J. Cent. South Univ. (2012) 19: 675-681
DOI: 10.1007/s11771-012-1056-8
Airflow characteristics by air curtain jets in full-scale room
YIN Hai-guo(尹海国), LI An-gui(李安桂)
School of Environmental and Municipal Engineering, Xi’an University of Architecture and Technology,Xi’an 710055, China
? Central South University Press and Springer-Verlag Berlin Heidelberg 2012
Abstract: A new air distribution pattern, air curtain jet ventilation was presented. The ventilation or airflow patterns and the air velocity produced by air curtain jet were investigated in detail. To identify the airflow characteristics of this novel air curtain jet ventilation system, a full-scale room was used to measure the jet velocity with a slot-ventilated supply device, with regards to the airflow fields along the vertical wall as well as on the horizontal floor zones. The airflow fields under three supply air velocities, 1.0, 1.5 and 2.0 m/s, were carried out in the full-scale room. The experimental results show the velocity profiles of air distribution, the airflow fields along the attached vertical wall and the air lake zones on the floor, respectively. The current experimental research is helpful for heating, ventilation and air conditioning (HVAC) engineers to design better air distribution in rooms.
Key words: air distribution; air curtain; ventilation; airflow fields
1 Introduction
Air movement within spaces affects the diffusion of ventilation air and, therefore, indoor air quality and comfort. Traditionally, two distinct flow patterns are commonly used to characterize air movement in rooms: entrainment flow and displacement flow [1].
Entrainment flow is also known as conventional mixing flow. The mixing ventilation (MV) systems occupy a large portion of the market, but they have poor ventilation efficiency and are less energy efficient. Since displacement flow system was first applied to a welding industry in 1978 [2], it seemed at the time to be a promising ventilation concept due to its good indoor air quality (IAQ) and saving energy [3]. Displacement ventilation (DV) systems can meet moderate cooling loads (up to 40-50 W/m2); however, this directed supply of cold air and characteristic temperature stratification in the occupied zone may cause thermal discomfort [4]. Therefore, the temperature difference between the supply and room air should be relatively small, which limits DV cooling capacity. For higher cooling capacities, supply airflow rate is considerably increased, and this may turn the stratified flow into mixing ventilation [5]. Another disadvantage of a displacement system is that it can only be used for cooling and is not suitable for winter heating [6]. To overcome this problem, new systems like ceiling mounted textile bag supply and down-to-floor impinging supply have been developed [7].
A new method of air distribution known as the air curtain jet (ACJ) has been developed in this work, which is based on Coanda effect [8] and impinging jet ventilation (IJV) [9]. Generally, the air supply outlet has a large aspect ratio and uses a ceiling mounted slot inlet, and the supply jet direction is downward. If the distance between the jet inlet and the adjacent wall is quite short, the supply jet can be attached to the wall by the Coanda effect. With the jet of high momentum downwards onto the floor, it impinges the wall-floor corner, then separates from the wall and reattaches to the floor surface. The jet spreads over the ground, causing the jet momentum to recede but still have a sufficient force to reach long distances. The resulting flow from the ACJ forms the air lake phenomena over the floor, which is very similar to the displacement ventilation to some extent. Air curtain jet has higher momentum than wall displacement ventilation, but produces a similar flow field to DV system. So, it has promising applications.
In the previous studies, theoretical models have been established and validated to predict the velocity distribution before the jet impinging the wall-floor corner [10]. Furthermore, earlier studies revealed that the air velocity distribution in the corner is nearly symmetric, which means that the maximum jet velocity before the corner is approximately equal to the jet velocity behind it [11]. However, different jet diffusers require rather specific dynamic tests regarding their own characteristics in room conditions.
The main aim of this work is to obtain detailed data and knowledge of the flow field of an air curtain jet in a full-scale room. The specific objectives are:
1) To validate the maximum jet velocity decay model given by Rajaratnam in the vertical wall-attached zone (region I) at slot velocities of 1.0, 1.5 and 2.0 m/s.
2) To establish an efficient model to predict the maximum jet velocity decay in the horizontal floor-attached zone (region II).
3) To verify the self similarity characteristic of the ACJ in regions I and II.
4) To investigate the turbulence intensity of ACJ over the vertical wall and the floor.
2 Air curtain jet modelling
The application of a linear slot diffuser is presented in Fig. 1 and the probable airflow patterns of air curtain jet in rooms are also shown. Meanwhile, based on the Coanda effect and impinging jet ventilation theory, the airflow structure of air curtain jet is shown in Fig. 2.
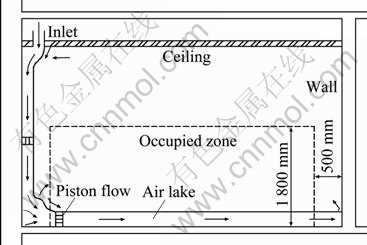
Fig. 1 Probable airflow patterns with use of ceiling mounted slot inlet
In attached plane jet modelling, some theoretical and semi-empirical models were found and validated in the earlier studies.
As Fig. 2 shows, the local maximum jet velocities at separation point and reattachment point are approximately equal, and the separation distance in the corner can be expressed as [12]
(1)
where sy is the jet separation distance along the wall in region I, h is the distance from the slot jet to the floor, and Reslot is the Reynolds number of the jet slot calculated by the width of the jet slot and initial average jet velocity. Likewise, according to Ref. [11], the reattached distance sx in region II is assumed to be approximately equal to sy.
For the maximum jet velocity decay, RAJARATNAM gave the following expression for the attached plane jet velocity prediction [10]:
(2)
where um(y) is the local maximum velocity at distance y, u0 is the average slot velocity, K is the dynamics characteristic parameter of the jet, b is the width of the slot, and y is the distance downstream from the jet slot.
In region I, the maximum jet velocity could be directly calculated by Eq. (2). While in region II, the maximum jet velocity could be calculated by virtual origin model set up by GAO et al [11]:
(3)
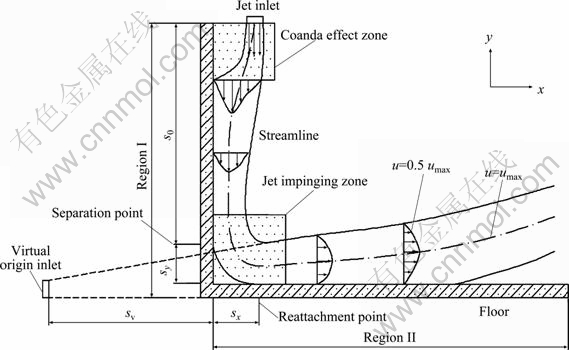
Fig. 2 Airflow structure of air curtain jet
where um(x) is the local maximum velocity after the jet has impinged the corner, x is the distance downstream from the corner, sv is the virtual origin distance, and s0 is the distance from the jet slot to the separation point.
K is the dynamics characteristic parameter of the jet that depends on the Reynolds number of the slot in Eqs. (2) and (3). If the Reynolds number of the slot is less than 104, RAJARATNAM [10] gave a value of 3.5 to K for the attached plane jet. In addition, K=2.6 has also been presented for a jet with a low Reynolds number (79slot<770) proposed in Ref. [11]. Here, K of 3.0 is used in Eqs. (2) and (3), which is derived from the measured velocity data.
In order to study the self similarity characteristic of ACJ, the experimental results obtained from the maximum velocity line measurements (see Fig. 2) will be compared with the empirical equation of VERHOFF for a wall jet [13]:
(4)
where u is the velocity at a distance of y from the jet slot at a different width normal to the wall surface (u x) or at a distance of x from the impinging corner at a different height normal to the floor surface (uy); um is the local maximum velocity at distance y or x, in region I; η is equal to x/x0.5, x is the distance normal to the wall surface, and x0.5 is the distance where the jet velocity is a half of maximum velocity, um(y); in region II, η is equal to y/y0.5.
The turbulence property of the supply airflow plays an extremely important role in the jet flow process. Turbulence intensity is one of the main evaluation indexes of the property. The turbulence intensity could be calculated by the following expression [14]:
(5)
su is standard deviation of the air velocity, and defined as
(6)
where n is the number of measured points at a distance of y from the jet slot or at a distance of x from the impinging corner, ui is the velocity of point i at distance of y or x, and
is the mean velocity of the whole points at distance of y or x.
3 Experimental
3.1 Test chamber and facilities
Velocity field measurements were carried out in a full-scale room (5.4 m×7.0 m×3.16 m). Configuration and dimensions of the test chamber used are shown in Fig. 3. The test chamber consists of a well-insulated wall and the jet supply device (2.5 m×0.5 m×0.5 m). The slot outlet is located in the bottom of the device, with the size of 2.0 m×0.05 m. The inlet aspect ratio is 40. During the measurement, the supply air for the jet was generated by a variable-speed centrifugal fan. The turbulence intensity at the centre of the jet slot was about 0.59%–1.04%.
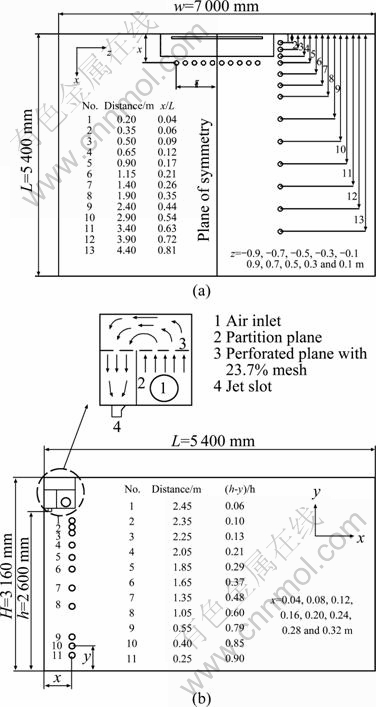
Fig. 3 Layout of test room and distribution of measurement points: (a) Vertical view; (b) Front view and schematic view of air jet supply device
The distributions of the measurement points are also shown in Fig. 3. In the test chamber, the measured points were distributed throughout two regions: the vertical wall-attached zone and the horizontal floor-attached zone. In order to study the three-dimensional characteristics of ACJ, the measurement was carried out in three directions. The measurement scheme used a non-uniform interval in longitudinal direction, but used uniform interval in transverse and radial direction with 0.2 m and 0.04 m between traversing intervals, respectively.
3.2 Measurement conditions
According to the mean air speed recommended by ASHRAE 55—2010 [14] for indoor airflow design, three slot velocities, 1.0, 1.5 and 2.0 m/s, were used to study the behaviors of ACJ. Three different cases were measured in undisturbed isothermal conditions, as presented in Table 1.
Table 1 Experimental conditions in three measured cases for isothermal airflow
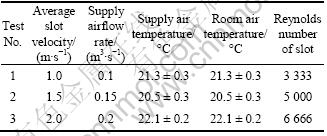
The acquiring period for each measurement was 180 s to ensure accurate time-averaged results for turbulent airflow [15], and the sampling frequency was set at 2 Hz. The average value over time, at a point, was used for analysis and presentation.
3.3 Measuring instrument and accuracy
Air velocity measuring instrument of Swema 3000 was used with an omni-directional draught probe of type SWA 03. The probe has a measurement range of 0.05-3.0 m/s with an accuracy of ±0.03 m/s at 0.05-1.00 m/s and ±3% reading value at 1.00-3.00 m/s. Pressure measuring for supply duct of Swema 3000md was used with a probe of type SWA 10. The probe has a measurement range from -300 to 1 500 Pa with an accuracy of ±1% reading value but the minimum was 0.3 Pa. The Swema 3000 and 3000md instruments both have an upper frequency of 40 Hz, which means that they can collect 40 data in 1 s. Before the measurements were made, all these probes have just been calibrated by manufacturer.
4 Results and discussion
4.1 Maximum jet velocity decay
The measured maximum jet velocity decay and the fitting model are presented in Fig. 4, and the comparison with the earlier maximum jet velocity decay models using Eqs. (2) and (3) is shown. As shown in Fig. 4(a), the maximum jet velocity decay obtained at different slot velocities in region I is plotted for comparison with the empirical equation of Rajaratnam for the attached plane jet. These values at a certain distance from the jet slot of three velocities are very close to each other, while the magnitude of the maximum velocity is proportional to the distance from the slot, as shown in Eq. (7). The measured results have good correlation to Eq. (2) at distance of 0.75-2.35 m (0.29-0.90b) downstream from the jet slot. However, this empirical equation overestimates the maximum velocity at distance of 0.15-0.75 m (0.06-0.29b). The calculated values by Eq. (2) are even larger than slot velocity at the distance of 0.15-0.35 m (0.06-0.13b). Thus, the earlier models could not be used to predict the maximum jet velocity decay of ACJ without any modification at this distance:
(7)
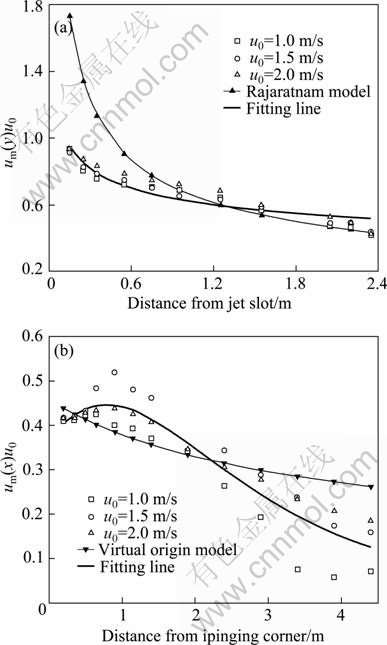
Fig. 4 Maximum velocity decay at different slot velocity: (a) Vertical zone; (b) Horizontal zone
The same procedure was repeated for region II (see Fig. 4(b)), and the virtual origin model can be used to calculate the maximum jet velocity decay at the distance of 1.0-2.9 m (0.19-0.54L) downstream from the impinging corner. However, this model could not predict the speed-up process which happens at the distance of 0.2-1.0 m (0.04-0.19L). In addition, this model tends to incorrectly predict the maximum velocity decay above the horizontal distance of 2.9 m. By contrast, the fitting model based on Giddings function complies very well with the measured velocity in the horizontal floor-attached zone. This model is written as
(8)
where I1 is the modified Bessel function of the first kind.
4.2 Self similarity characteristic
To study the self similarity characteristic of air curtain jet, the measured velocity profiles are collected and compared with the similarity equation Eq. (4). Figure 5 shows that the jet self similarity could only be found in the case of 2.0 m/s slot velocity, but three profiles from the vertical centerline, z=0.1, 0.5 and 0.9 m are used to study the three-dimensional characteristics of ACJ. Figure 5(a) presents the self preserving characteristic in region I at different vertical distances from the floor. In the case of z=0.1 m, at the distance of 2.35-0.55 m, the measured velocity profiles are similar to each other and have good correlation to Eq. (4), while the distortion in velocity distribution for distance of 2.45 m is caused by the attachment of jet due to the Coanda effect as the jet slot is located at a distance of 0.066 m from the wall. At the distance of 0.55 m, a bad agreement is obtained, where it is considered the zone of jet separating from the sidewall. The similarity was also analyzed in case of z=0.5 and 0.9 m. The self similarity can be found in these cases, and this means that the ACJ with a slot aspect ratio of 40 has two-dimensional characteristics and can be treated as a plane jet [16]. In addition, better self similarity of the jet velocity profiles can be found in case of z=0.9 m, especially for the profiles with lower distance from the floor.
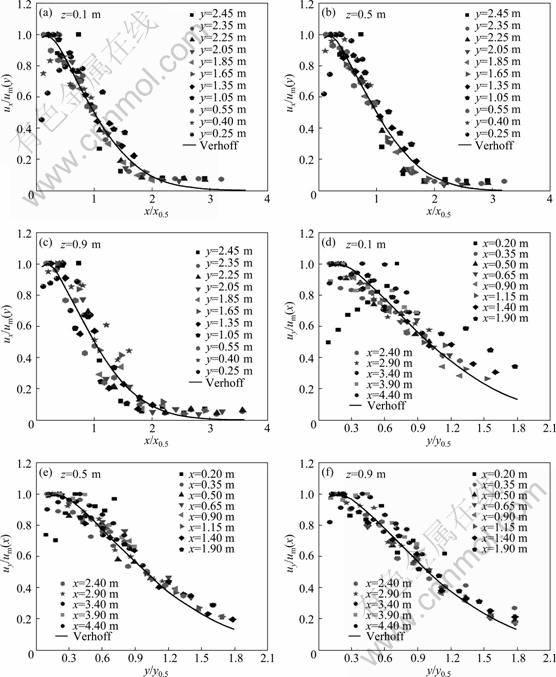
Fig. 5 Non-dimensional jet velocity profiles at different distances: (a)-(c) Vertical zone; (d)-(f) Horizontal zone
For the horizontal floor-attached zone (region II), a similar non-dimensional profile appears at distance of 0.35-3.9 m, but there are different similarity characteristics at the distance of 0.2 and 4.4 m. These zones could be explained as beyond the fully developed zone of a plane jet.
4.3 Turbulence intensity
The transverse velocity at different distances from the jet slot or from the impinging corner in Eq. (5) is applied to calculating the turbulence intensity of ACJ over the vertical wall and the floor, respectively. Figure 6 shows the development of turbulence intensity in air jet flow process. Abscissa value of 0.1-1.0L1 is the change in distance from the jet slot in region I, while the value of 1.0-2.0L2 is the change in distance from the impinging corner in region II.
As shown in Fig. 6, the calculated curves at slot velocities of 1.5 and 2.0 m/s have similar tendency. At the distance of 0-0.1L1 from the jet slot, the jet turbulence intensity has a certain fluctuation, which can be explained as the subsistent distance between the slot and the sidewall, and the air jet attaches to the sidewall in this process. At the distance of 0.1-0.8L1, the jet turbulence intensity stability is in a certain scope, and the mean intensity value is 13.8% and 8.7% for 1.5 and 2.0 m/s, respectively. The jet turbulence intensity has changed considerably at the distance of 0.8-1.0L1 in region I, and at the distance of 1.0-1.2L2 in region II, where the jet separates from the wall, impinges the wall-floor corner, and reattaches to the floor surface. At the later region, the jet turbulence intensity is stationary. In addition, the whole turbulence intensity in region I is higher than that in region II. The mean intensity values for slot velocity of 1.5 and 2.0 m/s are 13.5% and 10.5%, respectively, in region I, while 8.4% and 7.6%, respectively, in region II. The turbulence intensity at slot velocity of 1.0 m/s has behavior similar to other velocities at the distance of 0.1-1.0L1 in region I and 1.0-1.3L1 in region II. However, the jet turbulence intensity at the later points is much larger, and the magnitude of the mean turbulence intensity can increase to a value of 32.2%. The anisotropy of turbulence might be caused by the weaker horizontal spread over the floor and the greater test error at a low slot velocity of 1.0 m/s.
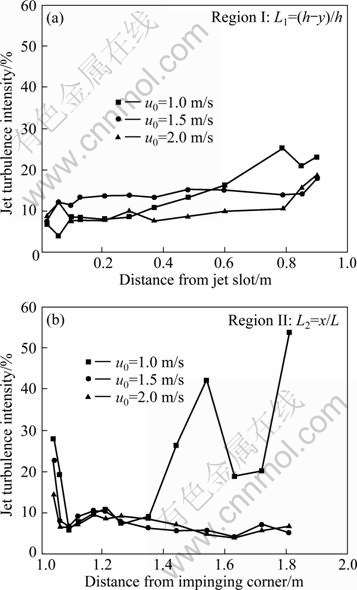
Fig. 6 Variations of jet turbulence intensity at different slot velocities: (a) Vertical zone; (b) Horizontal zone
5 Conclusions
1) The maximum jet velocity decay model given by Rajaratnam is validated and revised in a full-scale room. An efficient model to predict the maximum jet velocity decay after the air curtain jet impinging the wall-floor is established. The model could contribute to airflow design by linear jet diffusers with a large aspect ratio, such as ceiling slot or chilled beams.
2) The velocity profile displays a self similarity characteristic over the vertical wall and the floor. The comparison of different profiles from the vertical centerline shows that the ACJ with a slot aspect ratio of 40 has two-dimensional characteristics and can be treated as a plane jet. However, the characteristic at the impinging and transitional zones is not explicit. More detailed measurement results may be needed before any conclusive statements can be made.
3) The mean turbulence intensity is below 20% for different slot velocities, and the magnitude of average value in horizontal floor zone is lower than that in vertical wall zone. Thus, the air curtain jet also has better spread feature after impinging the corner, and this is helpful to decreasing the local discomfort. However, in air curtain jet, the slot velocity of 1.0 m/s is insufficient to produce a greater horizontal spread over the floor.
References
[1] ASHRAE. 2001 ASHRAE Handbook: Fundamentals [M]. Atlanta: American Society of Heating, Refrigeration and Air-Conditioning Engineers Inc, 2001: F26.2-26.3.
[2] YUAN Xiao-xiong, CHEN Qing-yan, GLICKSMAN L R. A critical review of displacement ventilation [J]. ASHRAE Transactions, 1998, 104(1A): 78-90.
[3] SEPPANEN O. Ventilation strategies for good indoor air quality and energy efficiency [J]. International Journal of Ventilation, 2008, 6(4): 297-306.
[4] CHEONG K W D, YU W J, SEKHAR S C, THAM K W, KOSONEN R. Local thermal sensation and comfort study in a field environment chamber served by displacement ventilation system in the tropics [J]. Building and Environment, 2007, 42(2): 525-533.
[5] HAO Xiao-li, ZHANG Guo-qiang, CHEN You-ming, ZOU Sheng-hua, MOSCHANDREAS D J. A combined system of chilled ceiling, displacement ventilation and desiccant dehumidification [J]. Building and Environment, 2007, 42(9): 3298-3308.
[6] BURT L W. Life cycle cost of displacement ventilation in an office building with a hot and humid climate [D]. Gainesville: University of Florida, USA, 2007: 13.
[7] KARIMIPANAH T, SANDBERG M, AWBI H B. A comparative study of different air distribution systems in a classroom [C]// Proceedings of the ROOMVENT 2000. Oxford: Elsevier, 2000: 1013-1018.
[8] BOSCOIANU M, PRISECARIU V, CIRCIU I. Applications and computational aspects regarding the Coanda effect [J]. Science & Military Journal, 2010, 5(1): 26-30.
[9] KARIMIPANAH T. Turbulent jets in confined spaces [D]. Sweden: Royal Institute of Technology, 1996: 26-35.
[10] RAJARATNAM N. Turbulent jets [M]. Amsterdam: Elsevier, 1976: 23.
[11] CAO Guang-yu, RUPONEN M, KURNITSKI J. Experimental investigation of the velocity distribution of the attached plane jet after impingement with the corner in a high room [J]. Energy and Buildings, 2010, 42(6): 935-944.
[12] CAO Guang-yu, KURNITSKI J, RUPONEN M, MUSTAKALLIO P, SEPPANEN O. Plane air jet corner zone modelling in a room ventilated by an active chilled beam [J]. International Journal of Ventilation, 2008, 7(4): 287-297.
[13] VERHOFF A. The two-dimensional turbulent wall jet without an external free stream [M]. Princeton: Princeton University, 1963: 626.
[14] ASHRAE. ANSI/ASHRAE Standard 55-2010: Thermal environmental conditions for human occupancy [S]. Atlanta: American Society of Heating, Refrigerating, and Air-conditioning Engineers Inc, 2010: 3, 6.
[15] YU H. A modified estimation of a plane wall jet trajectory horizontally diffused from a ceiling slot in non-isothermal ventilated enclosures [J]. Biosystems Engineering, 2006, 95(2): 255-269.
[16] CAO Guang-yu, KURNITSKI J, RUPONEN M, SEPPANEN O. Experimental investigation and modeling of the attached plane jet velocity development characteristics in the transition process in a room [J]. HVAC & R Research, 2009, 15(3): 489-508.
(Edited by YANG Bing)
Foundation item: Projects(50778145, 50278025) supported by the National Natural Science Foundation of China; Project(2009ZDKG-47) supported by “13115” Science and Technology Innovation Program of Shaanxi Province, China
Received date: 2011-07-26; Accepted date: 2011-11-14
Corresponding author: LI An-gui, Professor, PhD; Tel: +86-29-82202507; Fax: +86-29-82202729; E-mail: liag@xauat.edu.cn