
Deformation behavior of fine-grained 5083 Al alloy at elevated temperature
ZHANG Kai-feng(张凯锋), YAN Hong-hua(闫宏华)
School of Materials Science and Engineering, Harbin Institute of Technology, Harbin 150001, China
Received 10 June 2009; accepted 15 August 2009
Abstract: The microstructure evolution of the fine-grained 5083 Al alloy was investigated in annealing temperature range of 150-300 ℃. Then the effects of the different annealed microstructures on high-temperature deformation behavior were further studied. The results indicate that the initial recrystallization temperature is about 200 ℃. By tensile tests at 380-570 ℃ and in strain rate range of 4.17×10-4-1.0×10-2 s-1, the optimum superplastic parameters are obtained as follows: the annealed temperature 250 ℃, the tensile temperature 550 ℃ and the strain rate 4.17×10-4 s-1. With the aid of scanning electronic microscopy (SEM), the fractography of the alloy after the superplastic deformation was analyzed. The results reveal that intergranular cavities with fine size and homogeneous distribution are beneficial to superplastic deformation.
Key words: fine-grained 5083 Al alloy; annealing; superplastic deformation
1 Introduction
Al-Mg based 5083 Al alloy has gotten broad applications in commercial superplastic formation for its special characteristics, such as low price, moderate strength, good corrosion resistance and high formability with moderate superplasticity[1-3]. The microstructure with stable fine grain in size less than 10 μm is crucial in superplastic deformation of materials. Many researchers have produced fine-grained microstructure of the alloy via severe plastic deformation (SPD). For instance, the microstructure consisting of (sub)grains of about 0.5 μm in a commercial 5083 Al alloy was obtained using equal channel angular pressing for 8 passes with route Bc[4]. Most of the grains were significantly refined to 0.3 μm using the same method by KO et al[5]. INDRANIL et al[6] utilized gas atomization, cryomilling, consolidation, and extrusion to get the bulk ultrafine-grained 5083 Al alloy with average grain size of 305 nm. Recently, 5083 Al alloys modified with grain refiner, 0.25% Zr and 0.46% Mn, were processed by reciprocating extrusion to yield an equiaxed grain structure with the grain size of about 4.5 μm[7]. JIANG et al[8] reported that after ECAP at 350 ℃ for 6 passes, fine-grained structure with (sub)grain size of about 2 μm is obtained. However, some drawbacks of fine-grained 5083 Al alloy obtained by using SPD method have been reported in Ref.[9], such as internal stress. This issue inevitably increases the trend of stress corrosion. Thus, it attenuates stability of microstructure and mechanical properties of materials. In addition, the internal stress has a pernicious influence on the superplastic formation of materials. In this work, the effects of annealing temperature on the microstructure and deformation behavior at elevated temperature for the fine-grained 5083 Al alloy are investigated.
2 Experimental
The commercial 5083 (H112 condition) Al alloy plates (Al-4.56%Mg-0.61%Mn) with 6.2 mm in thickness was firstly annealed at 540 ℃ for 2 h to fully remove defects, and then cold rolled with reduction in pass of around 22%. Rolling pass was repeated until total reduction ratio arrived at 77.42%. Finally, the fine-grained 5083 Al alloy was annealed at 150-300 ℃ for 30 min and the microstructure evolution was observed.
The microstructure evolution of the fine-grained sheet during annealing was examined using transmission electron microscopy. Thin foils vertical to rolling plane of the sheet and along rolling direction were prepared by a twin-jet polishing technique using a mixture of 30% nitric acid and 70% methanol at -30 ℃.
Uniaxial tensile tests were carried out with the loading direction parallel to the rolling direction on a
testing machine equipped with a three-zone furnace. Tensile specimens with 10 mm in length (excluded round angle) and 4 mm in width were machined from the fine-grained alloy sheet of 1.4 mm in thickness and were tested at 380-570 ℃ and strain rate range of 4.17×10-4-1.0×10-2 s-1. The fractographies of tensile specimens were analyzed by SEM.
3 Results and discussion
3.1 Microstructure evolution
Fig.1 shows the unannealed and annealed microstructures of the fine-grained 5083 Al alloy. The unannealed microstructure mainly consists of severely elongated grains along the rolling direction. These grains are mainly made of parallel bands of elongated substructures with high density dislocation, as shown in Fig.1(a). When the specimen of the fine-grained 5083 Al alloy is annealed at 150 ℃, the partial subgrains form due to the polygonization of dislocations, and many of elongated substructure boundaries are recovered into subgrain boundaries (Fig.1(b)). This represents incomplete recovery characteristic including the formation of partial subgrain and the rearrangement of some dislocations. No recrystallization is found at this temperature. When the alloy is annealed at 200 ℃, the
Fig.1 Unannealed and annealed microstructures of fine-grained 5083Al alloy: (a) Unannealed microstructure; (b) Incompletely recovered microstructure annealed at 150 ℃; (c) Duplex microstructure of recovered and freshly recrystallized microstructure annealed at 200 ℃; (d) Fully recrystallized microstructure annealed at 250 ℃; (e) Coarsened microstructure annealed at 300 ℃
boundaries within subgrains are further formed due to the rearrangement of dislocations. Meanwhile, the width of subgrain increases slightly. This leads to the reduction of the subgrain aspect ratio. Additionally, a small quantity of nearly equiaxed fine fresh-grains is observed at this temperature (see arrow direction in Fig.1(c)). The microstructure mainly consisted of distinct-boundary subgrains and small quantity of fresh grains. Namely, complete recovery and initial recrystallization occur at 200 ℃. Fig.1(d) shows that the fully recrystallized microstructure consists of equiaxed free-dislocation grains in the alloy annealed at 250 ℃. Most equiaxed grains reveal fully recrystallizing and coarsening grains to some extent. When the temperature is 300 ℃, grains severely grow up so that a whole grain is not observed in a field of vision, as shown in Fig.1(e).
3.2 Deformation at elevated temperature
To determine the optimum superplastic temperature, tensile tests on the specimens annealed at 250 ℃ were conducted under condition of 380-570 ℃ and strain rate of 4.17×10-4 s-1. The stress—strain curves of the tensile tests are shown in Fig.2. The amplitude of flow stress decreases with tensile temperature increasing. When the temperature is less than 500 ℃, the flow stress reaches a maximum value quickly due to the strain hardening, and then decreases continuously until fracture. A well-defined peak in flow stress could be observed. No steady-state flow occurs. At 500 ℃, strain hardening and strain softening alternate. The flow stress fluctuates in small variation range. At 550 ℃, the stress—strain curve displays classical superplastic steady-state flow. However, the curve fluctuates in wide variation range and no steady-state flow is found with temperature up to 570 ℃, since it is close to the incipient melting temperature of 574 ℃ of the alloy[10]. Partial macroscopical melt of the materials causes some grain
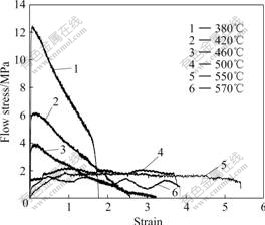
Fig.2 Stress—strain curves of specimen annealed at 250 ℃ tested under conditions of 380-570 ℃ and strain rate of 4.17×10-4 s-1
boundaries that they could not support the applied tensile force[11]. Hence, the optimum superplastic temperature is 550 ℃. To obtain the optimum strain rate, the tensile tests in specimens annealed at 250 ℃ were carried out under conditions of 550 ℃ and strain rate range of 4.17×10-4-1.0×10-2 s-1. The fractured specimens after tension are shown in Fig.3. It can be found that elongation-to-failure increases with strain rate decreasing and no necking occurs on the specimens pulled to failure in the testing strain rate range. The maximum 570% in elongation-to-failure is obtained at strain rate of 4.17×10-4 s-1. The result indicates that atom has enough time to diffuse at low strain rate during superplastic deforming.
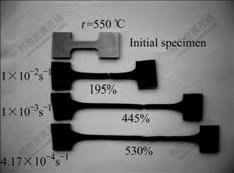
Fig.3 Fractured specimens annealed at 250 ℃ tested under conditions of strain rate range of 4.17×10-4-1.0×10-2 s-1 and 550 ℃
Further tensile experiments were carried out under optimum superplastic conditions, i.e., 550 ℃ and strain rate of 4.17×10-4 s-1, to investigate the influence of annealed temperature on superplastic deformation behavior of the alloy. The specimens annealed at 150-300 ℃ were employed. Fig.4 shows the fractured specimens. It can be seen that the elongation enhances
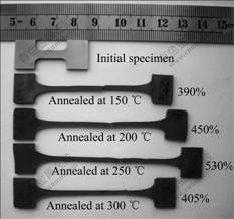
Fig.4 Fractured specimens annealed at different temperatures tested under conditions of 550 ℃ and strain rate of 4.17×10-4 s-1
with annealing temperature increasing, however, it reversely decreases as annealing temperature arrives at 300 ℃. This is related to the annealing microstructures under different temperatures. When the alloy is annealed at 150 ℃, the incomplete recovery microstructure including some subgrains and high-density dislocations is not beneficial to superplastic deformation. This is because that internal stresses caused by repeated cold-rolling still remain at inner of the specimens annealed at 150 ℃. Indeed, the existence of fine equiaxed grains is known to enhance superplastic elongation. However, a small quantity of equiaxed grains produced during annealing at 200 ℃ is not anticipated to greatly improve the elongation in the present case[12]. Usually, the elongation is related to the presence of the considerable amount of fine equiaxed free-dislocation grains[13-14]. In other words, tensile elongation can be effectively improved by the increment of the amount of equiaxed free-dislocation grains. It is obvious that high uniform elongation in the specimen annealed at 250 ℃ attributes to the increasing amount of fine equiaxed free-dislocation grains and fully removing of the internal stress. The microstructure consisting of severely coarsening grains is detrimental to superplastic deformation when the specimens are annealed at 300 ℃.
3.3 Fracture analysis
Fig.5 shows the fractographies of the specimens annealed at different temperatures when tensile tests were carried out under conditions of 550 ℃ and 4.17×10-4 s-1. Many cavities are observed using SEM in fracture of the specimens. Generally, the nucleation of cavities will occur when superplastic deformation reaches to some extent. Cavities will grow up and link together with the deformation. This process finally results in the fracture of materials. However, the cavities uniformly-distributed with fine and dispersal condition is beneficial to grain boundary sliding (GBS). This is because that the stress concentration caused by GBS can be relaxed through presence of the cavities[15]. In contrast, a large number of cavities with larger size, which present in the materials after deformation, can reduce strength of the materials especially for V-shape cavities with rather large size[16]. In Fig.5(a), cavities with non-uniform size are unevenly distributed on the fracture of specimen annealed at 150 ℃. In this case, the elongation of the specimen is not high. To contrast Fig.5(b) and Fig.5(c), the size of cavities in the specimen annealed at 250 ℃ is more fine and uniform than that at 200 ℃. Namely, because the cavities with more small and uniform size individually distribute at 250 ℃, the superplastic elongation at 250 ℃ is higher. In Fig.3(d), the numerous V-shape cavities with large-size are observed. This result further reveals that the presence of large V-shape cavities is detrimental to superplastic deformation when specimen is annealed at 300 ℃.
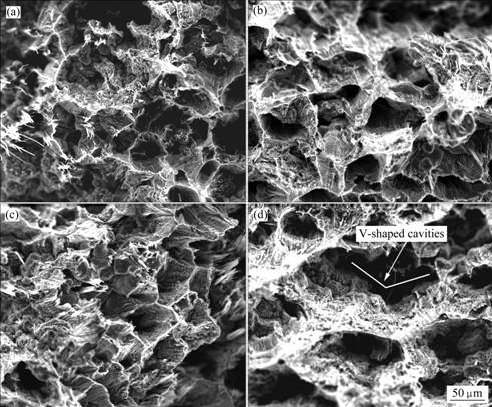
Fig.5 Fractographies of specimens annealed at different annealing temperatures tested under conditions of 550 ℃ and strain rate of 4.17×10-4 s-1: (a) 150 ℃; (b) 200 ℃; (c) 250 ℃; (d) 300 ℃
4 Conclusions
1) The microstructures of the fine-grained 5083 Al alloy display incomplete recovery annealed at 150 ℃, the coexistence of complete recovery and initial recrystallization at 200 ℃, the coexistence of completed recrystallization and coarsening grain at 250 ℃ and severely coarsening grain at 300 ℃. Simultaneously, the results show that the initial recrystallization temperature of the fine-grained alloy is about 200 ℃.
2) The tensile tests on the specimen annealed at 250 ℃ were carried out at 380-570 ℃ and strain rate range of 4.17×10-4-1.0×10-2 s-1. The experimental results indicate that the optimum superplastic parameters are 550 ℃ and 4.17×10-4 s-1.
3) The results of tensile tests at the optimum parameters reveal that the superplastic deformation behavior of the specimen annealed at 250 ℃ is better than others.
4) Fracture analysis of the specimens annealed at different temperatures implies that intergranular cavities with fine size and well distribution are beneficial to superplastic deformation. However, the intergranular V-shape cavities with large size have a pernicious influence on the deformation behavior.
References
[1] CHEN H H, WANG J Y, LEE J, LEE S. Superplasticity of AA5083 alloy as processed by equal channel angular extrusion [J]. Journal of Alloys and Compounds, 2008, 460: 305-308.
[2] PARK K T, HWANG D Y, LEE Y K, KIM Y K, SHIN D H. High strain rate superplasticity of submicrometer grained 5083 Al alloy containing scandium fabricated by severe plastic deformation [J]. Materials Science and Engineering A, 2003, A341: 273-281.
[3] KAIBYSHEV R, MUSIN F, LESUER D R, NIEH T G. Superplastic behavior of an Al-Mg alloy at elevated temperatures [J]. Materials Science and Engineering A, 2003, A342: 169-177.
[4] PARK K T, PARK J H, LEE Y S, NAM W J. Microstructures developed by compressive deformation of coarse grained and ultrafine grained 5083 Al alloys at 77 K and 298 K [J]. Materials Science and Engineering A, 2005, A408: 102-109.
[5] KO Y G, SHIN D H, PARK K T, LEE C S. Superplastic deformation behavior of ultra-fine-grained 5083 Al alloy using load-relaxation tests [J]. Materials Science and Engineering A, 2007, A449/451: 756-760.
[6] INDRANIL R, MANISH C, ENRIOUE J L, FARGHALLI A M. Thermal stability in bulk cryomilled ultrafine-grained 5083 Al alloy [J]. Metallurgical and Materials Transactions A, 2006, 37A: 721-730.
[7] LEE S W, YEH J W. Superplasticity of 5083 alloys with Zr and Mn additions produced by reciprocating extrusion [J]. Materials Science and Engineering A, 2007, A460/A461: 409-419.
[8] JIANG Da-ming, NING Jiang-li, SUN Jian-feng, HU Zhi-min, HOU Yi. Annealing behavior of Al-Mg-Mn alloy processed by ECAP at elevated temperature [J]. Trans Nonferrous Met Soc China, 2008, 18: 248-254.
[9] COURTNEY T H. Mechanical behavior of materials [M]. Beijing: China Machine Press, 2004: 179-186.
[10] WANG Zhu-tang, TIAN Rong-zhang. Aluminum alloy and its processing handbook [M]. Changsha: Central South University Press, 2000: 232-234.
[11] HIGASHI K, NIEH T G, MABUCHI M, WADSWORTH J. Effect of liquid phases on the tensile elongation of superplastic aluminum alloys and composites [J]. Scripta Metallurgica et Materialia, 1995, 32(7): 1079-1084.
[12] LEE Y B, SHIN D H, PARK K T, NAM W J. Effect of annealing temperature on microstructures and mechanical properties of a 5083 Al alloy deformed at cryogenic temperature [J]. Scripta Materialia, 2004, 51: 355-359.
[13] CHANG S Y, AHN B D, HONG S K, KAMADO S, KOJIMA Y, SHIN D H. Tensile deformation characteristics of a nano-structured 5083 Al alloy [J]. Journal of Alloys and Compounds, 2005, 386: 197-201.
[14] HAN B Q, HUANG J Y, ZHU Y T, LAVERNIA E J. Strain rate dependence of properties of cryomilled bimodal 5083 Al alloys [J]. Acta Materialia, 2006, 54: 3015-3024.
[15] SUN P H, WU H Y, LEE W S, SHIS S H, PERNG J Y, LEE S. Cavitation behavior in superplastic 5083 Al alloy during multiaxial gas blow forming with lubrication [J]. International Journal of Machine Tools & Manufacture, 2009, 49: 13-19.
[16] WU S D. Superplasticity deformation theory of metals[M]. Beijing: Defense Industry Press, 1997: 102-105.
(Edited by CHEN Ai-hua)
Corresponding author: ZHANG Kai-feng; Tel: +86-451-86413911; E-mail: kfzhang@hit.edu.cn