
Effects of vibration and grain refiner on microstructure of semisolid slurry of hypoeutectic Al-Si alloy
ZHAO Jun-wen(赵君文), WU Shu-sen(吴树森), XIE Li-zhi(谢礼志), AN Ping(安 萍), MAO You-wu(毛有武)
State Key Lab of Materials Processing and Die & Mould Technology,
Huazhong University of Science and Technology, Wuhan 430074, China
Received 31 October 2007; accepted 15 February 2008
Abstract: The effects of vibration and grain refiner on the microstructure of semisolid slurry of hypoeutectic Al-Si alloy were studied. The impact of vibration on the convection of liquid was conducted by using a system of water-particle tracer. The 356 melt at temperature of 630-660 ℃ with or without grain refiner Al-5%Ti-1%B was poured into a metal cup as the vibrating vessel, then it was cooled to 590-610 ℃ in the semisolid zone and kept for some time, subsequently vibration with different frequencies was applied. The results show that the primary α(Al) particles become finer and rounder with the increase of vibration frequency. The slurry with primary α(Al) equivalent particle diameter(EPD) of about 90 μm and average shape coefficient(ASC) of about 0.5 can be prepared under vibration of 20 Hz. With the combined action of vibration and grain refiner Al-5Ti-B, even smaller and rounder spheroids with EPD of about 85 μm and ASC of about 0.6 are obtained.
Key words: mechanical vibration; hypoeutectic Al-Si alloy; semisolid metal; grain refiner
1 Introduction
Over the last decade, a lot of attentions were attracted to metal rheoforming process[1-6], as it has many advantages, such as high mechanical properties of formed components, low cost and easy operation. As a result, a number of novel slurry preparation methods and rheoforming techniques have been invented, for example, semi-solid rheocasting[1], new rheocasting[7-8], liquidus casting[9], twin-screw rheomoulding[10], rotating barrel rheomoulding[11], swirled enthalpy equilibration device[12] and controlled nucleation method[13]. Nevertheless, efforts were still made to develop new ways of preparing semi-solid slurry with lower cost and simpler process[14]. As vibration is easy to operate and needs less expensive equipment, it could be an alternative for preparing semisolid slurry.
Although it was explored to prepare semisolid slurry with ultrasonic vibration[15], little study has been performed on preparing slurry of semisolid metal by mechanical vibration with a frequency less than 50 Hz. What’s more, the combined effects of vibration and refiner on microstructure of semisolid slurry have been little studied. Therefore, this work aims at studying the effect of low-frequency vibration, grain refiner and their combined effects on microstructure of hypoeutectic Al-Si alloy.
2 Experimental
The self-developed equipment shown in Fig.1 was employed in present trials, which consists of vibration controller, vibrating apparatus(VA), heating furnace, PID temperature controller, etc. The simplified schematic of the VA is shown in Fig.2. It consists of a vibration motor attached to the vibrating vessel via a vessel holding table supported by a group of springs. The vibrating vessel shall be fixed by bolts on the vessel holding table of the VA. The vibrating time, frequency and amplitude of the VA may be adjusted by the vibration controller. The preheating temperature and isothermal holding temperature can be controlled by the PID temperature controller.
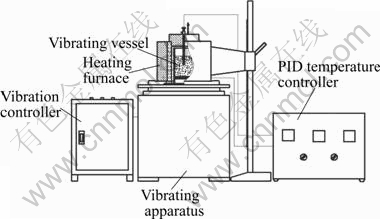
Fig.1 Equipment for mechanical vibration
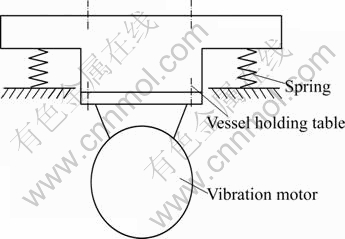
Fig.2 Simplified schematic diagram of vibrating apparatus
The raw material used in this study was commercial hypoeutectic Al-Si alloy 356 with a chemical composition (mass fraction) of 7.2% Si, 0.43% Mg, 0.29% Fe and balance Al. According to Ref.[16], its solidus and liquidus are approximately 554 ℃ and 614 ℃, respectively.
The ingot was melted in a resistance furnace. After the melt was heated to 750 ℃, it was degassed for 15 min with argon gas through a graphite lance. To examine the combined effects of grain refiner and vibration on the microstructure, 0.2% Al-5%Ti-1%B was added into the melt in one group of experiment. The metal vessel was preheated to 530 ℃ by the heating furnace. After degassing the melt was cooled to a temperature of 630-660 ℃. Then the VA was initiated and a certain amount of melt was poured into the vessel. The poured melt would be soon cooled down to 590- 610 ℃ through thermal balance between the melt and the vibrating vessel. At predetermined temperature and vibration time, some of the melt was subsequently drawn with quartz tube in 6 mm diameter and quenched in room temperature water.
The quenched rods were cut, polished and etched by 0.5% hydrofluoric acid solution, and the microstructure was observed and analyzed.
The size of the primary α(Al) particles is estimated by equivalent particle diameter(EPD) defined as
(1)
where LT is the overall length of measured lines used in the software, N is the number of particles passed through by the measured lines.
The shape of a primary α(Al) particle is characterized by shape coefficient, which is defined as
(2)
where A is the cross section area of a specific particle in the micrograph, and Lp is the circumference of the corresponding particle. SF varies in the range of 0-1. The greater the value of SF is, the closer to a round the particle is. The average
is regarded as the arithmetic average of shape coefficient of all the particles in a metallograph, which is named as average shape coefficient(ASC).
A group of physical simulation experiment utilizing a system of water-particle tracer was conducted in order to obtain some perceivable and qualitative information regarding the impact of vibration on the convection of liquid. A transparent plastic beaker with 60 mm in diameter was fastened to the top table of the VA, in which water of 40 mm in depth and polystyrene particles serving as tracing particles with diameter of about 360 μm and with density close to that of water were added.
3 Results and discussion
3.1 Simulation with water and tracing particles
The vibration amplitude of the equipment was about 1.5 mm, and the vibration frequency of the VA varied from 8 Hz to 42 Hz.
Fig.3 shows a series of streak photographs of particles movement in water under vibration with different frequencies. It is observed that flow is generated from the free surface of the fluid and spreads downwards, and the surface moves most intensively, which confirms the statement in Ref.[17]. When the liquid is kept quiescent, i.e. at 0 Hz, parts of the particles are uniformly suspended in the water while majority of them float on the free surface. At 8 Hz, some particles are transmitted to the deep place in liquid whereas not able to arrive to the bottom of the container, as well as the liquid surface fluctuates moderately. When the frequency is increased to 13 Hz, more particles are carried into the deeper liquid and the free surface moves severely. When the frequency reaches 17 Hz, the water and particles are completely mixed and the water gets so turbulent that the whole volume of liquid presents white, and this kind of unstable and strong convection lasts over19 Hz. At 20 Hz, the surface begins to move stably. Thereafter, with the increase of frequency, the surface goes more stable, and the particles carried to the bottom or lower parts of the container are reduced. Nevertheless, the particles still move without any regularity in the whole volume of liquid, which implies a turbulent convection for the bulk flow. In addition, the calculation of particle velocity was made by software based on the principle of Particle Image Velocimetry. And the average particle velocity above the frequency of 12 Hz was estimated to be 60-150 mm/s.
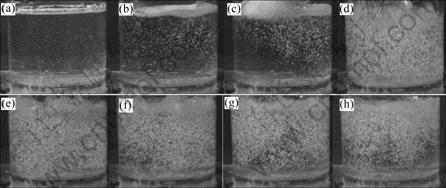
Fig.3 Particles movement in water under vibration with different frequencies: (a) 0 Hz; (b) 8 Hz; (c) 13 Hz; (d) 17 Hz; (e) 20 Hz; (f) 25 Hz; (g) 30 Hz; (h) 42 Hz
3.2 Effects of vibration frequency on microstructure of semisolid 356 slurry
From the physical simulation experiment, it can be concluded that the vibration frequency is a critical parameter in preparing slurry. In this group of experiment, four frequencies were adopted, 0, 12, 20 and 35 Hz, and the processing temperature and time were 605 ℃ and 5 min, respectively.
Fig.4 displays the comparison of the obtained microstructure with different frequencies, and Fig.5 shows the analysis result of the EPD and ASC. It can be seen that, when vibration is not imposed, the primary phase is dendrite with an EPD of more than 220 μm and a rather low ASC of 0.18. With the increase of frequency, the dendritic structure converts to rosette and globular. At 12 Hz, the EPD is only half of that without vibration, i.e. 110 μm, while the ASC is more than twice of that without vibration, i.e. 0.43. At the frequency of 20 Hz, the EPD of non-dendritic α(Al) crystals is 90 μm, and the ASC is 0.53. When the frequency is higher than 20 Hz, the EPD increases a little while the ASC decreases a little compared with that at 20 Hz. Moreover, it is indicated in Fig.4 that the non-dendritic primary α(Al) phase homogenously distributes and is fine with introduction of vibration.
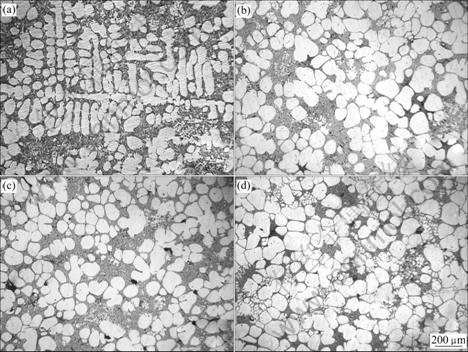
Fig.4 Microstructures of semisolid 356 slurry processed by different frequency vibration: (a) 0 Hz; (b) 12 Hz; (c) 20 Hz; (d) 35 Hz
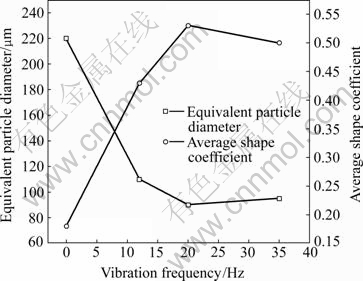
Fig.5 Effect of vibration frequency on EPD and ASC
OHNO[18] examined the effect of low-frequency vibration on the metal solidification and argued that vibration affects the microstructure mainly from two aspects, that is, enhancing the cooling efficiency through resulted good contact of melt and wall of container, and increasing the number of nuclei formed on the wall and liquid surface, detached and brought to the bulk melt. In addition, the particles can be refined by thermal fluctuation and nuclei multiplication in convection induced by oscillation. For producing slurry by vibration, multiplication and refinement of particles is further undergone in the course of isothermal holding. With the increase of vibration intensity, it is more effective that the nuclei generated at the wall and liquid surface would be brought to the bulk melt due to more intense turbulence in the melt. What’s more, the temperature and mass distribution become uniform due to the convection of the melt and isothermal holding, thus the dendritic formation and growth is depressed, which results in the round and refined particles.
Further understanding of the effect of vibration on ASC can be achieved by an equation proposed by WU et al[2], which relates the particle shape fraction with flow speed of fluid relatively to the particle, that is
(3)
where fi is the particle shape fraction, and fi=1 for a circle; R is the solidification rate, and v is the flow speed of fluid relative to the particle.
Solidification speed is affected by several factors such as latent heat and heat release conditions. In experiment, it is reasonable to assume that the solidification rate is constant. For the flow speed, with the increase of vibration frequency, it will become increasingly higher as the vibration becomes more intense. Therefore, it can be deduced that fi becomes larger from the above-mentioned equation. Since the ASC is the arithmetic average of shape fraction of all particles, it will accordingly become larger.
3.3 Effects of combined action of vibration and grain refiner on microstructure of semisolid 356 slurry
Fig.6 shows the microstructure of semisolid 356 slurry with only action of grain refiner. It can be seen that, compared with Fig.4(a), the primary particles have much less dendritic. However, most of the particles are not globular enough and not uniformly distributed. On the other hand, as can be observed in Fig.7, with the combined action of vibration and grain refiner, the microstructure of the slurry distributes well, and the primary particles are fine and spherical.
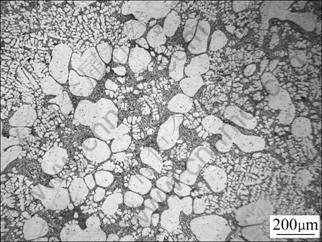
Fig.6 Microstructure of semisolid 356 slurry only with grain refiner
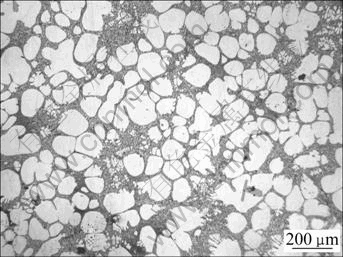
Fig.7 Microstructure of 356 slurry with combined action of grain refiner and vibration (20 Hz)
The effects of combined action of vibration and grain refiner on microstructure of semisolid 356 slurry are shown in Fig.8. With only action of grain refiner (at 0 Hz), the EPD is decreased by 36.4%, namely, from 220 μm to 140 μm, and the ASC is increased from 0.18 to 0.3. When the combined action of grain refiner and vibration is imposed, the EPD of the primary phase is reduced to100 μm at 12 Hz. At 20 Hz the minimum of EPD and maximum of ASC are gained, i.e. 85 μm and 0.61 respectively. In general, there is similar regularity of the ASC and EPD for the semisolid microstructure either with only action of vibration or with combined action of vibration and grain refiner. In addition, it is also revealed in Fig.8 that the EPD and the ASC with combined action of vibration and grain refiner are decreased and increased respectively at the same frequency.
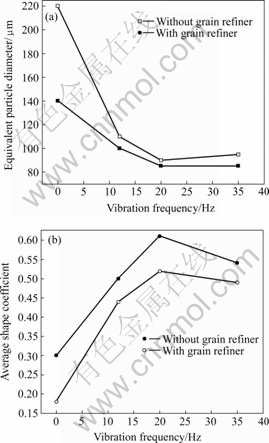
Fig.8 Effects of combined action of vibration and grain refiner on EPD (a) and ASC (b)
Since many heterogeneous nuclei such as TiAl3 and TiB2 are created prior to the formation of α(Al) with the addition of Al-5Ti-B, and most of them become the base of heterogeneous nucleation, the nucleation probability of primary phase is enhanced. At a certain temperature, the solid fraction is relatively stable, therefore, the increasing nuclei will result in smaller particles. When the melt is subject to vibration, the nuclei formed on the wall will be broken off. Furthermore, those nuclei distribute well in the bulk and are not easy to agglomerate. Consequently, the particles become finer and rounder with the combined action of vibration and grain refiner.
4 Conclusions
1) Intense convection can be caused in melt by vibration, which is generated from the free surface of the bulk melt and spreads downwards, consequently leading to the convection in the bulk.
2) Non-dendrite primary α(Al) crystals become finer and rounder with the increase of vibration, and slurry can be prepared with EPD of primary α(Al) about 90 μm and ASC above 0.5 under vibration of 20 Hz.
3) Rounder and finer spheroids, namely EPD of about 85 μm and ASC of about 0.6, are obtained with the combined action of vibration and grain refiner Al-5Ti-B.
References
[1] MARTINEZ R A, FLEMINGS M C. Evolution of particle morphology in semisolid processing [J]. Metall Mater Trans A, 2005, 36 (8): 2205-2210.
[2] WU Shu-sen, WU Xue-ping, XIAO Ze-hui. A model of growth morphology for semi-solid metals [J]. Acta Materialia, 2004, 52: 3519-3524.
[3] WU Shu-sen, ZHAO Jun-wen, WAN Li, LUO Ji-rong. Numerical simulation of mould filling in rheo-diecasting process of semi-solid magnesium alloys [J]. Solid State Phenomena, 2006, 116/117: 554- 557.
[4] MAO Wei-min, ZHAO Ai-min, YUN Dong, ZHONG Xue-you. Preparation study of semisolid 60Si2Mn spring steel slurry [J]. Acta Metallurgica Sinica, 2003, 16(6): 83-488.
[5] WANG T, PUSTAL B, ABONDANO M, GRIMMIG T, B?HRIG- POLACZEK A, WU M, LUDWIG A. Simulation of cooling channel rheocasting process of A356 aluminum alloy using three-phase volume averaging model [J]. Trans Nonferrous Met Soc China, 2005, 15(2): 390-394.
[6] GUO Hong-min, YANG Xiang-jie. Preparation of semi-solid slurry containing fine and globular particles for wrought aluminum alloy 2024 [J]. Trans Nonferrous Met Soc China, 2007, 17(4): 799-804.
[7] UBE Industries Ltd. Method and apparatus for shaping semisolid metals. EPO 745694A1 [P]. 1996.
[8] TOSHIO H, KAPRANOS P. Simple rheocasting processes [J]. Journal of Materials Processing Technology, 2002, 130/131: 594- 598.
[9] PAN Ye, AOYAMA S, LIU Chi. Spherical structure and formation conditions of semi-solid Al-Si-Mg alloy [C]// SUN Guo-xiong, YUAN Hao-yang, YAO Rui-bo. Proceeding of the 5th Asian Foundry Congress. Nanjing: Southeast University Press, 1997: 443- 451.
[10] XIAO Ze-hui, LUO Ji-rong, WU Shu-sen, LI Dong-nan. Performance of semi-solid slurry produced by twin-screw stirring mixer and rheo-diecasting process of AZ91D alloy [J]. Journal of Wuhan University of Technology-Mater Sci Ed, 2004, 19(3): 81-85.
[11] KANG Yong-lin, AN Lin, WANG Kai-kun, SUN Jian-lin, XIAO Bang-guo, XU Chen-yang. Experimental study on rheoforming of semi-solid magnesium alloys [C]// TSUTSUI Y, KIUCHI M, ICHIKAWA K. Proceeding of the 7th on Semi- solid Processing of Alloys and Composites. Tsukuba, Japan, 2002: 287-292.
[12] DOUTRE D, HAY G, WALES P. Semi-solid concentration processing of metallic alloys. US6428636 [P]. 2002.
[13] WANG H, NING Z L, YAO X D, DAVIDSON C J, JOHN D S. Thixotropic structure formation in hypoeutectic Al-Si alloys by controlled nucleation [J]. Materials Forum, 2004, 28: 1316-1321.
[14] WANNASIN J, MARTINEZ R A, FLEMINGS M.C. A novel technique to produce metal slurries for semi-solid metal processing [J]. Solid State Phenomena, 2006, 116/117: 366-369.
[15] GABATHULER J P, BUXMANN K. Process for producing a liquid-solid metal alloy phase for further processing as material in the thixotropic state. US 5186236 [P]. 1993.
[16] JIAN X, XU H, Meek T T, HAN Q. Effect of power ultrasound on solidification of aluminum A356 alloy [J]. Materials Letters, 2005, 59: 190-193.
[17] ZAWILSKI K T, CLAUDIA M, CUSTODIO C, DEMATTEI R C, FEIGELSON R S. Vibroconvective mixing applied to vertical Bridgman growth [J]. Journal of Crystal Growth, 2003, 258(1): 211- 222.
[18] OHNO A. An introduction to the solidification of metals [M]. Tokyo: Chijinshokan Press, 2003. (in Japanese)
Foundation item: Project(50775086) supported by the National Natural Science Foundation of China; Project (2007AA03Z557) supported by the Hi-tech Research and Development Program of China
Corresponding author: ZHAO Jun-wen; Tel/Fax: +86-27-87556262; E-mail: zhaojunwen1982@yahoo.com.cn
(Edited by YUAN Sai-qian)