
Effect of large cold deformation after solution treatment on precipitation characteristic and deformation strengthening of 2024 and 7A04 aluminum alloys
NING Ai-lin(宁爱林)1, 2, LIU Zhi-yi(刘志义)1, ZENG Su-min(曾苏民)1,2
1. School of Materials Science and Engineering, Central South University, Changsha 410083, China;
2. Department of Machinery Engineering, Shaoyang Institute, Shaoyang 422000, China
Received 2 March 2006; accepted 26 June 2006
Abstract: The effects of large cold deformation after solution treatment on the precipitation characteristic and deformation strength of 2024 and 7A04 Al alloys were investigated. The tensile property tests indicate that the ageing response of the 2024 aluminum alloy is accelerated when treated by cold deformation after solution treatment, and the tensile strength is increased to about 140 MPa while the elongation still keeps above 8%. However, compared with the 2024 alloys, although the aging response of the cold deformed 7A04 aluminum alloy is accelerated, the tensile strength has not changed obviously and the elongation is even decreased drastically. The results of TEM observation show that the S′ phase inside the dislocation cells and at the boundaries of the dislocation cells of the 2024 aluminum alloy has a uniform distribution. But in 7A04 aluminum alloy the club-shaped η′ phase forms at the boundaries of dislocation cells even on dislocation lines, while there still exists small spheric G.P zone in the region with less dislocation. In addition the precipitates in 7A04 alloy with cold rolling present more obvious tendency of growth and coarsening than those without cold rolling. It is indicated that the coherent strain energy of the cylinder formed G.P zones with matrix in the 2024 alloy is smaller than that of the spherical G.P zone in 7A04 alloy, for which a different distribution of precipitates and different effect of strengthening are caused in artificial ageing after resolution treatment and large cold deformation.
Key words: aluminum alloy; aging precipitation; strengthening; cold deformation
1 Introduction
Studies on thermomechanical treatment of aluminum alloy can date from the 1960s[1,2]. A great deal of achievements concerning this study has been obtained after near 40 years of development[3-7]. Among them, the achievement of intermediate thermomechanical treatment is outstanding, such as aiming at solving the problem of the low fracture toughness of 7075 aluminum alloy thick plate in ST direction, refining cast microstructure and eliminating as-casted defects. RUSSO et al[3] and WALDMAN et al[4] have obtained a refined, equiaxial and homogeneous grain structures by adopting a series of thermomechanical treatments as homogenization heat treatment, hot deformation, recrystallization treatment and so on which eliminates the casting defect and ingredient segregation. OANDERS et al[5] have studied the ITMT for enhancing the fatigue properties of the 7075 aluminum alloy. Not only the conventional tensile properties of the alloy are improved, but also the fatigue cracking growth resistance is highly increased by refining the grain structures and eliminating the casting defects. WERT et al[6] have obtained the fine grain structure with 10 μm in L-T direction and 6 μm in T-L direction by employing a series of process as homogenization heat treatment, hot rolling, solution treatment, overaging treatment, low temperature rolling deformation and recrystallization treatment. And PATON et al[7] have used this technique of process to treat 7475 aluminum alloy, and acquired superplastic elongation as high as 525%. Until recently EL-BARADIE et al[8] have obviously enhanced the mechanical properties of 7075 alloy in rolling state of thick plate still by adopting two-step thermomechanical heat treatment. Now the achievements of these ITMT have been widely used. And the final thermomechanical treatment has also acquired a series of progress since the pioneer research work of MCEVILY et al[2] and his co-workers. In Ostermann’s work[9], an increase of 25% in the fatigue stress of 107 cycles-to-failure in 7075 aluminum alloys was achieved by thermomechanical treatment. The thermomechanical treatment employing step-aging was studied by RUSSO et al[10], and better ductility and fracture toughness were obtained. The research of RACK [11] and CHIA and STRARKE[12] indicated that a desirable microstructure for good fatigue properties was fine equiaxed grains with a uniform dislocation distribution which was strongly pinned by fine precipitates. Whereas, only a few of state of final thermomechanical treatment have been applied in practical process of aluminum alloy, such as heat treatment state of T8 of 2024,2219 and T9 of 2A12 alloys[13], in which the deformation applied is relatively small, such as the deformation in the state of T851 and T87 treatment is only 5% and 7%, respectively, for which the reason is to avoid the introduction of the non-uniform distribution of dislocation cell structures which may act as the site of nucleation of the precipitations[11,12]. However, in Refs.[14,15], a significant increase of the strength was achieved by relatively large cold deformation after solution treatment. STOLYARON et al[16] suggested that the G.P zones of main strengthening phase η′ didn’t heterogeneously nucleate on the dislocations. It is suggested that the heterogeneous nucleation of precipitates at the dislocation has not been completely understood. In order to further understand this problem, the behavior of heterogeneous nucleation at dislocations of the S′ and η′ and its strengthening effect of commercial 2024 and 7A04 aluminum alloy have been discussed in this study by using tensile properties tests and microstructure investigation.
2 Experimental
The experimental materials are 2024 and 7A04 aluminum alloys, the chemical compositions are listed in Table 1. 2024 aluminum alloy and 7A04 aluminum alloy are treated by the process listed in Table 2. The as-received materials of the 2024 aluminum alloy are rolled to 4 mm in thickness at 250 ℃ after solution and over-aging treatment, then solution treatment, and cold rolled to 2 mm in thickness, finally aged at 190 ℃ for different times. The as-received materials of the 7A04 aluminum alloy are rolled to 4 mm in thickness at 400 ℃ after solution and over-aging treatment, followed by resolution treatment. Then the materials are divided into two groups. One group is directly aged at 120 ℃ for different time. The other group is rolled to 2 mm in thickness, and finally aged at 120 ℃ for different time. The solution treatment was performed on an electric resistance furnace with temperature fluctuation within ±1 ℃ controlled by a potential difference meter. The samples were quenched into water at room temperature within 5 s. Over-aging and single-step aging were performed on a hot-air- circulating furnace with temperature fluctuation within ±1 ℃ controlled by mercury thermometer. The aging treated plates were processed to compact test specimen. The tensile properties (σb, σ0.2 and δ5) of different heat treatment conditions were measured on a CSS-4400 test machine. Dislocation structure, boundary configuration and structure of precipitation phases were investigated using H-800 transmission electron microscope.
3 Results
The results of the tensile properties tests are shown in Figs.1 and 2. Compared with the peak strength (440
Table 1 Chemical compositions of 2024 and 7A04 aluminum alloys (mass fraction, %)

Table 2 Testing process and parameter of 2024 and 7A04 aluminum alloys
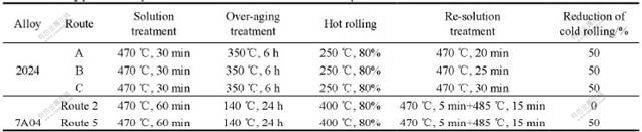
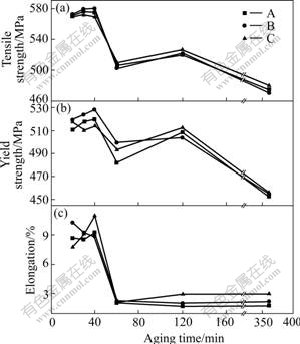
Fig.1 Mechanical properties of 2024 alloys after Routes A, B and C treatments
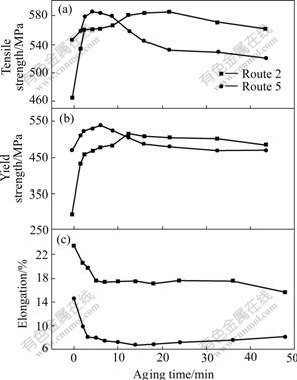
Fig.2 Mechanical properties of 7A04 alloys after Routes 2, 5 treatments
MPa) and elongation (9.2%) of 2024 alloy after peak aging treatment (T62), the peak strength and elongation after thermomechanical treatment are up to 580 MPa and 9.2%, respectively. It can be seen from Fig.2 that although the yield strength increase slightly, the tensile strength of 7A04 aluminum alloy after solution and cold deformation treatment does not increase and the corresponding elongation decrease obviously. Figs.1 and 2 also show that the aging response of the alloy is accelerated by cold deformation after solution treatment. SEM observation shows that the 2024 aluminum alloy exhibits a typical dimple-ductile fracture behavior (Fig.3), while the 7A04 aluminum alloy treated by two different processes shows a ductile fracture behavior, but there are some shear-plateaus on the fracture surface, especially on the surfaces of the specimen treated by route 5 (Figs.3(b) and (c)). High density of dislocation cells is introduced into both 2024 and 7A04 aluminum alloys after solution treatment by cold deformation (Fig.4). It is shown that the S′ precipitates nucleate and grow to the same size and space whether in the high density of dislocation regions
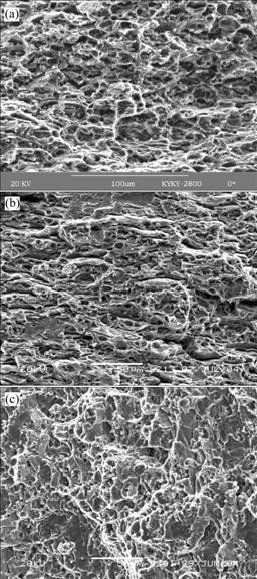
Fig.3 SEM images tensile fracture of two alloys after different treatments: (a) 2024 alloy+50%cold rolling (Route B); (b) 7A04 alloy (Route 2); (c) 7A04 alloy+50%cold rolling (Route 5)
or the low density of dislocation regions in the 2024 aluminum alloy by magnified observation (Figs.5(a) and (b)). Whereas, η′ precipitates in the 7A04 aluminum alloy nucleate and grow at the dislocation lines and the boundaries of the dislocation cells (Figs.6(a) and (b)). Meanwhile, in the low density of dislocation regions the spherical G.P zones still exist. When aged at 120 ℃ for 48 h, η′ phase has become coarse and η equilibrium phase has presented in samples of 7A04 alloy with cold rolling in 50% reduction, while small spheric and homogenous G.P zone is still observed in samples of 7A04 alloy without cold rolling, as shown in Figs.7(a) and (b).
4 Analysis and discussion
The results of the tensile properties test show that the aging strength of the 2024 aluminum alloy treated by cold deformation after solution treatment is up to 580 MPa, which is about 140 MPa higher than the strength of the T62 condition, and corresponding elongation is 9.2% (Fig.1). Although the yield strength increase slightly and
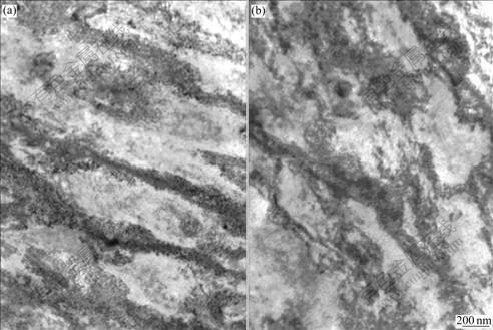
Fig.4 Dislocation structures of different alloys: (a) 2024 alloy, solution treatment+50% cold rolling+190 ℃ aging for 20 min; (b) 7A04 alloy, solution treatment+50% cold rolling+120 ℃ aging for 120 min
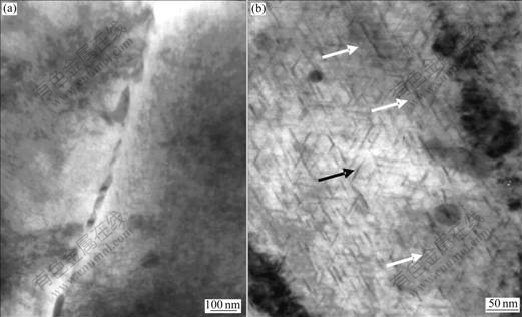
Fig.5 TEM images of 2024 alloy at different aging stages (Route B): (a) 190 ℃, 60 min; (b) 190 ℃, 360 min
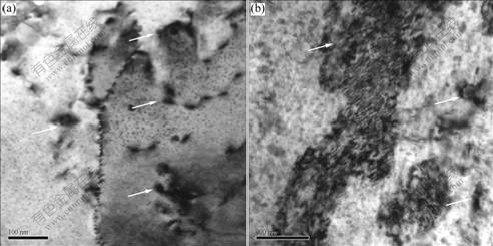







Fig.6 Nucleation and growth of η′ phase at different site at dislocation lines (7A04 alloy, route 2) (a) and dislocation cell (7A04+50%cold rolling, route 5) (b)
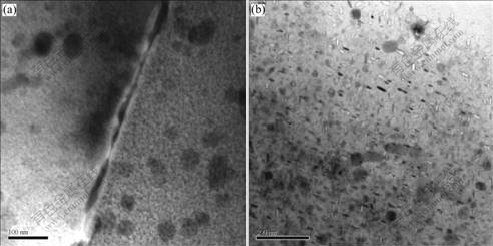
Fig.7 Precipitates in 7A04 alloy under different conditions (120 ℃, 48 h): (a) Without cold rolling, route 2; (b) With cold rolling in 50% reduction, route 5
the aging response of 7A04 aluminum alloy is accelerated by cold deformation after solution treatment, the tensile strength of the alloy does not increase and the corresponding elongation decrease obviously (Fig.2). The effect of cold deformation after solution treatment on the 2024 aluminum alloy and 7A04 aluminum alloy is different. Observing the fracture surfaces of the 2024 aluminum alloy by SEM, it is indicated that the fracture surfaces are fine equiaxial dimple fracture structures (Fig.3(a)), which represents the homogeneous deformation and is consistent with the high elongation of the alloy (Fig.1). There are many dimples on the fracture surfaces of 7A04 aluminum alloy after aging treatment. This is consistent with the high elongation of the alloy (17%). But there are also a few shear-plateaus on the fracture surfaces, which gives evidence to the heterogeneous fracture (Fig.3(b)). Compared with the 2024 alloy, there are more shear-plateaus on the fracture surfaces of the 7A04 alloy treated by cold deformation after solution treatment, and the quantity of the dimples is relatively low (Fig.3(c)). This is consistent with the low elongation of the 7A04 alloy (Fig.2) and reflects the increase of the heterogeneous deformation during fracture process.
TEM observation shows that at the early stage of aging the dislocation cell structures are still retained in both alloys treated by cold deformation after solution treatment (Fig.4). Furthermore, there are no obvious differences in size and the spacing of the S′ precipitates between the high density of dislocation region and the low density of dislocation region with the prolonging of aging time (labeled by the filled and unfilled arrows in Fig.5). It is indicated that the nucleation and the growth of the S′ phase seem to be independent of the dislocation. Therefore, it has no obvious tendency of heterogeneous nucleation and exhibits a homogeneous nucleation and growth. It is suggested that the precipitation of the G.P zones is independent on the dislocation and the dislocation cell structures in 2024 aluminum alloy. As can be seen in Fig.6 that although in the 7A04 aluminum alloy the spherical G.P zones with size about 1-2 nm is still distributed on the non-dislocation matrix, the rod formed η′ phase has already appeared on the dislocation line and within the dislocation cells (labeled by the unfilled arrows in Fig.6). When aged at 120 ℃ for 48 h, a coarse club-shaped η′ phase of 20 nm in size and even a small amount of η equilibrium phase in size close to 200 nm have appeared in samples of 7A04 alloy with cold rolling, while a homogeneous small spheric G.P zone still in samples without cold rolling. Those results mentioned above suggest that the nucleation and growth of η′-phase are dependent on the dislocation, and exhibit a strong tendency of heterogeneous nucleation behavior. As a result, the homogeneous strengthening and the deformation strengthening effect in the 2024 aluminum alloy is due to the homogeneous nucleation and growth of the G.P zones and S′ phase. And the alloys present uniform equiaxial dimple fracture structures in the fracture surface (Fig.3(a)). The heterogeneous nucleation of the G.P zones and η′ precipitates in the 7A04 aluminum alloy is dependent on the dislocations and the dislocation cells, causing the heterogeneous deformation effect and the heterogeneous strengthening effect. And the alloys present non-uniform deformation structures in the fracture surfaces and low strengthening effect (Figs.2, 3(a) and (b)).
From the observation and analysis mentioned above, it is indicated that the S′ phase of the 2024 aluminum alloy does not tend to heterogeneously nucleate and grow on the dislocations and the dislocation cells. Whereas, the η′ phase in the 7A04 aluminum alloy is inclined to heterogeneously nucleate and grow on the dislocations and the dislocation cells. The opinion that the homogeneous nucleation of the S′ phase is independent of the dislocation is different from the previous results which show the dislocation inducing the heterogeneous nucleation and growth of the precipitates[17]. SINGH et al[14] and ZHAO et al[15] have obtained a great improvement of the strength by adopting cold deformation after solution and aging treatment, but the authors still suggest that the dislocation induce the heterogeneous nucleation and growth of the strengthening phase. As for the opinion that the η′ phase in the 7A04 aluminum alloy heterogeneously nucleate and grow on the dislocations and the dislocation cells is at variance with the result of Ref.[16]. The reason for the preferential nucleation of precipitates on the dislocations and the dislocation cells is due to the reduction of the nucleation energy of precipitation. As for the 2024 aluminum alloy, in the early stage of aging the precipitates are mainly cylindrical G.P zones[19]. Because the part of the G.P zones which are coherent with the matrix are in the edges of the two ends of the cylinder with only several atomic layer thickness, so the coherent strain energy is relatively small. But for the 7A04 aluminum alloy, spherical G.P zones are precipitated in aging when solution-treated above 460 ℃ [19], and the coherent strain energy is higher than the 2024 aluminum alloy due to the spherical G.P zones coherent with the matrix in the whole surface of sphere within a range of a few atom layers. Meanwhile, because the G.P zones are not the phase, so there are no interfaces between the G.P zones and the matrix. In this case, the coherent strain energy predominates the nucleation of the G.P zones. GANG and ALFRED[19] have indicated that the reason for spherical G.P zones elongated to short rod form is to reduce the strain energy in the 7000 serial aluminum alloy. Therefore, the tendency of nucleation and growth of the spherical G.P zones on dislocation line or dislocation cell in the 7A04 aluminum alloy is higher than in the 2024 aluminum alloy. So it is suggested that the G.P zones and the η′ phase nucleate and grow heterogeneously in the 7A04 aluminum alloy, while the G.P zones and S′ phase nucleate and grow homogeneously in the 2024 aluminum alloy. Therefore, the alloys present non-uniform deformation and low strengthening effect in the 7A04 aluminum alloy. And it represents uniform deformation and strong strengthening effect in the 2024 aluminum alloy. These are consistent with the results of the present work.
5 Conclusions
From the analysis and experimental results above it can be concluded that the tendency for S′ phase in 2024 aluminum alloy to nucleate heterogeneously on dislocation line and at dislocation cell is small, by which a dispersed uniform S′ phase is precipitated and an obvious strengthening effect obtained when aged under the condition of solid solution treatment followed even by large cold rolling deformation; contrarily the tendency for η′ phase in 7A04 alloy to heterogeneously nucleate on dislocation line and at dislocation cell is great and a nonuniform growth and rapid coarsening of precipitates easily occur, therefore a relative rise in strength in 7A04 aluminum alloy can not be achieved by large cold deformation after solid resolution.
References
[1] DUCKWORTH W E. Thermomechanical treatment of metals [J]. JOM, 1966, 1: 915-922.
[2] MCEVILY A J, CLARK J B, BOND A P. Effect of thermal-mechanical processing on the fatigue and stress-corrosion properties of an Al-Zn-Mg alloy [J]. ASM Trans, 1967, 60: 661-671.
[3] RUSSO E D, CONSERVA M, BURATTI M, GATTO F. A new thermo-mechanical procedure for improving the ductility and toughness of Al-Zn-Mg-Cu alloys in the transverse directions [J]. Mater Sci Eng A, 1974, 14: 23-36.
[4] WALDMAN J, SULINSKI H, MARKUS H. Effect of ingot processing treatments on the grain size and properties of Al alloy 7075 [J]. Metall Trans, 1974, 5(3): 573-584.
[5] SANDERS R E, STARKE E A. The effect of intermediate thermomechanical treatments on the fatigue properties of A 7050 aluminum alloy [J]. Metall Trans, 1978, 9(8): 1087-1100.
[6] WERT J A, PATON N E, HAMITON H, MAHONEY M W. Grain refinement in 7075 aluminum by thermo-mechanical processing [J]. Metall Trans, 1981, 12A(7): 1267-1276.
[7] PATON N E, HAMITON H, WERT J A, MAHONEY M W. Characterization of fine-grained superplastic aluminum alloys [J]. JOM, 1982, 8: 2-27.
[8] EL-BARADIE Z M, EL-SAYED M. Effect of thermomechanical treatments on the properties of 7075 Al alloy [J]. J Mater Processing Tech, 1996, 62: 76-80.
[9] OSTERMANN F. Improved fatigue resistance of Al-Zn-Mg-Cu alloys through thermo-mechanical processing [J]. Met Trans, 1971, 2(10): 2897-2902.
[10] RUSSO E D I, CONSERVA M, BURATTI M, GATTO F. A new procedure for improving the ductility and toughness of Al-Zn-Mg-Cu alloys in the transverse directions [J]. Mater Sci Eng, 1974, 14: 23-36.
[11] RACK H J. The influence of prior strain upon precipitation in a high-purity 6061 aluminum alloy [J]. Mater Sci Eng A, 1977, A29: 179-188.
[12] CHIA E H, STRARKE E A. Application of subgrain control to aluminum wire products [J]. Metall Trans A, 1977, A8(6): 825-832.
[13] TIAN Rong-Zhang, WANG Zhu-Tang. Handbook of the aluminum alloys and its fabrication [M]. 2nd ed. Changsha: Central South University Press, 2000, 186-221.
[14] SINGH S, GOEL D B. Influence of thermomechanical ageing on tensile properties of 2014 aluminum alloy [J]. J Mater Sci, 1990, 25: 3894-3900.
[15] ZHAO Gang, LI Hong-xiao, LIU Chun-ming, GUO Ya-ping. Thermomechanical ageing of 2014 aluminum alloy [J]. J Northeastern Univ (Natural Science Edition), 2001, 22(6): 664-667.
[16] STOLYAROV V V, LATYSH V V, SHUNDALOV V A, SALIMONENKO D A, ISLAMGALIEV R K, VALIEV R Z. Influence of severe plastic deformation on aging effect of Al-Zn-Mg-Cu-Zr alloy [J]. Mater Sci Eng A, 1997, A234-236: 339-342.
[17] RADMILOVIC V, THOMAS G, SHIFLET G J, STARKE E A. On the nucleation and growth of Al2CuMg(S′) in Al-Li-Cu-Mg and Al-Cu-Mg alloys [J]. Scripta Metall, 1989, 23: 1141.
[18] KOVARIK L, GOUMA P I, KISIELOWSKI C, COURT S A, MILLS M J. A HRTEM study of metastable phase formation in Al-Mg-Cu alloys during artificial aging [J]. Acta Materialia, 2004, 52(3): 2509-2520.
[19] GANG Sha, ALFRED C. Early precipitation in Al-Zn-Mg-Cu alloy (7050) [J]. Acta Mater, 2004, 52: 4913-4516.
(Edited by LONG Huai-zhong)
Foundation item: Project(50571069) supported by NSFC and Project(05A061) education department of Hunan province, China
Corresponding author: LIU Zhi-yi; Tel: +86-731-8836927; E-mail: liuzhiyi@mail.csu.edu.cn