
Preparation of nanoparticles of magnesium hydroxide from bittern
XU Bao-qiang(徐宝强)1, 2, 3, DENG Hua(邓 桦)4, DAI Yong-nian(戴永年)1, 2, 3, YANG Bin(杨 斌)1, 2, 3
1. National Engineering Laboratory for Vacuum Metallurgy, Kunming University of Science and Technology, Kunming 650093, China;
2. Vacuum Metallurgy Key Laboratory for Nonferrous Metal of Yunnan Province, Kunming University of Science and Technology, Kunming 650093, China;
3. Faculty of Materials and Metallurgical Engineering, Kunming University of Science and Technology, Kunming 650093, China;
4. Yunnan Nationality University, Kunming 650031, China
Received 15 July 2007; accepted 10 September 2007
Abstract: Mg(OH)2 nano-particles with fiber-like morphology were prepared by chemical precipitation process, with the bittern from Qinghai Salt Lakes used as Mg2+ raw material and ammonia water as precipitator. The influence of aging procedure on the Mg(OH)2 crystal was investigated by TEM and crystalline size calculation. The Mg(OH)2 nano-particles were characterized by XRD, SEM, TEM and TG-DTA methods. The results indicate that the Mg(OH)2 nano-particles with perfect crystal are obtained after aging procedure above 50 ℃, and the grain size increases with increasing aging temperature and prolonging aging time. Mg(OH)2 particles with fiber-like morphology, 20-60 nm in diameter and 300-500 nm in length are observed, Mg(OH)2 will decompose into MgO at temperature range 347-623 ℃.
Key words: magnesium hydroxide; nano-particles; chemical precipitation; bittern
1 Introduction
The magnesium hydroxide, as an important kind of inorganic materials, has been widely used as neutralizing agent[1], flame retardant[2], smoke suppressant, desulfurizing agent and decolorizing agent[3]. Especially contrasted with other flame retardants (i.e. aluminum hydroxide), Mg(OH)2 has high decomposition temperature, good effects on depressing smoke and neutralizing acid, ect. Fatherly, Mg(OH)2 nanocrystallines as the flame retardant are favorable to reduce the loading level, increase the flame-retardant efficiency and improve the compatibity of Mg(OH)2 and polymer matrixes. So it has gotten more and more attention in recent years. For example, ZOU et al[4] have employed chemical-liquid-deposition method successfully to prepare lamellar-like Mg(OH)2, by using the natural oxidation of magnesium metal under formamide/water mixtures and oxidative conditions. LIANG et al[5] have synthesized Mg(OH)2 nano- structures with wormhole-, tube-, rod- or platelet-like morphologies by pulsed laser ablation of an Mg plate target in deionized water or aqueous solutions of sodium dodecryl sulfate surfactant. DING et al[6] have prepared Mg(OH)2 nanocrystallines with rod-, needle-, tube-, or lamella-like morphologies by a hydrothermal reaction using different magnesium precursors and solvents as raw materials. Fiber-like Mg(OH)2 nanoparticles were synthesized in aqueous solution at room temperature employing microware irradiation using common compounds magnesium nitrate and sodium hydroxide as reactants by WU et al[7]. L? et al[8] have researched on the controlled growth of Mg(OH)2 nanoparticles with needle-, lamellar- and rod-like morphologies through solution precipitation in the presence of complex dispersants. The above-mentioned researches prepared the MH nanoparticles using the chemical reagent MgCl2·6H2O or MgSO4 as Mg2+ reactant, in this study Mg(OH)2 nanoparticles with fiber-like morphology was synthesized using the bittern from Qinghai Salt Lake in China.
2 Experimental
2.1 Raw materials
The composition of the main elements in bittern from Qinghai Salt lakes as the Mg2+ raw material is listed in Table 1. 25% ammonia water (AR), sodium sulfate (AR), and absolute ethanol were used as raw materials. 10% (mass fraction) complex solution of gelatin, lauryl sodium sulfate (1?1) and absolute ethanol was used as dispersant agent [8].
Table 1 Composition of main elements in bittern (g/L)

2.2 Preparation of magnesium hydroxide nanopowders
In order to eliminate the influence of Ca2+ on the following precipitation process, the sodium sulfate, 1.5 times equivalent quantity as much as the quantity of Ca2+ in bittern, was added in bittern solution with continuous agitation at 50 ℃. Several time later, Ca2+ ion was precipitated into Ca(OH)2 precipitation and removed from the bittern solution. 200 mL magnesium salt solution of concentration 50 g/L was gotten by the above bittern solution diluted. The precipitation process was carried out in a three-necked bottle in water bath at 20 ℃. The above magnesium salt, 10 mL absolute ethanol and 8 mL complex solution of gelatin and lauryl sodium sulfate were added into the reactor firstly. Then 25% ammonia water was injected into the three-necked bottle at the discharge of about 2 mL/min with vigorous stirring of 1 000 r/min. The pH value was monitored during the precipitation process. At pH 10, the suspension was aged at different temperatures for times. After aged, the suspension was filtered and washed using dilute ammonia water several times to remove other impure ions, such as Na+, K+, Cl- and NH4+, then the final Mg(OH)2 nanopowders were obtained after dried at 70 ℃ for 24 h in vacuum oven and grinded.
2.3 Characterization of nanopowders
The phase and the crystallographic structure of the samples were characterized by XRD, which was recorded by using a Rigaku (Japan) D/max-3B X-ray diffractometer equipped with graphite-monochromatized Cu Kα radiation and working at 40 kV and 30 mA. Transmission electron microscope (TEM, Hitachi H-800) and scanning electron microscope (SEM, XI30ESEM-TMP model from Philip Company) were used to observe the morphology of the MH nanopowders. And the TG-DTA curves of the MH sample was recorded by SDT2960 TGA-DTA analyzer, from TA company in America, at 10 ℃/min in atmosphere.
3 Results and discussion
3.1 XRD analysis
The phase and crystallographic structure can be identified by XRD pattern of the MH nanoparticle in Fig.1. The powder XRD peaks are consistent with the data of the card 44-1482 in the JCPDS file of Mg(OH)2 with hexagonal phase[9]. The peaks at 2θ values of 18.48?, 32.80?, 37.92?, 50.72?, 58.64?, 62.02? and 68.16? correspond to the crystal planes of (001), (101), (102), (110), (111) and (103) of crystalline MH, respectively. The intensity ratio of 1.98 between reflection [001] and [110] exhibits a igher I001/I110 ratio than that of 1.7 for needle-like morphology reported by L? et al[8], which possibly indicates a more distinct orientation effect in the fiber-like morphology. In addition, the significant broadened peaks indicate that the MH particle has very small grain size. And there are not any additional peaks in Fig.1, showing that the MH sample has high purity.
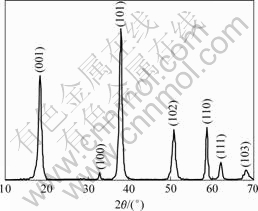
Fig.1 XRD pattern of magnesium hydroxide product (I001/I110=1.98)
3.2 Influence of aging procedure on Mg(OH)2 nano- particles
The aging procedure is very important to prepare Mg(OH)2 nanoparticles with perfect crystal. Fig.2(a) shows the TEM image of Mg(OH)2 particle without aging process and Figs.2(b), (c) and (d) aged at 30 ℃, 50 ℃ and 60 ℃, individually. The particles with imperfect crystal are seen in Figs.2(a) and (b), but the particles with perfect fiber-like crystal are shown in Fig.2(c) and (d). It is well known that the crystal nucleus needs enough energy to overcome its energy barrier. So adopting higher aging temperature after precipitation process is beneficial to supplying enough energy for MH nanocrystal to form perfect crystal nucleus. At the same time, higher aging temperature contributes to the volatilization loss of ammonia and growth of grain size,
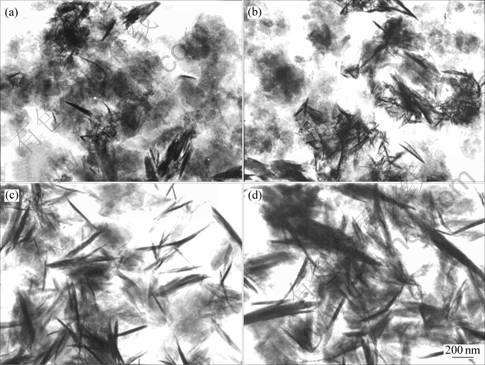
Fig.2 TEM images of magnesium hydroxide powders after different treatments: (a) Without aging process; (b) Aged at 30 ℃; (c) Aged at 50 ℃; (d) Aged at 60 ℃
just as grain size in Fig.2(d) is bigger than that in (c) at length and diameter. In addition, the influence of aging time on crystalline size of MH nanoparticles was investigated. The crystalline sizes of particles synthesized at different aging time were calculated by Derby-Scherrer formula and the results are listed in Table 2. Similar to the influence of aging temperature, the crystalline sizes of crystal planes (101), (102) and (111), respectively, grow with the aging time prolonging, through which the essential energy for crystal growth is obtained.
Table 2 Influence of aging time on crystalline size of magnesium hydroxide
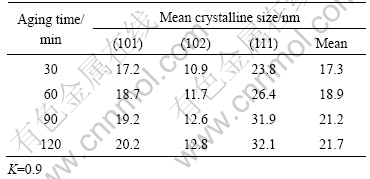
3.3 Size and morphology of Mg(OH)2 nanoparticles
The morphology of MH nanoparticles is shown in Fig.3. Because the conductivity of Mg(OH)2 powders is very low, the surface charge of MH sample
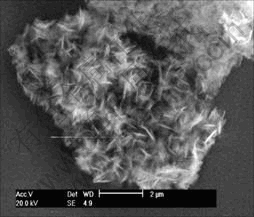
Fig.3 SEM image of magnesium hydroxide sample
accumulates and the surface discharge happens strongly with increasing resolution of image. The clear SEM image of MH particles was obtained after 20 000 times resolution, from which MH nanoparticles existing as agglomerates have seemly branch-like morphology. But fiber-like MH particles with size of 20-60 nm in diameter and 300-500 nm in length, of the same sample, are seen in Fig.4. Some researches indicate that the fiber-like nano-MH particles as flame retardant filled with polymers can improve the flame retardant property[10] and toughness of the compounds.
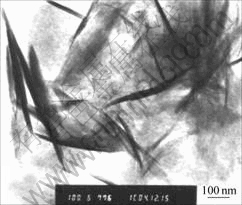
Fig.4 TEM image of magnesium hydroxide sample
3.4 Decomposition behavior of Mg(OH)2 nano-powders
Compared with other inorganic hydroxides, Mg(OH)2 have high decomposition temperature and is a non-toxic and smoke suppressing HFFR additive as flame retardant polymeric materials. TG-TDA curves show the decomposition behavior of the sample MH powders in Fig.5. About 9% of the MH sample is predicted to be loss of adhesive water and crystal water below 336.44 ℃. The decomposition process of Mg(OH)2 to MgO begin at about 347 ℃ and the strongest endothermic peak appears at 377.26 ℃. The rate of mass loss from 90.99% at 336.44 ℃ to 59.95% at 623.25 ℃, over 31% closed to the theoretical one of the reaction Mg(OH)2 to MgO, indicates that the decomposition process have mostly ended. This result is similar to that reported by HALIKIA et al[11].
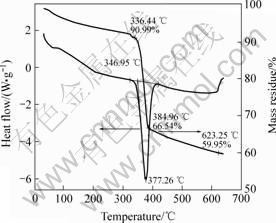
Fig.5 TG-DTA curves of sample magnesium hydroxide
4 Conclusions
1) It is feasible experimentally for the bittern from Qinghai Salt Lakes as Mg2+ raw materials to be used to synthesize nano-Mg(OH)2 particles through chemical precipitation process. Mg(OH)2 nanoparticles have the fiber-like morphology and sizes of 20-60 nm in diameter and 300-500 nm in length.
2) In the preparing process for nano-Mg(OH)2 particles, aging procedure at certain temperature is essential to get nanoparticles with perfect crystal. And perfect crystal were obtained through above 50 ℃ aging procedure.
3) The grain size increases with increasing aging temperature and prolonging aging time. The decomposition process of Mg(OH)2 nanopowders sample to MgO happens from about 347 ℃ to 623 ℃, and the strongest endothermic peak appears at 377.26 ℃.
Acknowledgements
The authors gratefully acknowledge to Professor KAN Jia-de, Professor LIU from Yunnan University and Professor WANG from Kunming University of Science and Technology for their help on the XRD analysis, DTA-DTG analysis, TEM and SEM observations.
References
[1] ZHAI De-wei, CHEN Ai-min, NI Zhe-ming. Preparation of nanocrystalline magnesium hydroxide and using for wastewater containing chromium disposal[J]. Bulletin of Science and Technology, 2007, 23: 141-145.
[2] GUI Hua, ZHANG Xiao-hong, LIU Yi-qun, et al. Effect of dispersion of nano-magnesium hydroxide on the flammability of flame retardant ternary composites[J]. Composites Science and Technology, 2007, 67: 974-980.
[3] ZHU Hong-tao. Study on decolour of reactive brilliant red using magnesium hydroxide[J]. Chemical Engineer, 2007, 3: 55-57.
[4] ZOU Guang-long, LIU Run, CHEN Wei-xiang, XU Zhu-de. Preparation and characterization of lamellar-like Mg(OH)2 nanostructures via natural oxidation of Mg metal in formamide/water mixture[J]. Materials Research Bulletin, 2007, 42: 1153-1158.
[5] LIANG Chang-hao, SASAKI T, SHIMIZU Y, KOSHIZAKI N. Pulsed-laser ablation of Mg in liquids: surfactant-directing nanoparticle assembly for magnesium hydroxide nanostructures[J]. Chemical Physics Letters, 2004, 383: 58-63.
[6] DING Y, ZHANG G, WU H, HAI B, WANG L, QIAN Y. Nanoscale magnesium hydroxide and magnesium oxide powders: control over size, shape, and structure via hydrothermal synthesis[J]. Chem Mater, 2001, 13: 435.
[7] WU Hua-qiang, SHAO Ming-wang, GU Jia-shan, WEI Xian-wen. Microwave-assisted synthesis of fibre-like Mg(OH)2 nanoparticles in aqueous solution at room temperature[J]. Materials Letters, 2004, 58: 2166-2169.
[8] L? Liang-ping, QIU Long-zhen, QU Bao-jun. Controlled growth of three morphological structures of magnesium hydroxide nanoparticles by wet precipitation method[J]. Journal of Crystal Growth[J]. 2004, 267: 676-684.
[9] Joint Committee on Powder Diffraction Standards. Diffraction data file, JCPDS international center of diffraction data[M]. Pennsylvania, 1991.
[10] QIU Long-zhen, XIE Rong-cai, DING Peng, QU Bao-jun. Preparation and characterization of Mg(OH)2 nanoparticles and flame-retardant property of its nanocomposites with EVA[J]. Composite Structures, 2003, 62: 391-195.
[11] HALIKIA I, NEOU-SYNGNOUNA P, KOLITSA D. Isothermal kinetic analysis of the thermal decomposition of magnesium hydroxide using thermogravimetric data[J]. Thermochimica Acta, 1998, 320: 75-88.
(Edited by LONG Huai-zhong)
Foundation item: Project supported by Promoting Program of National Engineering Laboratory for Vacuum Metallurgy
Corresponding author: XU Bao-qiang; Tel: +86-871-5114017-15; E-mail: kmxbq@126.com