micropores decrease significantly. BARTON and JOHNSON[14] reported that citrate ions (C5H7O5COO-) help to form controllable moving luminescent sparks and to prevent the forming of pitting corrosion in the micro-arc oxidation process. Therefore, adding the additives into the basic electrolyte solution makes the luminescent sparks on the specimen surface dense and uniform. The ceramic coating gets smoother and more compact, and the roughness decreases consequently.
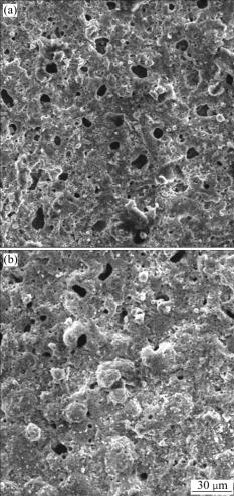
Fig.1 Surface morphologies of MAO coatings on substrates treated for 30 min: (a) Without additives; (b) With additives
The XRD patterns of the MAO coating in different solutions are shown in Fig.2. From Fig.2, without additives, the MAO coating mainly consists of Al2TiO5, Al2SiO5 and TiO2. And with additives, the MAO coating contains WO3, Ti2O3, graphite and Al2O3 corundum phases, besides Al2TiO5 and Al2SiO5.
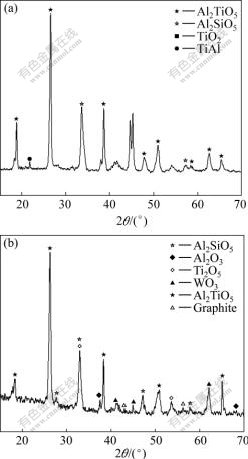
Fig.2 XRD patterns of MAO coating without additives (a) and with additives (b)
During the micro-arc oxidation process, because of micro-arc discharge, WO42- ions are very easily driven to the anode surface, and have a series of chemical reaction with the substrate elements produced by plasma, under high temperature and high pressure. A series of chemical reactions may happen as follows:
WO42-+2Al-2e→WO3+Al2O3+O2↑ (3)
WO42- +2Ti-2e→WO3+Ti2O3+O2↑ (4)
Consequently, O2 results in the following reactions:
2O2+4Al→2Al2O3 (5)
2O2+4Ti→2Ti2O3 (6)
So, adding appropriate additives into the basic electrolyte solution can promote the forming of hard and wear-resistant phases including WO3, Ti2O3 and Al2O3 in the MAO coating.
3.2 Corrosion resistance
Potentiodynamic polarization curves of bare and coated TiAl alloys (with and without additives) are given in Fig.3. The corrosion current density and the corrosion potential got by Tafel method are given in Table 1.
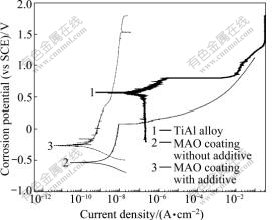
Fig.3 Potentiodynamic polarization curves of TiAl alloy substate and MAO coatings
Table 1 Open circuit potentials and corrosion current densities of different specimens
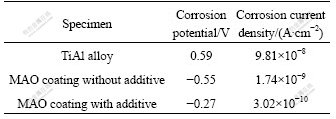
It can be seen from Fig.3 that the corrosion potential of MAO coatings (with additives and without additives) are moved negatively than that of TiAl substrate. The potentiodynamic polarization curves of MAO coatings have an obvious passivation region. The MAO coating with additives shows the lowest corrosion current density of 3.02×10-10 A/cm2, which is almost two orders of magnitude lower than that of TiAl substrate, because the dense MAO coating contains the chemically stable phases, such as Al2TiO5, Al2SiO5 and Al2O3.
The corrosion potential of the MAO coating is more negative than that of TiAl substrate. Once the MAO coating is cracked in the corrosive medium, the MAO coating serves as anode and can still protect TiAl substrate from corrosion. Therefore, the corrosion resistance of coated TiAl alloys by MAO treatment is improved.
3.3 Tribological properties
The variation of friction coefficients (μ) of bare and coated TiAl alloys (with and without additives) with sliding distances under dry friction are shown in Fig.4. The friction coefficient of TiAl substrate is the lowest, and the MAO coating made in basic electrolyte has the highest friction coefficient. The wear rates of three specimens of 1#, 2# and 3# are 9.4×10-7, 28.3×10-7 and -3.27×10-7 g/(N·m), respectively. By adding the additives, the friction coefficient of 3# specimen is lower than that of 2# specimen and the wear rate is the lowest.
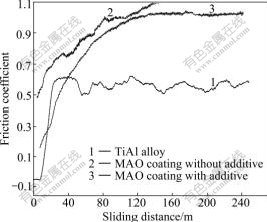
Fig.4 Variation of friction coefficient with sliding distance
The micro-arc oxidization ceramic coating is structured with a porous loose outer layer and a dense inner layer[15]. At the initial stage of friction, the loose layer is easy to be damaged and the friction coefficient increases dramatically. After the loose outer layer is abraded away, the dense inner layer containing hard phases is exposed to contact against the steel counterpart and the friction coefficient varies gently. The dense layer and hard phases in the MAO coatings play an important role.
Fig.5 shows the worn surface of the coated specimen by adding additives after friction test. The element contents of different areas on the worn surface of MAO coating are listed in Table 2. From Fig.5, the worn track of MAO coating appears superficially in peeling off and adhering of transferred layer. EDS indicates Fe dose not present in the unworn MAO coating (Area 1 in Fig.5) but presents in the worn track (Area 2 in Fig.5). Meanwhile, there is a certain content of graphite, but Al and Ti contents in worn track are obviously lower than those in the unworn coating. During dry sliding friction test, the loose outer layer is soon abraded away, then the dense inner layer containing hard phases is exposed to contact against the steel counterpart. Because of the high hardness of the dense inner layer, the surface of the steel counterpart is cut and plastically deformed. The products cut off from the steel counterpart transfer and adhere to the coating surface in the process of repeated rolling. The Fe originated from the steel counterpart presents in the worn track. Because the adhered layer on worn track is greater than the abraded-away loose outer layer, the wear rate of the coating leads to negative growth, namely -3.27×10-7 g/(N?m). Besides, graphite particles deposited in the coating act as an excellent solid lubricant[16-17] during the friction process, making the friction coefficient of coating reduced. Therefore, by adding appropriate additives into the basic electrolyte solution, MAO coating could improve the wear resistance of TiAl alloy.
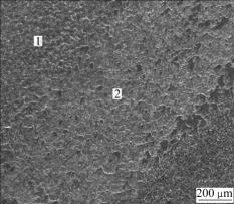
Fig.5 Surface morphology of MAO coating with additives after friction
Table 2 Element contents of different areas on worn surface of MAO coating (mole fraction, %)
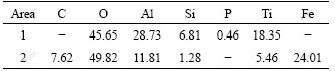
4 Conclusions
1) By adding adequate sodium citrate, graphite and sodium tungstate into the basic electrolyte, the compactness of the MAO coating can be improved. The MAO coating is composed of WO3, Ti2O3, graphite and Al2O3 besides Al2TiO5 and Al2SiO5.
2) The corrosion potential of MAO coating is more negative than that of TiAl substrate. As the anodic film, MAO coating can protect TiAl substrate from corrosion in electrochemical reaction. By adding additives, the corrosion current density of MAO coating is much lower than that of TiAl substrate. It can be reduced from 9.81×10-8 A/cm2 to 3.02×10-10 A/cm2.
3) The wear rate of MAO coating by adding additives is -3.27×10-7 g/(N?m) and the micro-arc oxidation is a promising method to improve the wear resistance of TiAl alloy.
References
[1] YOSHIHARA M, KIM Y W. Oxidation behavior of gamma alloys designed for high temperature applications [J]. Intermetallics, 2005, 13(9): 952-958.
[2] MUKHERJEE S, MAITZ M F, PHAM M T, RICHTER E, PROKERT F, MOELLER W. Development and biocompatibility of hard Ti-based coatings using plasma immersion ion implantation-assisted deposition [J]. Surface and Coatings Technology, 2005, 196(1/3): 312-316.
[3] REINHOLD B, MAIK F, WOLFGANG B, CHRISTOPH L. Oxidation behaviour of gamma titanium aluminides with EB-PVD thermal barrier coatings exposed to air at 900 ℃ [J]. Surface and Coatings Technology, 2007, 202(4/7): 676-680.
[4] WANG Jing, SONG Ren-guo, LIN Xin, HUANG Wei-dong. Investigations of laser cladding TiC-Ti-Al coatings on γ-TiAl intermetallic alloy [J]. Rare Metal Materials and Engineering, 2008, 37(12): 2191-2195. (in Chinese)
[5] LIU Zhen-yu, WANG Guo-dong. Improvement of oxidation resistance of γ-TiAl at 800 and 900 ℃ in air by TiAl2 coatings [J]. Materials Science and Engineering A, 2005, 397(1/2): 50-57.
[6] XIANG Z D, ROSE S R, DATTA P K. Codeposition of Al and Si to form oxidation-resistant coatings on γ-TiAl by the pack cementation process [J]. Materials Chemistry and Physics, 2003, 80(2): 482-489.
[7] FROHLICH M, BRAUN R, LEYENS C. Oxidation resistant coatings in combination with thermal barrier coatings on TiAl alloys for high temperature applications [J]. Surface and Coatings Technology, 2006, 201(7): 3911-3917.
[8] WIRTZ G P, BROWN S D, KRIVEN W M.Ceramic coatings by anodic spark deposition [J]. Materials and Manufacturing Processes, 199l, 6(1): 87-l15.
[9] LI Xi-jin, CHENG Guo-an, XUE Wen-bin, ZHENG Rui-ting, CHENG Yun-jun. Wear and corrosion resistant coatings formed by micro-arc oxidation on TiAl alloy [J]. Materials Chemistry and Physics, 2008, 107(1): 148-152.
[10] LI Xi-jin, WU Xiao-ling, XUE Wen-bin, CHENG Guo-an, ZHENG Rui-ting, CHENG Yun-jun. Structures and properties of ceramic films on TiAl intermetallic compound fabricated by micro-arc oxidation [J]. Surface and Coatings Technology, 2007, 201(9/11): 5556-5559.
[11] HAO Jian-min, YE Yu-de, CHEN Hong, JIE Wan-qi. Study of high temperature property on micro-arc oxidization ceramic coating of TiAl alloy [J]. Journal of Materials Protection, 2005, 38(1): 24-26. (in Chinese)
[12] WANG Li, WU Xiang-qing, XIE Fa-qin, ZHANG Jun. The optimization of silicate solution system for the TiAl alloy micro-arc oxidation [J]. China Surface Engineering, 2008, 21(5): 31-34. (in Chinese)
[13] ALBELLA J M, MONTERO I. Electron injection sand avalanche during the anoxic oxidation of tantalum [J]. Journal of the Electrochemical Society, 1984, 131: 1101-1104.
[14] BARTON T F, JOHNSON C B. The effect of electrolyte on the anodized finish of a magnesium alloy [J]. Plating and Surface Finishing, 1995, 82(5): 138-141.
[15] HAO Jian-min, JIE Wan-qi, CHEN Hong, YE Yu-de. The effect of SiO32- on micro-arc oxidization ceramic layer of TiAl alloy [J]. Rare Metal Materials and Engineering, 2005, 4(9): 1455-1459. (in Chinese)
[16] Institute of Tribology in China Mechanical Engineering Institute. Lubricate Engineering [M]. Beijing: China Machine Press, 1986: 148-153. (in Chinese)
[17] APELFELD A V, BESPALOVA O V, BORISOV A M, DUNKIN O N, GORYAGA N G, KULIKAUSKAS V S, ROMANOVSKY E, SEMENOV S V, SOUMINOV I V.Application of the particle back-scattering methods of the study of new oxide protective coatings at the surface of Al and Mg alloys [J]. Nuclear Instruments and Methods in Physics Research B, 2000, 161/163: 553-557.
Foundation item: Project(2006KG03) supported by the Science and Technology Program of Shannxi Province, China
Corresponding author: WU Xiang-qing; Tel: +86-13572258168; E-mail: wxqwsy@nwpu.edu.cn
DOI: 10.1016/S1003-6326(09)60253-3
(Edited by LI Xiang-qun)