
Comparison of LiNi0.5Mn1.5O4 cathode materials prepared by different coprecipitation methods
SUN Qiang(孙 强), WANG Zhi-xing(王志兴), LI Xin-hai(李新海),
GUO Hua-jun(郭华军), PENG Wen-jie(彭文杰)
School of Metallurgical Science and Engineering, Central South University, Changsha 410083, China
Received 15 July 2007; accepted 10 September 2007
Abstract: LiNi0.5Mn1.5O4 was synthesized by two different coprecipitation methods: composite carbonate process and composite hydroxide method. The effects of calcination temperature of precursors on the physical properties and electrochemical performance of the samples were investigated. The results of scanning electron microscopy(SEM) show that as calcination temperature increases, the crystallinity of the samples is improved, and their grain sizes are obviously increased. X-Ray diffraction(XRD) data show that the LiNi0.5Mn1.5O4 compounds obtained by two coprecipitation methods both exhibit a pure cubic spinel structure without any impurities. Furthermore, it is found that the samples prepared with relatively high temperature precursors present large initial discharge capacity (>125 mA?h/g) and excellent cycling stability with a capacity retention rate larger than 91% after 30 cycles at current density of 1 C. This probably derives from their higher crystallinity and larger grain sizes. However, the initial discharge capacity of LiNi0.5Mn1.5O4 synthesized by composite carbonate process is smaller than that prepared by composite carbonate process, but it shows better capacity retention ability.
Key words: lithium ion battery; LiNi0.5Mn1.5O4; coprecipitation method; precursor; spinel
1 Introduction
Since energy density is related to cell voltage and capacity, both high voltage and large capacity of positive materials are necessary[1-2]. Recently, some research groups have reported that transition metal-substituted spinel materials (LiMxMn2-xO4, M represents Cr, Co, Fe, Ni, Cu) showed high voltage plateau at around 5 V[3-7]. The capacity and voltage plateau in Li/LiMxMn2-xO4 cells strongly depend on the kind of transition metals (M) and their content. Among those materials, LiNi0.5Mn1.5O4 received great attentions for its dominant potential plateau at 4 V and capacity plateau at 5 V. Moreover, LiNi0.5Mn1.5O4 showed the highest discharge capacity (146.7 mA?h/g) with stable cycleability at the higher potential.
In recent years, many methods to synthesize LiNi0.5Mn1.5O4 have been reported, of which include solid-state method[8], sol-gel method[9], co-precipitation method[10], composite carbonate process[11], molten
salt method[12], emulsion drying method[13], ultrasonic spray pyrolysis method[14], and so on. Different synthesizing methods can result in products with different properties. In synthesizing LiNi0.5Mn1.5O4, secondary phases such as NiO and LixNi1-xO usually exist in the products, which can deteriorate their electrochemical behaviors[15].
In the present work, we synthesize and compare the structure and electrochemical properties of the LiNi0.5Mn1.5O4 employing two chemical wet methods: composite carbonate method and composite hydroxide method. In these two processes, NaHCO3 or NaOH is respectively used to precipitate Ni2+ and Mn2+ to obtain homogeneous sediment, which is calcined into oxide and then mixed with a stoichiometric amount of lithium to prepare LiNi0.5Mn1.5O4. It is found the obtained materials by two different methods both show high capacity and excellent cycling performance, proving clearly that the appropriate synthesizing conditions, such as calcination temperature and calcination time, have been employed.
2 Experimental
To compare the effect of synthesis method, two LiNi0.5Mn1.5O4 powders were synthesized by the composite carbonate process (termed method A) and composite hydroxide method (termed method B). The difference of these two methods lies in that the precipitation agent used in forming sediment, i.e. NaHCO3 was used in method A; NaOH was employed in method B. In both methods, NiCl2·6H2O and MnCl2·4H2O were used as the starting materials. At first, NiCl2·6H2O and MnCl2·4H2O (with cationic ratio of Ni?Mn=1?3) were dissolved in distilled water respectively, and then these solutions were mixed with different precipitation agent (NaHCO3 or NaOH) under stirring, with a subsequent aging period of 2 h, finally sediment containing Ni and Mn was obtained. The sediment was filtered via suction filtration, washed three times with distilled water and dried at 120 ℃ for 10 h. Then the sediment was calcined at 700, 800 and 900 ℃ for 10 h in air atmosphere to form a precursor containing Ni and Mn. After mixed with stoichiometric of Li2CO3, the precursor was calcined at 900 ℃ for 10 h, then directly cooled down to 600 ℃ for 24 h in air atmosphere to obtain products.
The thermogravimetric(TG) analysis special for the precursor was performed on a TA instrument (Universal V4.0C TA Instruments: SDT Q600 V8.0 Build 95) at a heating rate of 10 ℃/min in a constant flow of extra dry air at 100 mL/min. The powder X-ray diffraction(XRD, Rint-2000, Rigaku) measurement using Cu Kα radiation was employed to identify the crystalline phase of the synthesized materials, recorded at room temperature. The particle size and morphology of the LiNi0.5Mn1.5O4 powders was observed by scanning electron microscope (JEOL, JSM-5600LV) with an accelerating voltage of 20 kV.
The electrochemical characterizations have been performed using CR2025 coin-type cell. For cathode fabrication, the prepared powders were mixed with 10% (mass fraction) of acetylene black and 10% (mass fraction) of polyvinylidene fluoride in N-methyl pyrrolidinone(NMP) until a slurry was obtained. And then, the blended slurries were pasted onto an aluminum current collector, and the electrode was dried at 80 ℃ for 20 h in vacuum. The test cell consisted of the cathode fabricated and lithium foil anode separating by a porous polypropylene film, and 1 mol/L LiPF6 in EC?EMC?DMC i.e. C3H4O3?C4H8O3?C3H6O3 (1?1?1, in Volume) as the electrolyte. The assembly of the cells was carried out in a dry Ar-filled glove box. The cells were charged and discharged over a voltage range of 3.5-4.95 V versus Li/Li+ electrode at room temperature.
3 Results and discussion
Fig.1 shows the thermogravimetric(TG) curves obtained over the temperature range of 25-950 ℃, for the different sediments prepared by composite carbonate method and composite hydroxide method. The TG curve for the carbonate mixture of nickel and manganese (Ni-Mn) is shown as A in Fig.1. When temperature reached 700 ℃, the mass loss of carbonate mixture of Ni-Mn is 37%, which is higher than the mass loss of hydroxide mixture of Ni-Mn shown as Fig.1 B, only 12%. The mass losses below 700 ℃ are associated with the water release and pyrolysis of the carbonate mixture or hydroxide mixture of Ni-Mn. When the carbonate mixture of Ni-Mn decomposed, it would release CO2, but hydroxide mixture of Ni-Mn would release H2O. That is the reason why the curve of Fig.1 A shown a higher mass loss than the curve of Fig.1 B. However, when the temperature reached 700 ℃, there are only some small losses observed in both curves, which are likely caused by oxygen loss originating in the decomposition of Ni-Mn oxide[2]. To investigate the effects of calcination temperature of precursors on the physical properties and electrochemical performance of the LiNi0.5Mn1.5O4, based on the TG data, we initially used the calcining temperature of 700, 800 and 900 ℃ to prepare the Ni-Mn precursors in both methods.
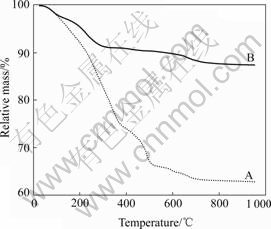
Fig.1 TG curves for carbonate mixture of Ni-Mn (A) and hydroxide mixture of Ni-Mn (B)
The precursors prepared at various temperatures mixed with stoichiometric of Li2CO3, then calcined at 900 ℃ for 10 h to obtain LiNi0.5Mn1.5O4 samples. Fig.2 shows the XRD patterns of the products prepared by methods A, B mentioned above. All the materials prepared by both methods are confirmed as a cubic spinel structure with a space group of Fd3m. As the calcination temperature of the precursors increases from 700 to 900 ℃, the diffraction peaks become sharper and stronger.
Fig.3 shows the SEM images of the LiNi0.5Mn1.5O4 prepared with precursors calcined at 700, 800 and 900 ℃
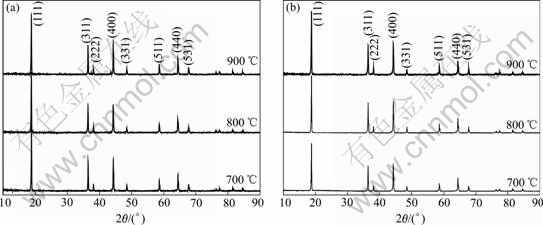
Fig.2 XRD patterns of LiNi0.5Mn1.5O4 prepared with different precursors calcined at 700, 800 and 900 ℃ respectively by composite carbonate method A (a) and composite hydroxide method B (b)
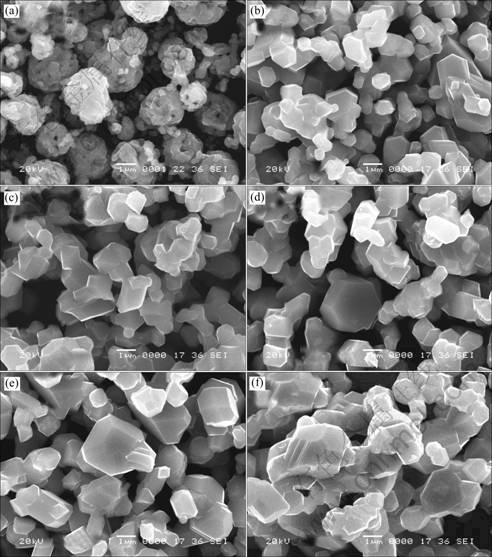
Fig.3 SEM images of LiNi0.5Mn1.5O4 prepared with precursors calcined at 700, 800 and 900 ℃ by method A, B (Among others, the calcining temperatures for (a), (b) are 700 ℃; for (c), (d) 800 ℃; for (e), (f) 900 ℃)
by composite carbonate method and composite hydroxide method. When preparing with the 700 ℃ precursor by composite carbonate method, the product consists of some spherical morphologies particles (2-5 μm) which composing many of small particles (<0.4 μm). As calcination temperature increases to 800 ℃ and 900 ℃, spherical particles are broken up and small grains grow up to 0.8-2 μm. While the product prepared with the 700℃ precursors by composite hydroxide method, it is just composed of 0.5-1 μm particles showing a little agglomeration, but the particles also grow up when the calcination temperature increases together with an homogenous distribution (0.6-5 μm). When samples are prepared with the higher temperatures (800 ℃ and 900℃) precursors by both methods, the clearer octahedral shaped characteristics of cubic spinel are observed, indicating well-developed crystallinity. These are in the line with the results from XRD.
The charge/discharge curves of Li/LiNi0.5Mn1.5O4 cells obtained using different synthetic methods are shown in Fig.4, which carried out at different current density. When the LiNi0.5Mn1.5O4 samples were prepared
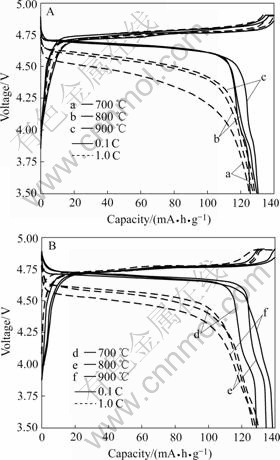
Fig.4 Charge/discharge curves of Li/LiNi0.5Mn1.5O4 prepared at 700, 800 and 900 ℃ by using composite carbonate method A and composite hydroxide method B
by both composite carbonate process and composite hydroxide method, as the precursors calcined at higher temperatures, the higher initial discharge capacity can be obtained. This may originate from relatively higher crystallinity and less electrolyte decomposition products formed in the surfaces of these samples prepared at higher temperature. It can be seen that both the plateau voltage and the discharge capacity decrease with the current density increasing, but there are some differences existed in the discharge curves of high current density (1 C). When employing composite hydroxide method, the discharge capacity of LiNi0.5Mn1.5O4 prepared with 900 ℃ precursor is lower than that of 700 ℃ and 800 ℃ at the current density of 1 C. While a composite carbonate method was adopted, the discharge capacity of the products still increased with the rising of preparing temperatures of precursors at 1 C. Compared with composite carbonate process (125-130 mA?h/g), though the LiNi0.5Mn1.5O4 products prepared by composite hydroxide method have a larger initial discharge capacity (129-138 mA?h/g), they also display a much greater decline.
Fig.5 shows the variation in the specific discharge capacity after 30 cycles for the LiNi0.5Mn1.5O4 materials prepared by different methods. The cell was charged using a current density of 14 mA/g (0.1 C rate) before each discharge test. In both the composite carbonate process and composite hydroxide method, the discharge capacity slowly decreases with increasing current density, but the sample prepared by later methods displays a greater decline than that of the first method. When the composite carbonate process was employed, the samples prepared with precursors calcined at relatively higher temperatures (800-900℃) show an excellent cycling performance at the current density of both 0.1 C and 1 C, and their capacity retention rates after 30 cycles are larger than 97%. However, the product prepared by composite hydroxide method has a larger initial discharge capacity than that of composite carbonate process, it also shows a very stable cycling behavior and the cycling retention rate after 30 cycles is over 97% at low current density of 0.1 C, but when the current density increased to 1 C, it shows aninferior cycling behavior, the cycling retention rates of products prepared with 700, 800 and 900 ℃ precursors after 30 cycles is 91%, 92% and 91%, respectively. It is reported that the dissolution of transition metals (Mn and Ni) suffering from unavoidable HF in electrolyte and the formation of LiF and carbonaceous species in the surfaces of cathode materials due to electrolyte decomposition are mainly responsible for the capacity loss of electrode materials during electrochemical cycling[10]. Moreover, it is also found that the higher crystallinity of the samples also
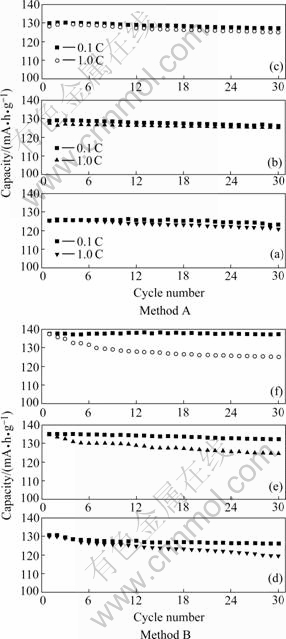
Fig.5 Cycle performances of LiNi0.5Mn1.5O4 prepared with 700, 800 and 900 ℃ precursors at charge current density of 0.1 C and 1 C using method A and method B, respectively
has essential contributions to their larger capacity and structural stability during cycling. Hence, it can be concluded that higher crystallinity and less electrolyte decomposition amount resulting from smaller reactive area are mainly responsible for the excellent cycling performance of the LiNi0.5Mn1.5O4 samples prepared with the precursors calcined at higher temperatures (800-900 ℃). So the crystallinity of the products prepared with the precursors by composite carbonate process is better than that of composite hydroxide method.
4 Conclusions
1) The composite carbonate method and composite hydroxide method of synthesizing the spinel compounds LiNi0.5Mn1.5O4 are compared in this work. The effects of calcination temperature of different precursors on the physical properties and electrochemical performance of the samples have been investigated.
2) The products prepared by the above methods can be classified as the cubic spinel Fd3m structure with simple phase. The electrochemical performance measure- ments demonstrate that LiNi0.5Mn1.5O4 powders prepared by composite carbonate process have a smaller initial discharge capacity, but have much excellent rate capability both at high current density of 1 C in comparison with those prepared by composite hydroxide process.
3) The higher temperature of preparing precursors, the better crystallinity of LiNi0.5Mn1.5O4 products can be obtained; LiNi0.5Mn1.5O4 prepared by the composite hydroxide method has inferior cycling behavior, which may results from its a little worse crystallinity.
References
[1] KAWAI H, NAGETA M, KAGEYAMA H, TUKAMOTO H, WEST A R. 5 V lithium cathodes based on spinel solid solutions Li2Co1+xMn3-xO8: -1≤x≤1 [J]. Electrochimica Acta, 1999, 45: 315-327.
[2] LIU Li-ying, TIAN Yan-wen, ZHAI Yu-chun, XU Cha-qing. Influence of Y3+ doping on structure and electrochemical performance of layered Li1.05V3O8 [J]. Trans Nonferrous Met Soc China, 2007, 17(1): 110-115.
[3] MOLENDA J, MARZEC J, SWIERCZEK K, OJCZUK W, ZIEMNICKI M, MOLENDA M, DROZDEK M, DZIEMBAU R. The effect of 3d substitutions in the manganese sublattice on the charge transport mechanism and electrochemical properties of manganese spinel [J]. Solid State Ionics, 2004, 171: 215-227.
[4] OHZUKU T, TAKEDA S, IWANAGA M. Solid-state redox potentials for Li[Me1/2Mn3/2]O4 (Me: 3d-transition metal) having spinel-framework structures: A series of 5 volt materials for advanced lithium-ion batteries [J]. Journal of Power Sources, 1999, 81/82: 90-94.
[5] OHZUKU T, ARIYOSHI K, TAKEDA S, SAKAI Y. Synthesis and characterization of 5V insertion material of Li[FeyMn2-y]O4 for lithium-ion batteries [J]. Electrochimica Acta, 2001, 46: 2327-2336.
[6] BONINO F, PANERO S, SATOLLI D, SCROSATI B. Synthesis and characterization of Li2MxMn4-xO8 (M=Co, Fe) as positive active materials for lithium-ion cells [J]. Journal of Power Sources, 2001, 97/98: 389-392.
[7] TERADA Y, YASAKA K, NISHIKAWA F, KONISHI T, YOSHIO M, NAKAI I. In situ XAFS analysis of Li(Mn, M)2O4 (M=Cr, Co, Ni) 5V cathode materials for lithium-ion secondary batteries [J]. Journal of Solid State Chemistry, 2001, 156: 286-291.
[8] FANG Hai-sheng, WANG Zhi-xing, LI Xin-hai, GUO Hua-jun, PENG Wen-jie. Exploration of high capacity LiNi0.5Mn1.5O4 synthesized by solid-state reaction [J]. Journal of Power Sources, 2006, 153: 174-176.
[9] AMDOUNI N, ZAGHIB K, GENDRON F, MAUGER A, JULIEN C M. Magnetic properties of LiNi0.5Mn1.5O4 spinels prepared by wet chemical methods [J]. Journal of Magnetism and Magnetic Materials, 2007, 309:100-105.
[10] FAN Yu-kai, WANG Jian-ming, YE Xue-bo, ZHANG Jian-qing. Physical properties and electrochemical performance of LiNi0.5Mn1.5O4 cathode material prepared by a coprecipitation method [J]. Materials Chemistry and Physics, 2007, 103: 19-23.
[11] LIU G Q, WANG Y J, QI L, LI W, CHEN H. Synthesis and electrochemical performance of LiNi0.5Mn1.5O4 spinel compound [J]. Electrochimica Acta, 2005, 50: 1965-1968.
[12] KIM J H, MYUNG S T, SUN Y K. Molten salt synthesis of LiNi0.5Mn1.5O4 spinel for 5V class cathode material of Li-ion secondary battery [J]. Electrochimica Acta, 2004, 49: 219-227.
[13] MYUNG S T, KOMABA S, KUMAGAI N, YASHIRO H, CHUNG H T, CHO T H. Nano-crystalline LiNi0.5Mn1.5O4 synthesized by emulsion drying method [J]. Electrochimica Acta, 2002, 47: 2543-2549.
[14] PARK S H, SUN Y K. Synthesis and electrochemical properties of 5V spinel LiNi0.5Mn1.5O4 cathode materials prepared by ultrasonic spray pyrolysis method [J]. Electrochimica Acta, 2004, 50: 431-434.
[15] ALCANTARA R, JARABA M, LAVELA P, TIRADA J L. Optimizing preparation conditions for 5V electrode performance, and structural changes in Li1-xNi0.5Mn1.5O4 spinel [J]. Electrochimica Acta, 2002, 47: 1829-1835.
(Edited by LAI Hai-hui)
Foundation item: Project(2007CB613607) supported by the National Basic Research Program of China
Corresponding author: WANG Zhi-xing; Tel: +86-731-8836633; E-mail: zxwang@mail.csu.edu.cn