
Desiliconization kinetics of nickeliferous laterite ores in molten sodium hydroxide system
MU Wen-ning(牟文宁), ZHAI Yu-chun(翟玉春)
Institute of Metallurgical Physical Chemistry, Northeastern University, Shenyang 110004, China
Received 16 October 2008; accepted 19 May 2009
Abstract: A novel process was proposed for treating nickeliferous laterite ores with molten sodium hydroxide. The effect on silicon extraction caused by the factors, such as stirring speed, reaction temperature, particle size and NaOH-to-ore mass ratio, was investigated. The results show that increasing stirring speed, reaction temperature and NaOH-to-ore mass ratio while decreasing particle size increases silicon extraction rate. The desiliconization kinetics of nickeliferous laterite ores in molten sodium hydroxide system was described successfully by chemical reaction control model. The activation energy of the desiliconization process was found to be 44.01 kJ/mol, and the reaction rate based on a chemical reaction-controlled process can be expressed as: 1-(1-α)1/3= 27.67exp[-44 010/(RT)]t.
Key words: nickeliferous laterite ores; sodium hydroxide; silicon dioxide; kinetics; desiliconization
1 Introduction
In recent years, there is an increasing focus on the utilization of nickel laterite ores, along with the growing of the demand for stainless steel and the declining of sulphide ores[1-3]. It was estimated that 70% of the world land-based nickel resources are contained in nickel laterites[4], although they only account for about 40% of the world’s nickel production[5-7]. Nickeliferous laterite ores deposits are formed by the chemical weathering of nickeliferous peridotite rock under humid climates[8-9]. Through weathering processes, the nickel is finally concentrated in different secondary minerals. The complex mineralogy and low nickel content of the nickeliferous laterite ores render beneficition almost impossible and the direct extraction of nickel is quite difficult[10-11].
At present, the typical metallurgical methods for treating nickel laterite ores include pyro- and hydro- metallurgical processes. These methods, however, are costly, especially the pyro-metallurgical treatment[12], which generates huge volumes of waste residues with silicon so that brings the enormous burden to environment. Therefore, in the long term it will be necessary to develop new economical processing technologies, and improve value-added utilization of nickeliferous laterite ores.
One new technology, which extracts silicon from nickel laterite ores taking fusing NaOH as the reaction medium, is established. The process not only takes full advantage of silicon, but also makes nickel enriched in the solid residuals and creates the conditions for the high value-added utilization of nickeliferous laterite ores. The objective of this work is to assess the effects of different variables, such as stirring speed, reaction temperature, NaOH-to-ore mass ratio and particle size, on the reaction rate, to optimize the conditions and determine the kinetics of the reaction.
2 Experimental
2.1 Materials
The nickeliferous laterite ores were crushed and screened to different size fractions ranging from 44 ?m to 150 ?m. Table 1 shows the chemical analysis of the 44-61 ?m size fraction which was used in all the experiments except the study on the effect of particle size on silicon extraction.
Table 1 Chemical composition of nickeliferous laterite ores (mass fraction, %)

The X-ray diffraction pattern of nickeliferous laterite ores shown in Fig.1 indicates that serpentine is the major mineral phase, and dissociative silicon dioxide and hematite are minor phases. Furthermore, silicon is contained in magnesium silicates and dissociative silicon dioxide.
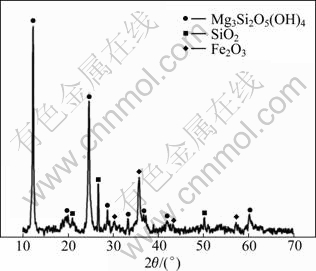
Fig.1 XRD pattern of nickeliferous laterite ores
2.2 Method
The chemical experiments were carried out batch- wise in an open stainless steel reactor. The resistance furnace of wire type was used, and the temperature of which was controlled by a programmable temperature controller with a precision of ±2 ℃. Agitation was provided by a mechanical stirrer that enables adequate dispersion of the mineral particles. The calculated mass of NaOH was added to the reactor and heated to the selected temperature. When the temperature was reached, the nickeliferous laterite ores were added and the reaction commenced. After reacted for selected time intervals, the slurry was cooled rapidly so that the reaction was stopped. After leaching with water of 80 ℃ for 20 min, the slurry was filtered. The content of silicon in sodium silicate solution was determined by using atomic absorption spectrometry, and the silicon dioxide extraction rate was calculated as
×100% (1)
where α(SiO2) is the silicon dioxide extraction rate; m′(SiO2) is the mass of silicon dioxide in sodium silicate solution; and m(SiO2) is the mass of silicon dioxide in nickeliferous laterite ores.
3 Results and discussion
3.1 Chemical reactions
The main chemical reactions that occur between nickeliferous laterite ores and NaOH are as follows:
Mg3Si2O5(OH)4+4NaOH=3Mg(OH)2+2Na2SiO3+H2O
(2)
SiO2+2NaOH=Na2SiO3+H2O (3)
Due to the existence of a small amount of alumina, the following reaction will take place in the system:
Al2O3+2NaOH+3H2O=2NaAl(OH)4 (4)
When the concentration of alumina reaches a certain value in the solution, the reaction between sodium aluminate and sodium silicate will occur[13-14], which can be written as
nNa2SiO3+2NaAl(OH)4=
Na2O?Al2O3?nSiO2+2nNaOH+(4-n)H2O (5)
3.2 Effect of stirring speed
The effect of stirring speed on the desiliconization rate was examined firstly. The experiments were carried out at stirring speeds of 100, 200, 300, 400, 500 and 600 r/min, respectively. In these experiments, NaOH-to-ore mass ratio, particle size and reaction temperature were fixed at 4?1, 44-61 ?m, and 550 ℃, respectively. The results are presented in Fig.2.
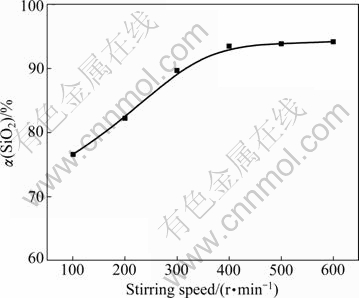
Fig.2 Effect of stirring speed on silicon dioxide extraction rate
It is evident from Fig. 2 that the stirring speed has a little effect on the extraction of silicon dioxide. As expected, silicon dioxide extraction rate increases steadily with stirring speed increasing from 100 to 400 r/min and afterwards it remained constant. At 400 r/min and above, there is an adequate suspension of the solid particles. Thus 400 r/min was chosen for the subsequent experiments to eliminate the effect of stirring speed as a variable in the rate study.
3.3 Effect of reaction temperature
The influence of reaction temperature on the silicon dioxide extraction rate under the following conditions: stirring speed 400 r/min, NaOH-to-ore mass ratio 4?1 and particle size 44-61 ?m, is shown in Fig.3.
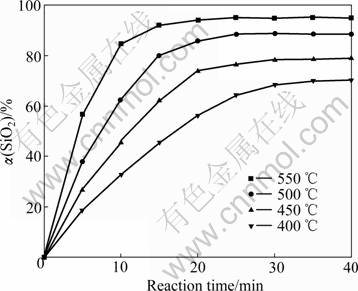
Fig.3 Effect of reaction temperature on silicon dioxide extraction rate
The temperature has a noticeable influence on the extraction of silicon dioxide. The extraction of silicon dioxide increases with the increase of the reaction temperature. At 400 ℃, 56.27% silicon dioxide is extracted after 20 min reaction; at 550 ℃, 94.35% silicon dioxide is extracted. A significant decreasing of the reaction rate in the final reaction stage was observed. The reason is that silicon dioxide in nickeliferous laterite ores tended to react completely.
3.4 Effect of NaOH-to-ore mass ratio
The influence of NaOH-to-ore mass ratio on the extraction of silicon dioxide was studied under the conditions of 550 ℃, stirring speed 400 r/min and particle size 44-61 ?m.
As shown in Fig.4, the silicon dioxide extraction rate is improved with the increase of NaOH-to-ore mass ratio. Sodium hydroxide acts as a fluidizing and fluxing agent in the reaction. Excess sodium hydroxide is necessary to maintain the liquidity of the reactants and ensure sufficient reactions. While the extra sodium hydroxide could significantly affect the economy of the whole process because of the recycling procedure in the overall process[15]. In order to investigate the other parameters, NaOH-to-ore mass ratio of 4?1 was chosen.
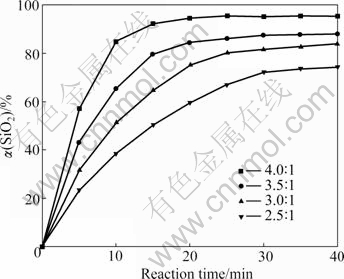
Fig.4 Effect of NaOH-to-ore mass ratio on silicon dioxide extraction rate
3.5 Effect of particle size
The influence of particle size on the silicon dioxide extraction was examined with four different size fractions (150-178, 104-124, 74-89 and 44-61 μm) at 550 ℃ under a stirring speed of 400 r/min and NaOH- to-ore mass ratio of 4:1. The results are shown in Fig.5. It can be seen that the silicon dioxide extraction rate increases with the decreasing of particle size, but the particle size plays a minor role in the reaction process. The extraction of silicon dioxide in the experiment with the particle size of 44-61 μm is only 6% higher than that with particle size of 150-178 μm. The small difference is probably due to the negligible increase of particle surface area with decreasing particle size for an effect of porosity and natural cracks. In order to get higher silicon dioxide extraction rate, the ore granularity of 44-61 μm in most experiments was controlled.
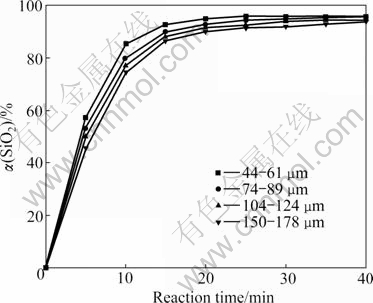
Fig.5 Effect of particle size on silicon dioxide extraction rate
3.6 Kinetics analysis
Desiliconization of nickeliferous laterite ores in molten sodium hydroxide system is a typical liquid-solid reaction, which can be analyzed with the shrinking core model[16]. According to this model, the rate of reaction between solid particle and reaction reagent may be controlled by one of the following steps: diffusion through the fluid film, diffusion through the product layer, and the chemical reaction at the surface. Assuming that the particles of nickeliferous laterite ores have spherical geometry and the surface chemical reaction is the rate-controlling step, the following expression of the shrinking core model can be used to describe the desiliconization kinetics of the process.
1-(1-α)1/3=krt (6)
Similarly, when the diffusion through the product layer is the rate-controlling step, the following expression of the shrinking core model can be used to describe the desiliconization kinetics.
1+2(1-α)-3(1-α)2/3=kdt (7)
From the experimental data, it was determined that the surface chemical reaction controlled model was suitable for this reaction process. The rate constants and their correlation coefficients are given in Table 2 for the diffusion through the product layer and the surface chemical reaction controlled model. Furthermore, the linear relationship between 1-(1-α)1/3 and the reaction time can be seen in Figs.6 and 7 for the particle size and the reaction temperature, respectively. In accordance with these results, the equation representing the kinetics of this process was determined to obey the surface chemical reaction model.
Table 2 Apparent rate constant and correlation coefficient values
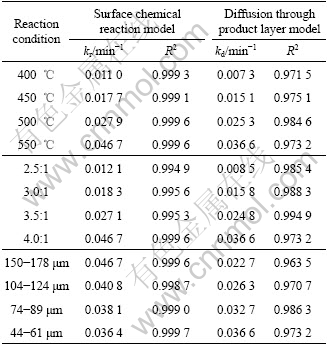
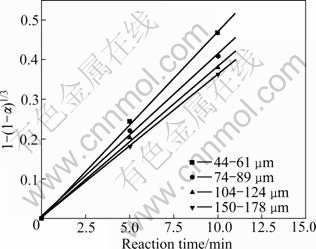
Fig.6 Plot of 1-(1-α)1/3 vs time under different particle sizes
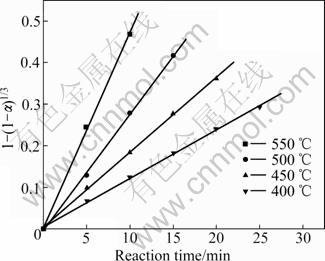
Fig.7 Plot of 1-(1-α)1/3 vs time at different temperatures
In addition, if the surface chemical reaction controls the reaction rate, the relationship between the apparent rate constant and the inverse of the particle radius must be linear. This relationship is given in Fig.8. Fig.8 confirms that the surface chemical reaction model can be the rate controlling step for this process.
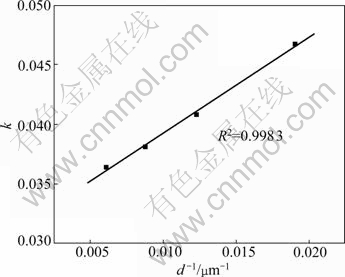
Fig.8 Plot of rate constant vs d-1
The apparent rate constant was determined from the straight lines in Fig.7 and was plotted according to the Arrhenius equation as shown in Fig.9. The apparent activation energy of the desiliconization process was calculated to be 44.01 kJ/mol. Diffusion controlled processes are slightly dependent on temperature, while chemically controlled processes are strongly dependent on temperature. Therefore, the value of activation energy of desiliconization reaction may be used to predict the rate-controlling step. The activation energy of diffusion controlled process is typically between 4 and 12 kJ/mol, while, for a chemically controlled process, the value is usually greater than 40 kJ/mol[17-18]. The value of the apparent activation energy in the desiliconization process of nickeliferous laterite ores confirms that this desiliconization process is controlled by the surface chemical reaction, and the kinetics model for the desiliconization process of nickeliferous laterite ores can be expressed as
1-(1-α)1/3=27.67exp[-44 010/(RT)]t (8)
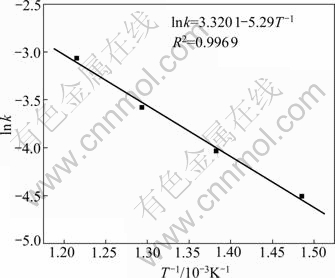
Fig.9 Arrhenius plot for desiliconization of nickeliferous laterite ores
3.7 Characterization of solid residuals
The solid residuals, reacted at 550 ℃ for 20 min under the conditions of stirring speed 400 r/min, particle size 44-61 ?m and NaOH-to-ore mass ratio 4?1, were characterized by SEM observation, X-ray diffractometry and chemical analysis.
The SEM images of nickeliferous laterite ores and solid residuals are presented in Fig.10. The contact surface of ore (Fig.10(a)) was eroded by molten sodium hydroxide and finally a loose surface formed after being dissolved at 550 ℃ for 20 min (Fig.10(b)). The morphology of the solid residuals implies that the desiliconization process of nickeliferous laterite ores in molten sodium hydroxide system is controlled by the surface chemical reaction.
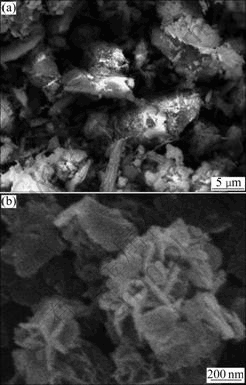
Fig.10 SEM images of nickeliferous laterite ores (a) and solid residuals (b)
The chemical composition of solid residuals is shown in Table 3. Compared with Table 1, it can be seen that the content of silicon dioxide is lowered, and the contents of magnesium, iron and nickel are improved, of which the content of nickel oxide is 1.62%. The purpose of cumulating other elements by extracting silicon from nickeliferous laterite ores is realized.
Table 3 Chemical composition of solid residuals (mass fraction, %)

The XRD patterns of solid residuals after different reaction time (Fig.11) indicate that the major mineral phase is magnesium hydroxide and hematite is the minor phase. The main reactions taking place between nickeliferous laterite ores and sodium hydroxide is reactions (2) and (3) during the desiliconization of nickeliferous laterite ores in molten sodium hydroxide system. The formation of sodium aluminosilicate at 30 min illustrates the occurrence of reaction (5).
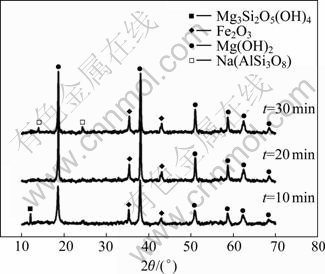
Fig.11 XRD patterns of solid residuals after different reaction time
4 Conclusions
1) The effect of reaction parameters on the desiliconization rate of silicon dioxide was examined and evaluated. It was found that the extraction rate of silicon dioxide increases with increasing the stirring speed, reaction temperature and NaOH-to-ore mass ratio, and with decreasing particle size.
2) The extraction rate of silicon dioxide is up to 94.35% under the conditions of reaction temperature 550 ℃, reaction time 20 min, mass ratio of NaOH to ore 4?1, particle size 44-61 ?m and stirring speed 400 r/min. The elements of iron, magnesium and nickel are enriched in the solid residuals, and the content of nickel oxide is 1.62%.
3) Desiliconization kinetics of nickeliferous laterite ores in molten sodium hydroxide system was studied, which was found to fit the shrinking core model for a chemical reaction controlled process. The activation energy was determined to be 44.01 kJ/mol, and the kinetics model can be expressed as: 1-(1-α)1/3=27.67× exp[-44 010/(RT)]t.
References
[1] PICKLES C A. Microwave heating behaviour of nickeliferous limonitic laterite ores [J]. Minerals Engineering, 2004, 17: 775-784.
[2] LEE H Y, KIM S G, OH J K. Electrochemical leaching of nickel from low-grade laterites [J]. Hydrometallurgy, 2005, 77: 263-268.
[3] McDONALD R G, WHITTINGTON B I. Atmospheric acid leaching of nickel laterites review (Part I): Sulphuric acid technologies [J]. Hydrometallurgy, 2008, 91: 35-55.
[4] MOSKALYK R R, ALFANTAZI A M. Nickel laterite processing and electrowinning practice [J]. Minerals Engineering, 2002, 15: 593-605.
[5] ELIAS M. Nickel laterite deposits—Geological overview, resources and exploration [C]// COOKE D, PONTGRATZ J. Giant Ore Deposits. Characteristics, Genesis and Exploration. Hobart: University of Tasmania, 2002: 205-220.
[6] GLEESON S A, HERRINGTON R J, DURANGO J, VEL?ZQUEZ C A, KOLL G. The mineralogy and geochemistry of de Cerro Matoso S.A. Ni laterite deposit, Montelíbano, Colombia [J]. Economic Geology, 2004, 99: 1197-1213.
[7] DALVI A D, BACON W G, OSBORNE R C. Past and future of nickel laterite projects [C]// Trade Show and Investors Exchange, International Nickel Laterite Symposium TMS 2004–133rd Annual Meeting and Exhibition. Charlotte, N. Carolina, 2004: 23.
[8] PICKLES C A. Drying kinetics of nickeliferous limonitic laterite ores [J]. Minerals Engineering, 2003, 16: 1327-1338.
[9] SOLER J M, CAMA J, GAL? S, MEL?NDEZ W, RAM?REZ A, EATANGA J. Composition and dissolution kinetics of garierite from the Loma de Hierro Ni-laterite deposit, Venezuela [J]. Chemical Geology, 2008, 249: 191-202.
[10] BRAND N W, BUTT C R M, ELIAS M. Nickel laterites: Classification and features [J]. AGSO Journal of Australian Geology and Geophysics, 1998, 17: 81-88.
[11] SWAMY Y V, KAR B B, MOHANTY J K. Physico-chemical characterization and sulphatization roasting of low-grade nickeliferous laterites [J]. Hydrometallurgy, 2003, 69: 89-98.
[12] DEEPATANA A, TANG J A, VALIX M. Comparative study of chelating ion exchange resins for metal recovery from bioleaching of nickel laterite ores [J]. Minerals Engineering, 2006, 19: 1280-1289.
[13] YANG Bo, WANG Jing-gang, ZHANG Yi-fei, ZHANG Yi. Pre-desiliconization of a bauxite ore through leaching by high concentration NaOH solution under atmospheric pressure [J]. The Chinese Journal of Process Engineering, 2007, 7(5): 922-927. (in Chinese)
[14] LI Guang-hui, JIANG Tao, FAN Xiao-hui, HUANG Zhu-cheng, QIU Guan-zhou. Technology of desilication from diasporic bauxite by roasting-alkali leaching process (Ⅲ) [J]. The Chinese Journal of Nonferrous Metals, 2000, 10(6): 899-904. (in Chinese)
[15] CHANDRA D, WANG G X, FUERSTENAU M C, SIEMENS R E. Alkali roasting of low-grade chromite [J]. Transactions of the Institution of Mining and Metallurgy (Section C: Mineral Process Extr Metall), 1996: C105-C112.
[16] LEVENSPIEL O. Chemical reaction engineering [M]. New York: John Wiley & Sons, 1999: 664.
[17] ABDEL-AAL E A. Kinetics of sulfuric acid leaching of low-grade zinc silicate ore [J]. Hydrometallurgy, 2000, 55: 247-254.
[18] ASHRAF M, ZAFAR Z I, ANSARI T M. Selective leaching kinetics and upgrading of low-grade calcareous phosphate rock in succinic acid [J]. Hydrometallurgy, 2005, 80: 286-292.
Foundation item: Project(2007CB613603) supported by the National Basic Research Program of China
Corresponding author: ZHAI Yu-chun; Tel: +86-24-83687731; E-mail: danae2007@yahoo.cn
DOI: 10.1016/S1003-6326(09)60142-4
(Edited by YUAN Sai-qian)