J. Cent. South Univ. Technol. (2008) 15: 209-213
DOI: 10.1007/s11771-008-0040-9

Preparation of precursor for stainless steel foam
ZHOU Xiang-yang(周向阳)1, LI Shan-ni(李善妮)2, LI Jie(李 劼)1, LIU Ye-xiang(刘业翔)1
(1. School of Metallurgical Science and Engineering, Central South University, Changsha 410083, China;
2. School of Biological Science and Technology, Central South University, Changsha 410013, China)
Abstract: The effects of polyurethane sponge pretreatment and slurry compositions on the slurry loading in precursor were discussed, and the performances of stainless steel foams prepared from precursors with different slurry loadings and different particle sizes of the stainless steel powder were also investigated. The experimental results show that the pretreatment of sponge with alkaline solution is effective to reduce the jam of cells in precursor and ensure the slurry to uniformly distribute in sponge, and it is also an effective method for increasing the slurry loading in precursor; the mass fraction of additive A and solid content in slurry greatly affect the slurry loading in precursor, when they are kept in 9%-13% and 52%-75%, respectively, the stainless steel foam may hold excellent 3D open-cell network structure and uniform muscles; the particle size of the stainless steel powder and the slurry loading in precursor have great effects on the bending strength, apparent density and open porosity of stainless steel foam; when the stainless steel powder with particle size of 44 μm and slurry loading of 0.5 g/cm3 in precursor are used, a stainless steel foam can be obtained, which has open porosity of 81.2%, bending strength of about 51.76 MPa and apparent density of about 1.0 g/cm3.
Key words: stainless steel foam; precursor; preparation; open porosity; bending strength; apparent density
1 Introduction
Porous or cellular metallic materials attract more and more researching interests and their application fields are being developed due to that they can offer many excellent performances such as light quality, high specific strength and stiffness, excellent crash energy and sound absorption abilities[1-4]. Recently, higher melting point porous metallic materials have found an increasing range of applications in many fields. For example, high porosity open-cell Fe-based metal foam can be used as heat exchangers[5], stainless steel foam with 3D open-cell network structure and excellent anti-corrosion property can be used as catalyst carriers in purifying automotive exhaust gases[6], high melting point metal foams can be used as flow field and gas diffusion layer in direct methanol fuel cells[7].
At present, porous metallic materials with higher melting points are usually prepared by powder metallurgy (PM) approaches; sometimes, metal fibre or foaming agents such as MgCO3, SrCO3 or others are used in the preparation process[1, 4, 8-11]. However, cells existing in these porous metallic materials prepared by the above methods are smaller, sometimes partial cells are closed; in addition, it is difficult to prepare higher melting points porous metallic materials with 3D open-cell network structure by the above methods. So, developing a novel preparation technology of higher melting points porous metallic materials with 3D open-cell network structure is significant.
Recently, interests have been focused on the research of stainless steel foams(SSFs) with 3D open-cell network structure in our group, and a novel preparation technology of SSFs has been developed[12]. The preparation technology of precursor for SSFs was mainly investigated in this work.
2 Experimental
2.1 Preparation of precursors and SSFs
Stainless steel powders with different particle sizes were the main materials used in this study. Their chemical compositions are given in Table 1. Other materials were additive A, polyurethane sponge (0.8 pores/mm) and high-purity argon (99.999%).
Main apparatuses used in this study were a controlled temperature oven with a maximal temperature of 300 ℃ and a controlled atmosphere electric furnace with a maximal temperature of 1 600 ℃.
The preparation processes of precursor and SSFs are simply described as follows: 1) confecting slurry contain- ing a given amount of solid mass fraction by mixing the stainless steel powder and additive A; 2) preparing pre-
Table 1 Chemical compositions of 316L stainless steel powder (mass fraction, %)

cursor with a certain loading of drying slurry by pouring the slurry into polyurethane sponge and drying at 120 ℃ for 24 h; 3) preparing SSFs samples by sintering the precursors under flowing argon at (1 260±2) ℃ for 30 min. The detailed preparation processes of precursors and SSFs were described in Ref.[12].
2.2 Testing methods
The load refers to the dry mass of slurry in unit volume of polyurethane sponge (g/cm3).
In addition, digital camera (SONY, DSC-P10) and scanning electron microscope (JEOL, JSM5600) were employed to observe the apparent appearance and section morphologies of samples, respectively. Metallurgical microscope (XJP-6A) was used to investigate the grain sizes and microstructures of samples. Electric omnipotence tester (CSS-44100) was employed to test the bending strength of samples. The apparent density and open porosity of samples were tested according to the usual methods described in Ref.[13].
3 Results and discussion
3.1 Effects of polyurethane sponge pretreatment on apparent appearance and load in precursors
In this work, pretreatment of sponge refers to immersing sponge in alkaline solution. In order to study the effects of polyurethane sponge pretreatment on precursors, fastening other technologic conditions was necessary. In the experiments, particle size of stainless steel powder was 44 μm, the mass fraction of additive A and solid content (i.e. mass fraction of stainless steel powder) in slurry were 10% and 60%, respectively.
Fig.1 shows the apparent appearance photographs of precursors before and after polyurethane sponge being pretreated with alkaline solution. Obviously, the slurry is more uniformly distributed in pretreated sponge, and the cell-jammed phenomenon is severer in un-pretreated sponge. This may be due to the improvement of wettability between slurry and pretreated sponge.
On the other hand, the pretreatment also influences the load of slurry in precursors. Fig.2 shows the effects of pretreatment on load. Apparently, pretreatment of sponge is an effective method to increase the load. So, all polyurethane sponge used in this work had been pretreated by alkaline solution with the exception of special statement.
Fig.3 shows the SEM image of SSFs from pre-
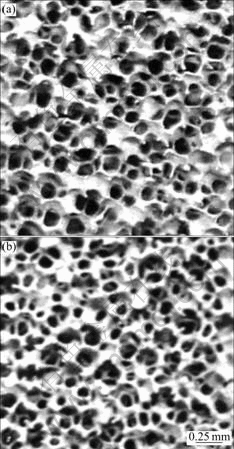
Fig.1 Apparent photographs of precursors with and without pretreatment: (a) Un-pretreated; (b) Pretreated
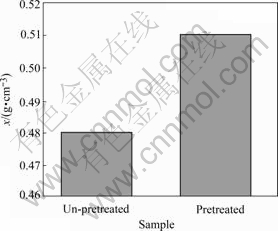
Fig.2 Effects of pretreatment on load of slurry in precursors
treated sponge. Obviously, the sample exhibits uniform muscles and excellent 3D open-cell network structure.
3.2 Effects of concentration of additive A and solid mass fraction in slurry on load in precursors
In the experiments, the particle size of the stainless
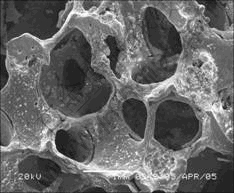
Fig.3 SEM image of SSFs sample
steel powder was 44 μm.
Fig.4 shows the effects of mass fraction of additive A on load in precursors. Apparently, the load gradually increases with rising the mass fraction of additive A in slurry. From experimental phenomena, it is found that the slurry is hardly poured into the sponge when the mass fraction of additive A is less than 9%, and a higher mass fraction of additive A (>13%) is unsuitable for much more slurry staying in sponge. This may be due to the fact that the thixotropy of slurry is poorer in the case of lower additive mass fraction, and the viscosity of slurry is too larger under higher additive mass fraction[14].
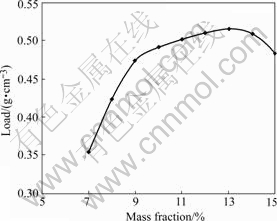
Fig.4 Effects of mass fraction of additive A on load of slurry in precursor (Mass fraction of solid in slurry is 66.7%)
Fig.5 shows the load changes at different solid mass fractions in slurry. Evidently, the load in precursor increases with rising solid mass fraction. Experimental phenomena also show that the slurry hardly settled in sponge when the solid mass fraction is less than 30% due to the fact that the slurry is too dilute, the cell-jammed phenomenon and bigger hollows co-exist on the surface of samples when the solid mass fraction is less than 52%, and the cell-jammed phenomenon becomes severer when the solid mass fraction is more than 75% due to the worsened fluidity of the slurry.
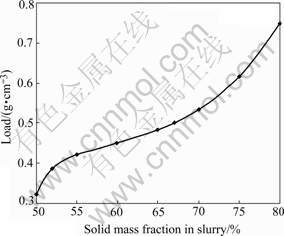
Fig.5 Effects of solid mass fraction in slurry on load (The mass fraction of additive A in slurry is 11%)
3.3 Effects of load in precursors on apparent density, open porosity and bending strength of SSFs samples
The load range of samples used in the experiment was from 0.42 to 0.69 g/cm3, and the particle size of stainless steel powder was 44 μm. The correlations between load in precursors and apparent density of SSFs samples are given in Fig.6. Fig.7 presents the effects of load on open porosity and bending strength of SSFs.
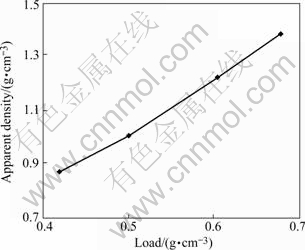
Fig.6 Effects of load on apparent density of SSFs samples
It is clearly observed that the apparent density of SSFs gradually increases with rising the load, while the open porosity falls with rising the load, and raising load is beneficial to the enhancement of bending strength. However, the cell-jammed phenomena appear while the load is more than 0.675 g/cm3. A SSFs sample with a bigger open porosity (about 81.2% ) and higher bending strength (51.76 MPa) can be obtained at the load of 0.5 g/cm3.
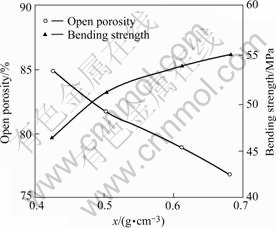
Fig.7 Effects of load on open porosity and bending strength of SSFs samples
3.4 Effects of particle size of stainless steel powder on open porosity and bending strength of SSFs samples
Four stainless steel powders with different particle sizes were used in the experiments; the particle sizes were 37, 44, 50 and 61 μm, respectively. Here, a kind of slurry containing additive A with mass fraction of 11% and a solid mass fraction of 67% was used, and the load of precursors was about 0.5 g/cm3.
The effects of particle size are shown in Fig.8 and Fig.9. The results indicate that the open porosity of SSFs samples gradually decreases with the decrease of particle size; the bending strength of SSFs from fine particle size is remarkably higher than that from coarse powder, only being 16.33 MPa at the particle size of 61 μm, but rising to 51.76 MPa at 44 μm. The reasons why the bending strength of SSFs from coarse powder is inferior to that from fine particle size may be as follows: 1) the intrinsic surface energy driving force of fine particle size powder with larger specific surface area is higher than that of coarse powder, so that the former can be more easily sintered compactly than the latter[15]; 2) the fluidity of slurry from coarse powder is poorer than that from fine powder, which will bring on the un-uniform distribution of slurry in precursor, as a result that the muscles in SSFs are inhomogeneous, which will cause lower bending strength; 3) there are many defects such as lower cells spheroidizing degree, bigger cavities at the grain boundary and bulky crystal grains exist in SSFs sample from coarse powder, which will also lower the bending strength. The defects existing in SSFs samples from coarse powder can be clearly observed in Fig.10.
Fig.10 presents the differences of metallurgical photographs of SSFs samples with size of 61 μm and 44 μm. The cavities on the grain boundary of sample from coarse powder (61 μm) are evidently bigger than that from fine powder (44 μm), the cavities are indicated by
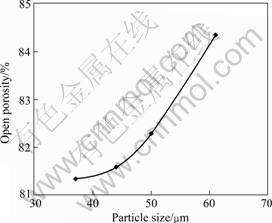
Fig.8 Effects of particle size on open porosity of SSFs samples
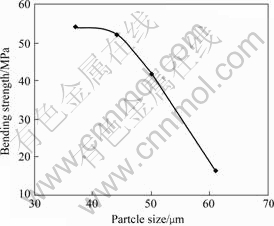
Fig.9 Effects of particle size on bending strength of SSFs samples
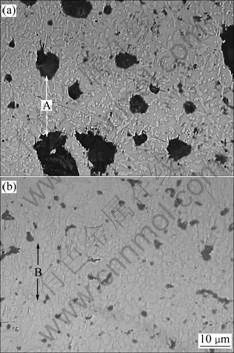
Fig.10 Metallurgical photographs of SSFs samples with different particle sizes: (a) 61 μm; (b) 44 μm
arrows A and B. In addition, the cells spheroidizing degree of sample from coarse powder is lower; however, for SSFs sample from fine powder, and the crystal grain morphology is near-spherical, and the grain boundary is smooth. The measuring results also show that the crystal grain size of sample from fine powder (44 μm) is 1/2 to 1/4 of that from coarse powder (61 μm).
4 Conclusions
1) The pretreatment of sponge with alkaline solution is effective to reduce the jam of cells in precursor and ensure the slurry to uniformly distribute in sponge, and pretreatment of sponge is helpful to increase the slurry loading in precursor.
2) The mass fraction of additive A and solid content in slurry greatly affect the slurry loading in precursor. When the mass fraction of additive A and the solid content in slurry are kept in 9%-13% and 52%-75% respectively, the stainless steel foam may hold excellent 3D open-cell network structure and uniform muscles.
3) With rising the load, the apparent density and bending strength of SSFs gradually increase, while the open porosity falls. With the decrease of particle size, the open porosity of SSFs gradually decreases and the bending strength rises.
4) A stainless steel foam sample with open porosity of 81.2% and bending strength of about 51.76 MPa and apparent density of about 1.0 g/cm3 can be obtained at stainless steel powder particle size of 44 μm and the slurry loading of 0.5 g/cm3 in precursor.
References
[1] ZHAO C Y, LU T J, HODSONA H P, JACKSON J D. The temperature dependence of effective thermal conductivity of open-celled steel alloy foams[J]. Materials Science and Engineering A, 2004, 367(1/2): 123-131.
[2] JOHN B. Manufacture, characterization and application of cellular metals and metal foams[J]. Progress in Materials Science, 2001, 46(6): 559-632.
[3] ZHAO C Y, LU T J, HODSONA H P. Thermal radiation in ultralight metal foams with open cells[J]. International Journal of Heat and Mass Transfer, 2004, 47(14/16): 2927-2939.
[4] PARK C, NUTT S R. PM synthesis and properties of steel foams[J]. Materials Science and Engineering A, 2000, 288(1): 111-118.
[5] LU W, ZHAO C Y, TASSOU S A. Thermal analysis on metal- foam filled heat exchangers (Part I): Metal-foam filled pipes[J]. International Journal of Heat and Mass Transfer, 2006, 49(15/16): 2751-2761.
[6] ZHOU X Y, LI J, LONG B, HUO D W. The oxidation resistance performance of stainless steel foam with 3-D open-celled network structure at high temperature[J]. Materials Science and Engineering A, 2006, 435/436(5): 40-45.
[7] ARISETTY S, PRASAD A K, ADVANI S G. Metal foams as flow field and gas diffusion layer in direct methanol fuel cells[J]. Journal of Power Sources, 2007, 165(1): 49-57.
[8] LIN Xiao-qing, HE Yue-fei, JIAO Yao, ZHANG Feng-shou. Experimental study on effective parameters for filtration performances of stainless steel porous materials[J]. Materials Science and Engineering of Powder Metallurgy, 2005, 10(2): 128-132. (in Chinese)
[9] PARK C, NUTT S R. Effects of process parameters on steel foam synthesis[J]. Materials Science and Engineering A, 2001, 297(1/2): 62-68.
[10] FANG Hui-hui, HUANG Zhao-qiang, XUE Song. SEM analysis of hole structure of metal fibre sintered filtration felt[J]. Physics and Chemistry Test: Physics Fascicule, 2000, 36(6): 258-259. (in Chinese)
[11] WANG Tong-qing. Filtration performance and science application of filtration material of metal fibre sintered felt[J]. Filtrating and Separating, 2003, 13(1): 26-28. (in Chinese)
[12] ZHOU Xiang-yang, LI Jie, DING Feng-qi, LONG Bo. The preparation means of the porous metal foam with 3-D open cells or portion cells connected with each other: CN, 200510032174.7[P]. 2005-09-22. (in Chinese)
[13] LIAO Ji-qiao. Powder metallurgy experiment technology[M]. Changsha: Central South University Press, 2003. (in Chinese)
[14] WANG Xiao-gang, WEI Yun-jing, LI Xiao-chi. Major factors influencing the performance of silicon carbide foam ceramic slurry[J]. Journal of Xi’an University of Science and Technology, 2004, 24(2): 190-192. (in Chinese)
[15] GUO Shi-ju. Powder metallurgy sintering theory[M]. Beijing: Metallurgical Industry Press, 1998. (in Chinese)
(Edited by YANG Hua)
Foundation item: Project(06SK2011) supported by the Science and Technology Program of Hunan Province, China
Received date: 2007-09-28; Accepted date: 2007-11-21
Corresponding author: ZHOU Xiang-yang, PhD; Tel: +861-731-8836329; E-mail: xyzhou@mail.csu.edu.cn