Trans. Nonferrous Met. Soc. China 22(2012) s364-s369
Formability and microstructure of AA6061 Al alloy tube for hot metal gas forming at elevated temperature
HE Zhu-bin1, FAN Xiao-bo1, SHAO Fei1,2, ZHENG Kai-lun1, WANG Zhi-biao1, YUAN Shi-jian1
1. National Key Laboratory for Precision Hot Processing of Metals, Harbin Institute of Technology, Harbin 150001, China;
2. Luoyang Ship Material Research Institute, China Shipbuilding Industry Corporation (CSIC), Luoyang 471039, China
Received 28 August 2012; accepted 25 October 2012
Abstract: Free bulging test was carried out at different temperatures ranging from 350 °C to 500 °C to evaluate the formability of AA6061 extruded tube, which can provide technology foundation for complex structures forming in hot metal gas forming (HMGF) process. Maximum expansion ratio (MER) and bursting pressure were obtained to evaluate directly the formability at heated conditions. Vickers hardness at different positions was measured. The fracture surface after bursting was observed with scanning electron microscope (SEM), and the microstructure change along axial and hoop directions was analyzed by electron backscattering diffraction (EBSD). The results show that the largest MER value is 86% at 425 °C. Bursting pressure decreases from 4.4 MPa to 1.5 MPa with temperature increasing. The Vickers hardness of fracture position is a little higher than other positions after gas bulging. The fracture mechanism is still the micro-pore aggregation fracture at elevated temperature, while overheated structure appears seriously at 500 °C. The initial fine equiaxial grain grows as temperature increases, which is elongated simultaneously in both axial and hoop directions.
Key words: aluminum alloy tube; free bulging test; hot metal gas forming; formability; microstructure; fracture morphology; grain morphology
1 Introduction
Weight reduction has become one of the research focuses in the field of materials processing, which can be realized by optimized structural design and usage of light materials [1]. Part with irregular cross-sections is manufactured by using light material and optimized structure, which has obtained more and more applications in automotive and aircraft industries [2,3]. Integrated design concept included in light-weight structure leads to the increase of shape complexity. Such parts, especially those with variable cross-sections, are formed exceptionally by internal high pressure forming [4]. However, poor formability of aluminum alloy at room temperature restricts seriously its wide application. One reasonable solution is to form such materials at elevated temperature, because their formability increases obviously with improving forming temperature. Warm hydro-forming using thermal conductive oil has been one promising method to manufacture complex-shaped parts [5-7]. Unfortunately, it was found that warm hydroforming is quite limited, because the heat resistant temperature of available oil is just about 300 °C [7,8].
Nowadays, hyperbaric gas has now become the ideal pressure medium for forming at higher temperature. Hot metal gas forming (HMGF) has been proposed based on superplastic forming and hot blow forming technique [9]. The feasibility for mass productions in automotive industry has been investigated. Without end feeding, about 150% expansion for aluminum alloy tube and 50% expansion for steel tube were achieved when formed at 480 °C and 1100 °C, respectively.
Formability of several engineering metal tubes has been tested in HMGF process [10]. The microstructures of AZ31 magnesium alloy tube before and after deformation have been investigated by EBSD, with special attention on the anisotropic characteristic of extruded tubes [11-13]. The deformation behavior and formability of aluminum alloy tube at room temperature has also been investigated [14,15]. However, there are few results concerning the formability and microstructure change of aluminum alloy tube in HMGF process.
In this work, experimental set-up for tube gas forming at elevated temperature is developed first. Free bulging test is carried out at different temperatures to evaluate the formability of AA6061 extruded tube. The hardness and microstructure change before and after the test are also analyzed. The reasonable temperature range for HMGF of the tested tube is given based on the results.
2 Experimental
2.1 Experimental setup
The tube bulging test was carried out in the platform developed by Engineering Research Center of Hydroforming in Harbin Institute of Technology (ERCH/HIT), as shown in Fig. 1. The tube was placed in the dies and sealed by punches. The upper and lower dies were heated by resistance heating, which act as heat maintainers to heat indirectly the tube, and temperature fluctuation can be controlled effectively. At the same time, the tested tube can be heated directly by induction heating at the position where the deformation is needed. The temperature was controlled by PID regulator with the temperature control accuracy of ±1.0 °C. When the tube was heated to a target temperature, high pressure gas was introduced into the tube until bursting happened. The available highest testing temperature of the experimental set-up is 600 °C, with gas pressure up to 35 MPa.
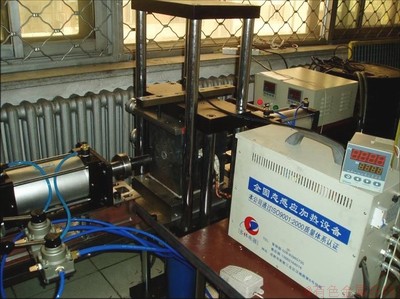
Fig. 1 Experimental setup for hot metal gas forming
2.2 Experimental procedure
In order to investigate the effect of temperature on formability and microstructure in HMGF process, free bulging test at different temperatures ranging from 350 °C to 500 °C has been conducted. The tested material was extruded tube of AA6061 Al alloy in annealed condition, with 27.8 mm in external diameter and 1.8 mm in thickness. The length of free bulging zone is two times of external diameter of the tube.
After each test, maximum expansion ratio and bursting pressure were obtained to evaluate directly the tube formability at elevated temperatures. Vickers hardness (HV0.1) at different positions was measured, with dwell time of 15 s. The measuring position is given in Fig. 2(a). The fracture surface after bursting was observed with scanning electron microscope (SEM), and microstructure change along axial and hoop directions was analyzed by electron backscattering diffraction (EBSD). Figure 2(b) shows the analyzing position of microstructure. The specimen needed to be prepared by electropolishing working at -20 °C within 15 s, with electrolytic solution containing 90% C2H5OH and 10% HCLO4.
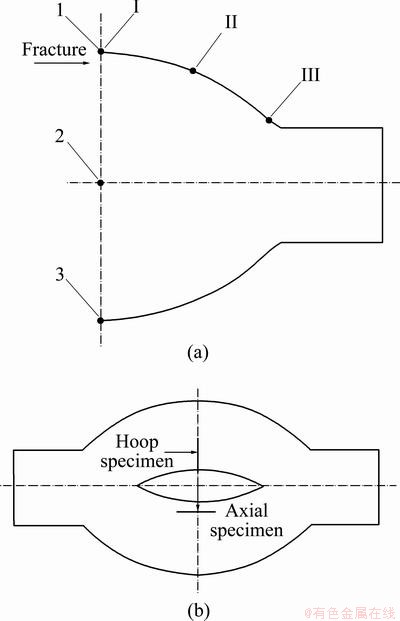
Fig. 2 Positions for hardness (a) and microstructure (b) analysis
3 Results and discussion
Figure 3 shows the tested tube workpieces obtained at different temperatures. Results and discussion are given as follows.
3.1 Bursting pressure
Effect of temperature on deformation resistance can be perceived on the basis of bursting pressure. Figure 4 gives the bursting pressure at different temperatures. It is clear to see that bursting pressure decreases quickly as temperature increases. The bursting pressure is 4.5 MPa when temperature is 350 °C, and bursting pressure decreases to 1.5 MPa when the temperature arrives at 500 °C. At a relatively higher temperature, a low gas pressure is required to form the AA6061 aluminum tube. This means that the deformation resistance can be reduced significantly at the high temperature.
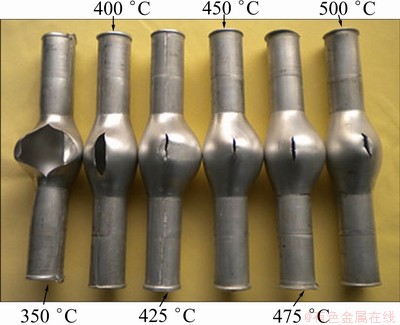
Fig. 3 Workpieces obtained at different temperatures
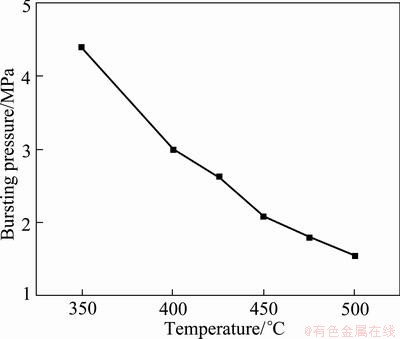
Fig. 4 Effect of temperature on bursting pressure
3.2 Maximum expansion ratio
Figure 5 shows the maximum expansion ratio at different temperatures. It can be found that the maximum expansion ratio increases first and reaches the maximum value of about 86% at 425 °C, then begins to decrease until 65% at 500 °C.
3.3 Vickers hardness
Hardness change can reflect the strength change from another point of view. The Vickers hardness change along axial and hoop directions at different temperatures is given in Fig. 6. The maximum hardness along axial direction appears in the position (point I). The same observation is got along hoop direction. The Vickers hardness near the fracture reaches the maximum value of 42.5, when the temperature is 350 °C. According to the Vickers hardness at different temperatures in the same position, the strength decreases with temperature increasing. Fortunately, the hardness difference is small, which means that a wide temperature range is appropriate for aluminum tube hot gas forming.
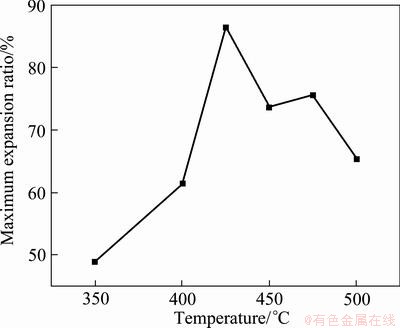
Fig. 5 Effect of temperature on maximum expansion ratio
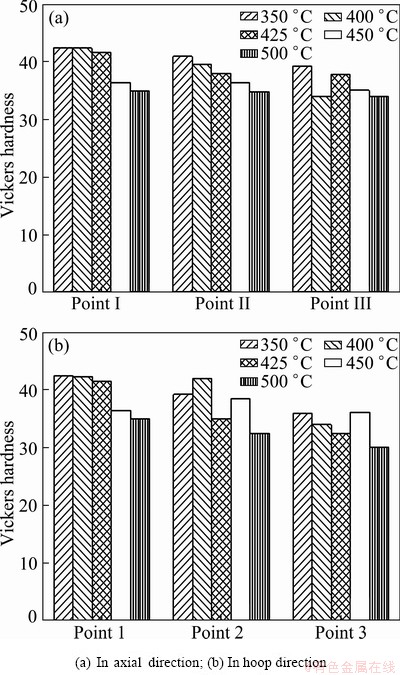
Fig. 6 Vickers hardness at different temperatures
3.4 Fracture morphology
The morphologies of fracture surface after bursting in different conditions observed with SEM are shown in Fig. 7. It can be seen that fracture surfaces are covered completely with dimples at all temperatures. The fracture mechanism is the fracture mode by congregation of micro-cavity. The size and distribution of dimples at 350 °C are uneven, whose depth is relatively shallow. The distribution of dimples tends to become even with temperature increasing, whose depth becomes deeper. This explains the reason of maximum expansion ratio increasing. As the temperature increases further, oxidation and overheating phenomenon are found to appear in the tearing ridge. Therefore, the forming temperature should be lower than 500 °C from the point of view of fracture morphology.
3.5 Microstructure
It can be seen from Fig. 8 that original structure along axial and hoop directions is equiaxed grain structure with uneven size distribution, with average grain size of 30 μm. There is few subgrain structure in equiaxed grain. Grain morphologies obtained by EBSD along axial and hoop directions at different temperatures are shown in Fig. 9, in analyzing position with the maximum amount of deformation. The equiaxed grain grows obviously and is stretched in the deformation direction with increasing the temperature. Abnormal grain growth appears at 500 °C, with size more than 200 μm in elongation direction. The higher the temperature, the larger the elongation. Fibrous tissue begins to appear after 450 °C, which means that a large deformation makes grain orientation tend to be consistent. Meanwhile, a large number of subgrain structures generate inside the grown grains, and the same stretching phenomenon along deformation direction is observed in as-grown grains.
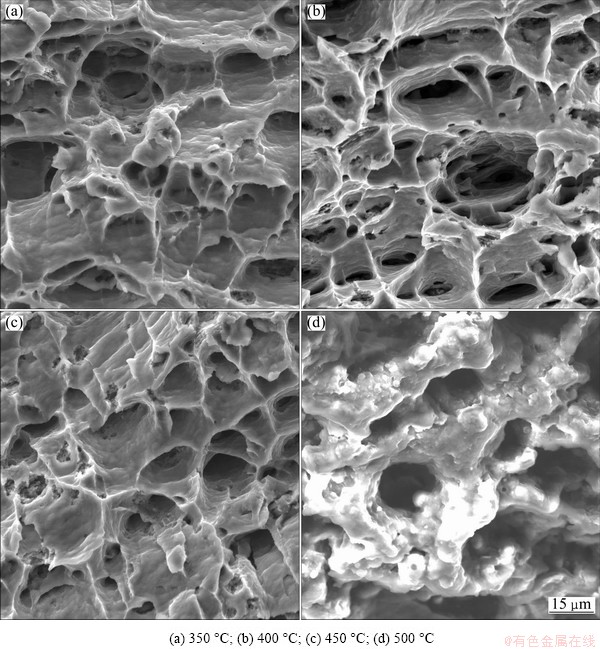
Fig. 7 Fracture morphologies at different temperatures
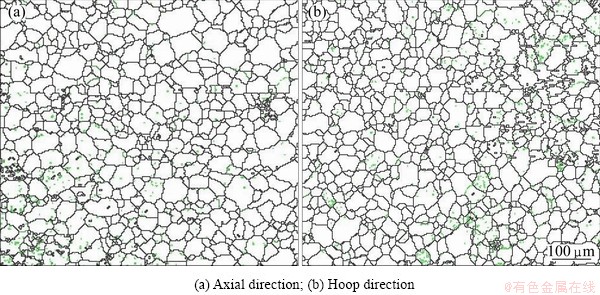
Fig. 8 Original grain structures
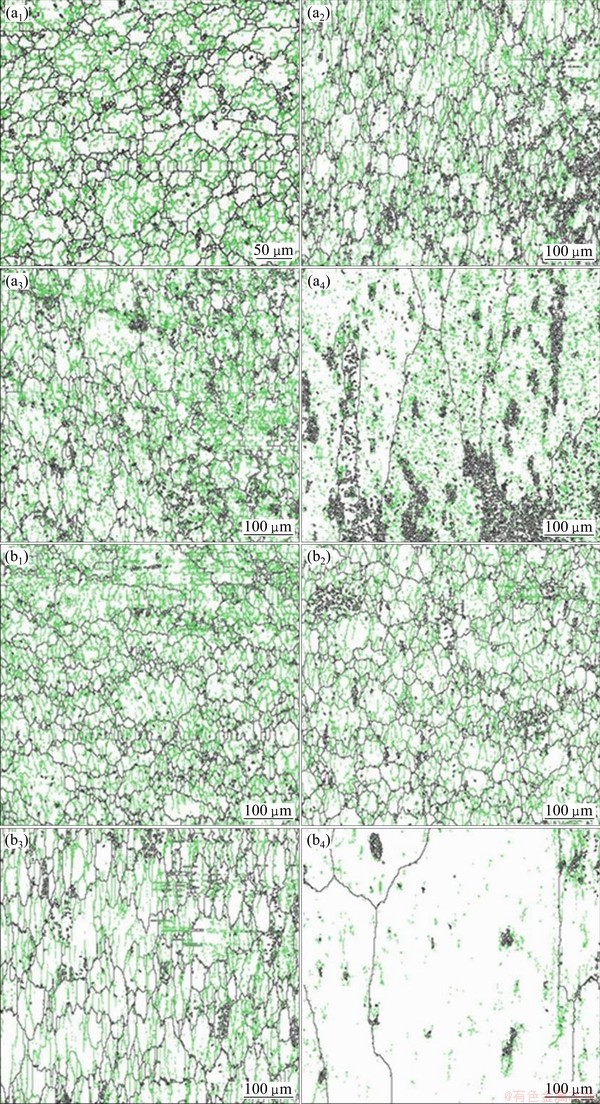
Fig. 9 Grain morphologies at different temperatures along axial direction at 350 °C (a1), 400 °C (a2), 450 °C (a3), 500 °C (a4), and along hoop direction at 350 °C (b1), 400 °C (b2), 450 °C (b3) and 500 °C (b4)
4 Conclusions
1) Bursting pressure decreases monotonously from 4.4 MPa to 1.5 MPa as temperature increases. The maximum expansion ratio increases firstly and reaches the maximum value of about 86% at 425 °C, then begins to drop to 65% at 500 °C.
2) The fracture mechanism is still the micro-pore aggregation fracture at elevated temperature. Oxidation and overheating phenomenon are found to appear in the tearing ridge after 450 °C. Original equiaxed grain is stretched in the deformation direction and grows obviously with increasing the temperature.
3) The ideal forming temperature range of AA6061 Al alloy tube for hot metal gas forming is from 400 °C to 450 °C.
References
[1] HARTL C. Research and advances in fundamentals and industrial applications of hydroforming [J]. Journal of Materials Processing Technology, 2005, 167(2-3): 383-392.
[2] YUAN Shi-jian, HE Zhu-bin, LIU Gang. Warm hydroforming process of light weight alloys [J]. Forging & Stamping Technology, 2005, 30(6): 75-80. (in Chinese)
[3] TOROS S, OZTURK F, KACAR I. Review of warm forming of aluminum-magnesium alloys [J]. Journal of Materials Processing Technology, 2008, 207(1-3): 1-12.
[4] YUAN Shi-jian, HE Zhu-bin, LIU Gang, WANG Xiao-song, HAN Cong. New developments in theory and processes of internal high pressure forming [J]. The Chinese Journal of Nonferrous Metals, 2011, 21(10): 2523-2533. (in Chinese)
[5] KLEINER M, GEIGER M, KLAUS A. Manufacturing of lightweight components by metal forming [J]. CIRP Annals—Manufacturing Technology, 2003, 52(2): 521-542.
[6] Jirang CUI, Hans J ROVEN. Recycling of automotive aluminum [J]. Transactions of Nonferrous Metals Society of China, 2010, 20: 2057-2063.
[7] NOVTNY S, GEIGER M. Process design for hydroforming of lightweight metal sheets at elevated temperatures [J]. Journal of Materials Processing Technology, 2003, 138(1-3): 594-599.
[8] YUAN Shi-jian, QI Jun, HE Zhu-bin. An experimental investigation into the formability of hydroforming 5A02 Al-tubes at elevated temperature [J]. Journal of Materials Processing Technology, 2006, 177: 680-63.
[9] BENEDYK J C. Hot metal gas forming for manufacturing vehicle structural components [J]. Light Metal Age, 2003, 61(11-12): 18-19.
[10] WU X. Elevated temperature formability of some engineering metals for gas forming of automotive structures [J]. Journal of Materials and Manufacture, 2001, 110(5): 1045-1056.
[11] LIU Y, WU X. A microstructure study on an AZ31 magnesium alloy tube after hot metal gas forming process [J]. Journal of Materials Engineering and Performance, 2007, 16(3): 354-459.
[12] HE Zhu-bin, LIU Gang, WU Jia, YUAN Shi-jian, LIANG Ying-chun. Mechanical property and formability of AZ31B extruded tube at elevated temperature [J]. Transactions of Nonferrous Metals Society of China, 2008, 18: 209-213.
[13] LIN Yan-li, HE Zhu-bin, WU Jia, YUAN Shi-jian, Formability determination of AZ31B tube for IHPF process at elevated temperature [J]. Transactions of Nonferrous Metals Society of China, 2011, 21: 851-856.
[14] YANNIS P K, STELIOS K. Hydroforming of anisotropic aluminum tubes: Part I. Experiments [J]. International Journal of Mechanical Sciences, 2011, 53: 75-82.
[15] YANNIS P K, STELIOS K. Inflation and burst of anisotropic aluminum tubes for hydroforming applications [J]. International Journal of Plasticity, 2008, 24: 509-543.
AA6061铝合金管材热态气压成形性能及微观组织
何祝斌1,凡晓波1,邵 飞1,2,郑凯伦1,王志彪1,苑世剑1
1. 哈尔滨工业大学 金属精密热加工国家级重点实验室,哈尔滨 150001;
2. 中国船舶重工集团公司 洛阳船舶材料研究所,洛阳 471039
摘 要:为测试AA6061挤压管材的成形性,在350~500 °C进行自由胀形实验,为复杂结构件的热态金属气压成形(HMGF)提供基础。通过测量最大胀形率(MER)和破裂压力直接表征加热状态下管材的成形性能。测量不同位置的维氏硬度,进行断口的SEM分析,并通过EBSD方法分析沿轴向和环向的微观组织。结果表明,在425 °C下胀形时最大胀形率达到极值86%。随着温度的升高,破裂压力从4.4 MPa降至1.5 MPa。破裂位置的维氏硬度略高于其它部位。在高温下发生微孔聚集型断裂,在500 °C出现过热组织。胀形时随着温度的升高,初始的细小等轴晶粒开始长大并沿轴向和环向被拉长。
关键词:铝合金管;自由胀形实验;热态金属气压成形;成形性;微观组织;断口形貌;晶粒形貌
(Edited by YANG Bing)
Foundation item: Project (NCET-11-0799) supported by the Program of New Century Excellent Talents in University of China; Project (HIT.BRETIII.201204) supported by Fundamental Research Funds for the Central Universities of China; Project (2011BAG03B03) supported by the National High Technology Research & Development Program of China
Corresponding author: HE Zhu-bin; Tel: +86-451-86414751; E-mail: hithe@hit.edu.cn
DOI: 10.1016/S1003-6326(12)61732-4