
Oxidation and corrosion behavior of titanium aluminum nitride coatings by arc ion plating
LI Ming-sheng(李明升)1, 2, FENG Chang-jie(冯长杰)2, ZHANG Zhi-feng(张志峰)2, WANG Fu-hui(王福会)2
1. Jiangxi Key Laboratory of Surface Engineering,
Jiangxi Science and Technology Normal University, Nanchang 330013, China;
2. State Key Laboratory for Corrosion and Protection,
Institute of Metal Research, Chinese Academy of Sciences, Shenyang 110016, China
Received 20 April 2006; accepted 30 June 2006
Abstract: Composite metastable TiN and Ti1-xAlxN coatings with different Al content were deposited on 1Cr11Ni2W2MoV stainless steel for aero-engine compressor blades by arc ion plating. The results show that all coatings have a B1NaCl structure and the preferred orientation changes from (111) to (220) with increasing Al content; the lattice parameter of Ti1-xAlxN decreases with the increase of Al content. The oxidation-resistance of (Ti,Al)N coatings is significantly improved owing to the formation of Al-riched oxide on the surface of the coatings. The nitride coatings can significantly improve the corrosion-resistance of 1Cr11Ni2W2MoV stainless steel under the synergistic of water vapor and NaCl, and the corrosion-resistance becomes better when the Al content increases, because not only the quick formation of thin alumina layer prevents the further corrosion but also the formation of alumina seals the pinholes or defects in the coatings, which prevents the occurrence of localized nodules-like corrosion.
Key words: (Ti,Al)N; arc ion plating; oxidation-resistance; corrosion-resistance
1 Introduction
It has been found that serious corrosion appeared on compressor blades of turbine engine operating at 300-600 ℃ after working for a long period of time in marine environment[1]. It’s very necessary to develop a suitable coating which resists against not only oxidation but also erosion for compressor blades. TiN coating is extensively used to increase the lifetime of cutting and forming tools[2-7] due to its high mechanical hardness and low friction coefficient. But TiN can be oxidized rapidly in air at temperature above 550 ℃. (Ti, Al)N ternary solid solution coating of B1NaCl structure has been successfully developed as an alternative coating material for cutting tools for more than ten years due to its superior oxidation-resistance and mechanical properties[8-13]. But there are few reports about this kind coating to be used as oxidation-resistant and corrosion-resistant purpose. In this study, the Ti1-xAlxN coatings with different Al content were deposited on 1Cr11Ni2W2MoV stainless steel for aero-engine compressor blades in an AIP-800-8 coating system and the effects of Al content on the phase structure, oxidation-resistance in air and corrosion-resistance under the synergistic effect of water vapor and NaCl were investigated.
2 Experimental
Titanium aluminum nitride coatings were deposited on 1Cr11Ni2W2MoV stainless steel sheets (15 mm×10 mm×2 mm) by AIP-800-8 Coating System. The steel sheets were prepared by mirror-polishing, followed by ultrasonic cleaning in alcohol and acetone solution. TiN, Ti0.9Al0.1N, Ti0.8Al0.2N, Ti0.7Al0.3N and Ti0.5Al0.5N coat- ings were deposited by using Ti, Ti0.9Al0.1, Ti0.8Al0.2, Ti0.7Al0.3 and Ti0.5Al0.5 targets respectively. Before depos- iting, the substrates were sputter-cleaned using Ar ion under -1 000 V dc bias voltage to remove contaminant layer. The process parameters were listed as following: deposition temperature 400-450 ℃, total pressure 1.2 Pa, N2 partial pressure 0.8 Pa, arc voltage 20 V, arc current 60 A, pulse bias voltage -450 V and duty cycle 30%.
The structural phases of the coatings were char- acterized using an X-ray diffraction (XRD) with Cu Kα radiation. The oxidation was carried out in thermal balance at 700 ℃ and 800 ℃ in air for 10 h. The oxide scale was examined using a field emission SEM. Corros- ion tests under the synergistic of water vapor and NaCl were carried out in a thermal balance in an atmosphere of O2 and water vapor (12.2%), in volume fraction at 600 ℃. NaCl was coated on the preheated specimens surface by brushing with saturated NaCl solution and then drying. The NaCl deposited on the specimens was approximately 0.6 mg/cm2. The equipment and method were described by SHU et al[1].
3 Results and discussion
3.1 XRD characterization of (Ti,Al)N coatings
Fig.1 shows the typical XRD patterns from the deposited Ti1-xAlxN coatings with various Al contents. All of them have the B1 NaCl structure which is the typical phase structure of TiN. The preferred orientation changes from (111) to (220) with increasing Al content. The XRD peaks gradually shift to higher angles as Al content increases, signifying that the lattice parameter decreases with the addition of Al (Fig.2). The Ti1-xAlxN coatings can be considered to be a solid solution in which Al atoms are substituted for Ti sites in the B1 structure. IKEDA et al[2] attributed the decrease of the lattice parameter to the substitution of Al atoms for Ti atoms. WAHLSTR?M et al[14] attributed it to the complex bonding characteristics of the transition metal nitride, where a charge transfer of 3d electrons from Ti to N occurs and the charge transfer is decreased by substi tuting Ti atoms for Al in TiN, resulting in a decrease in the effective radius of N, and thus a decrease in the size
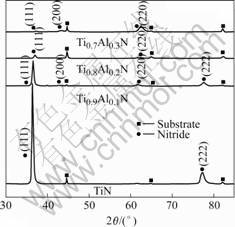
Fig.1 XRD patterns of (Ti,Al)N with different Al contents
of the unit cell.
3.2 Oxidation
Fig.2 shows the oxidation kinetics of TiN and (Ti, Al)N coatings at 700 ℃. The mass gain decreases with increasing Al content. No mass change could be detected for the Ti0.7Al0.3N and Ti0.5Al0.5N coatings and no oxide could be seen on them by SEM. The surface morphologies and cross sectional microstructures of the (Ti, Al)N coatings after 10h oxidation are shown in Fig.3 and Fig.4, respectively. Rutile TiO2 forms on the surface of TiN. Two-phase mixtures of TiO2 and Al2O3 form on the Ti0.9Al0.1N and Ti0.8Al0.2N coatings, as shown by X-ray diffraction and EDX analysis. Fig.3 shows that the oxide crystallites become finer with increasing Al content. The TiN coating is almost completely oxidized to TiO2 and the oxide layer is loose and porous; with increasing Al content, the oxide layer becomes thinner and denser, as shown in Fig.4.
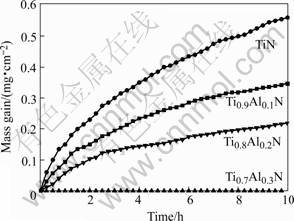
Fig.2 Oxidation kinetics of Ti1-xAlxN coatings at 700 ℃ in air
The surface morphologies of (Ti, Al)N coatings after 10 h oxidation at 800 ℃ are shown in Fig.5. Also, the oxidation-resistance is obviously improved as the Al content in coatings increases.
Auger electron sputter-depth profile of Ti0.5Al0.5N coating after 10 h oxidation at 700 ℃ is shown in Fig.6. As shown in Fig.6, an Al-riched oxide layer forms on the surface of the coating. The results in Fig.7 indicate that a double layer oxide forms on the surface of Ti0.5Al0.5N coating after 10 h oxidation at 800 ℃. The upper layer of the oxide scale is an Al-rich oxide while the lower one is Ti-riched oxide. The analysis result indicates that at 700 ℃ the aluminum diffusion outward through the oxide layer is the rate-limiting step and the oxygen diffusion can be ignored. While at 800 ℃, besides the aluminum
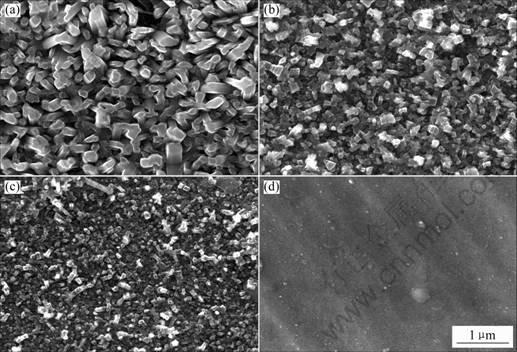
Fig.3 Surface morphologies of (Ti, Al)N coatings after 10 h oxidation at 700 ℃: (a) TiN; (b) Ti0.9Al0.1N; (c) Ti0.8Al0.2N;(d) Ti0.7Al0.3N
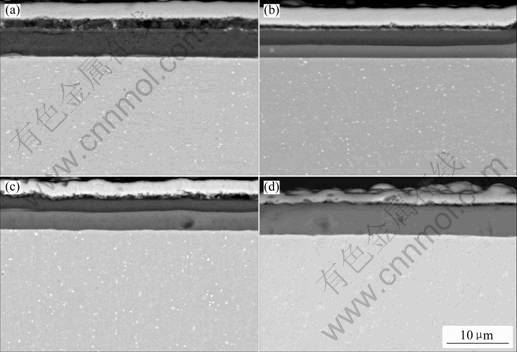
Fig.4 Cross-sectional microstructures of (Ti, Al)N coatings after 10 h oxidation at 700 ℃: (a) TiN; (b) Ti0.9Al0.1N; (c) Ti0.8Al0.2N; (d) Ti0.7Al0.3N
transport, the diffusion of oxygen inward also improves. The oxide bilayer of (Ti, Al)N were formed by the simultaneous outward diffusion of aluminum and the inward diffusion of oxygen. The formation of the Al-riched oxide gives rise to more excellent oxidation- resistance for (Ti, Al)N. The theoretical calculation and XPS measurement show that the oxidation of titanium in Ti1-xAlxN coatings is suppressed because the electric energy of titanium is stabilized owing to addition of Al into TiN[14]. This can explain the improvement of oxidation-resistance of (Ti, Al)N with the increase of Al content when Al content is low and then Al-rich oxide
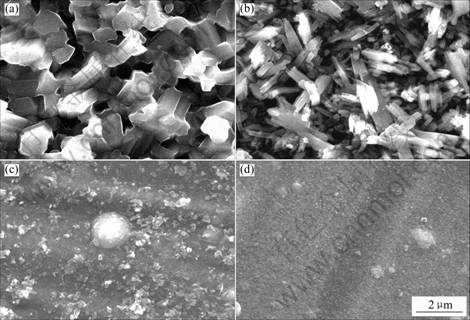
Fig.5 Surface morphologies of (Ti, Al)N coatings after 10 h oxidation at 800 ℃: (a) TiN; (b) Ti0.9Al0.1N; (c) Ti0.7Al0.3N; (d) Ti0.5Al0.5N
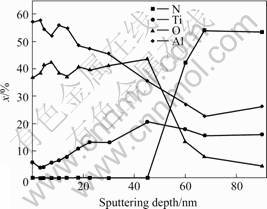
Fig.6 AES depth profile of Ti0.5Al0.5N coating after 10 h oxidation at 700 ℃
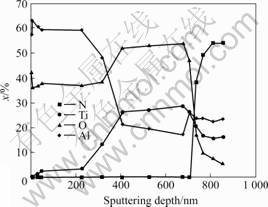
Fig.7 AES depth profile of Ti0.5Al0.5N coating after 10 h oxid- ation at 800 ℃
can not form.
3.3 Corrosion under synergistic of water vapor and NaCl
Fig.8 shows the corrosion kinetics of uncoated and coated 1Cr11Ni2W2MoV steel at 600 ℃. The corrosion of the uncoated steel is much severer than that of the steel coated with TiN or (Ti, Al)N. As shown in Fig.9, the oxide of the steel is rough and irregular. The element mapping of cross section indicates that the out part of the scale is mainly Fe2O3 and followed are successive thinner layers rich in Cr and Fe alternatively[1]. The morphologies of the coated steels after corrosion are
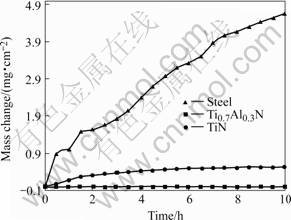
Fig.8 Corrosion kinetics of steel 1Cr11Ni2W2MoV coated with Ti1-xAlxN on synergistic effect of solid NaCl and water vapor
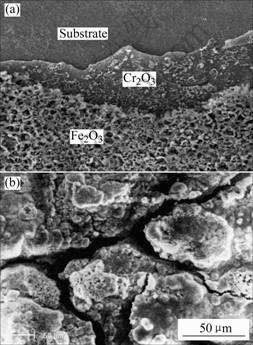
Fig.9 Surface morphologies of 1Cr11Ni2W2MoV steel after 10 h corrosion at 600 ℃ on synergistic effect of NaCl and water vapor
shown in Fig.10. On the TiN surface a lot of nodules appear, which are formed due to the corrosion of the substrate through the pinholes of TiN coating. The fine granules of oxide form on the Ti0.7Al0.3N coating and no obvious change is detected on the Ti0.5Al0.5N coatings. Since the corrosion is very slight, mass change can hardly be manifested on the kinetic curve for both Ti0.7Al0.3N and Ti0.5Al0.5N coatings. The quick formation of thin alumina layer prevents the corrosion. Most importantly, the formation of alumina seals the pinholes or defects in the coatings, which prevents the occurrence of localized nodules-like corrosion.
4 Conclusions
All coatings have a B1 NaCl structure. The preferred orientation changes from (111) to (220) and the lattice parameter of Ti1-xAlxN decreases with the increase of Al content. Oxidation-resistance of the coatings dramatically improves with the increasing of Al content, which is ascribed to the formation of Al-riched oxide on the surface of (Ti, Al)N coatings; TiN and (Ti, Al)N coatings significantly improve the corrosion-resistance of 1Cr11Ni2W2MoV stainless steel under the synergistic of water vapor and NaCl, and the corrosion-resistance becomes better when the Al content increases.
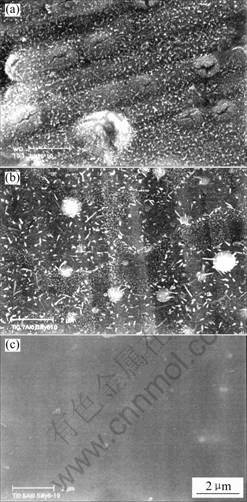
Fig.10 Surface morphologies of 1Cr11Ni2W2MoV steel with coatings after 10 h corrosion at 600 ℃ on synergistic effect of NaCl and water vapor: (a) TiN; (b) Ti0.7Al0.3N; (c) Ti0.5Al0.5N
References
[1] SHU Y H, WANG F H, WU W T. Synergistic effect of NaCl and water vapor on the corrosion of 1Cr-11Ni-2W-2Mo-V steel at 500-700 ℃[J]. Oxidation of Metals, 1999, 51: 97-110.
[2] IKEDA T, SATOH H. Phase formation and characterization of hard coatings in the Ti-Al-N system prepared by the cathodic arc ion plating method [J]. Thin Solid Film, 1991, 195: 99-110.
[3] SANDERW D. Review of ion-based coating processes derived from the catholic arc [J]. Journal of Vacuum Science and Technology A, 1987, 7: 2339-2345.
[4] TUFFY K, BYRNE G, DOWLING D. Determination of the optimum TiN coating thickness on WC inserts for machining carbon steels [J]. Journal of Materials Processing Technology, 2004, 155-156: 1861-1866.
[5] HARRIS S G, DOYLE E D, WONG Y C, MUNROE P R, CAIRNEY J M, LONG J M. Reducing the macroparticle content of cathodic arc evaporated TiN coatings [J]. Surface and Coatings Technology, 2004, 183(2-3): 283-294.
[6] PENG Z, MIAO H, QI L, YANG S, LIU C. Hard and wear-resistant titanium nitride coatings for cemented carbide cutting tools by pulsed high energy density plasma [J]. Acta Materialia, 2003, 5(11): 3085.
[7] CSELLE T, BARIMANI A. Today′s applications and future developments of coatings for drills and rotating cutting tools [J]. Surface and Coatings Technology, 1995, 76-77: 712-718.
[8] RICHTHOFEN A, CREMER R, WITTHAUT M, DOMNICK R, NEUSCH?TZ D. Composition, binding states, structure, and morphology of the corrosion layer of an oxidized Ti0.46Al0.54N film [J]. Thin Solid Film, 1998, 312: 190-194.
[9] COLL B F, FONTANA R, GATES A, SATHRUM P. (Ti-Al)N advanced films prepared by arc process [J]. Mater Sci Eng A, 1991, A140: 816-824.
[10] WUTHER R, YEUNG W Y, PHILLIPS M K, MCCREDIE G. Study on d.c. magnetron sputter deposition of titanium aluminium nitride thin films: effect of aluminium content on coating [J]. Thin Solid Film, 1996, 290-291: 339-342.
[11] SHUM P W, TAM W C, LI K Y, ZHOU Z F, SHEN Y G. Mechanical and tribological properties of titanium-aluminium-nitride films deposited by reactive close-field unbalanced magnetron sputtering [J]. Wear, 2004, 257(9-10): 1030-1040.
[12] LI M S, WANG F H. Effects of nitrogen partial pressure and pulse bias voltage on (Ti, Al)N coatings by arc ion plating [J]. Surface and Coatings Technology, 2003, 167: 197-202.
[13] UCHIDA H, YAMASHITA M, HANAKI S, UETA T. Characterization of (Ti, Al)N films prepared by ion mixing and vapor deposition [J]. Mater Sci Eng A, 2004, A387-389: 758-762.
[14] WAHLSTR?M U, HULTMAN L, SUNDGREN J E, ADIBI F, PETROV I, GREENE J E. Crystal growth and microstructure of polycrystalline Ti1-xAlxN alloy films deposited by ultra-high-vacuum dual-target magnetron sputtering [J]. Thin Solid Films, 1993, 235: 62-70.
[15] ZHOU M, MAKINO Y, NOSE M, NOGI K. Phase transition and properties of Ti-Al-N thin films prepared by r.f.-plasma assisted magnetron sputtering [J]. Thin Solid Films, 1999, 339: 203-208.
(Edited by HE Xue-feng)
Foundation item: Projects (59971052; 50401022) surpported by the National Natural Science Foundation of China
Corresponding author: LI Ming-sheng; Tel: +86-791-3801423; E-mail: mshli@163.com