Trans. Nonferrous Met. Soc. China 26(2016) 2960-2965
Catalytic reduction of SO2 by CO over CeO2-TiO2 mixed oxides
Li ZHANG, Yi-hong QIN, Bai-zhen CHEN, Ya-guang PENG, Han-bing HE, Yi YUAN
School of Metallurgy and Environment, Central South University, Changsha 410083, China
Received 11 November 2015; accepted 18 May 2016
Abstract: The structure and catalytic desulfurization characteristics of CeO2-TiO2 mixed oxides were investigated by means of X-ray diffraction (XRD), X-ray photoelectron spectroscopy (XPS) and catalytic activity tests. According to the results, a CeO2-TiO2 solid solution is formed when the mole ratio of cerium to titanium n(Ce):n(Ti) is 5:5 or greater, and the most suitable n(Ce):n(Ti) is determined as 7:3, over which the conversion rate of SO2 and the yield of sulfur at 500 °C reach 93% and 99%, respectively. According to the activity testing curve, Ce0.7Ti0.3O2 (n(Ce):n(Ti)=7:3) without any pretreatment can be gradually activated by reagent gas after about 10 min, and reaches a steady activation status 60 min later. The XPS results of Ce0.7Ti0.3O2 after different time of SO2+CO reaction show that CeO2 is the active component that offers the redox couple Ce4+/Ce3+ and the labile oxygen vacancies, and TiO2 only functions as a catalyst structure stabilizer during the catalytic reaction process. After 48 h of catalytic reaction at 500 °C, Ce0.7Ti0.3O2 still maintains a stable structure without being vulcanized, demonstrating its good anti-sulfur poisoning performance.
Key words: CeO2-TiO2 mixed oxides; solid solution; catalytic reduction; carbon monoxide; sulfur dioxide
1 Introduction
Sulfur dioxide (SO2), nitric oxide (NO) and carbon monoxide (CO) as by-products of combustion processes from industry, transportation and domestic activities are major components of atmospheric pollution. With the increasing environmental awareness, more and more researchers are committed to the development of efficient flue gas treatment technology. However, there is currently no widely accepted technology for the simultaneous treatment of NO, SO2 and CO.
The catalytic reduction of SO2 and NO to valuable sulfur and harmless N2 by CO has been receiving much attention. ZHUANG et al [1,2] studied the reaction of NO-SO2-CO on γ-alumina-supported sulfides of transition metals including CoMo and FeMo. They observed that stoichiometric catalytic reduction of NO and SO2 to N2 and elemental sulfur was achieved at 400 °C on the sulfided CoMo/Al2O3 and the sulfided FeMo/Al2O3. But both of them need sulfurization pretreatment before reaching the active phase. The resulting oxidized CoMo/Al2O3 regained its activity by in situ sulfurization with elemental sulfur produced by the reaction of SO2 and carbonyl sulfide (COS). In contrast, the sulfided FeMo/Al2O3 was easily oxidized by NO but hardly re-sulfided under the test conditions. ZHANG et al [3] investigated the simultaneous catalytic reduction of SO2 and NO by CO over TiO2-promoted cobalt sulfides, and found that 71% NO conversion and 84% SO2 conversion were achieved at 250 °C. The conversion rates of both NO and SO2 were improved with the increase of temperature. However, much COS formed at higher temperature, resulting in a decrease of SO2 selectivity to sulfur. ZHANG et al [4,5] had also studied the simultaneous catalytic reduction of SO2 and NO by CO over titanium-tin solid solution catalysts. Experimental results showed that the conversion rates of SO2 and NO at a temperature above 350 °C were greater than 91% and 99%, respectively. In the TiO2-SnO2 solid solutions, the stability of Sn was poorer than that of Ti, and Sn could be sulfided during the reaction process. HU et al [6] reviewed the efficient conversion of SO2 and NO on rare earth mixed compounds at 600 °C, but the conversion of SO2/NO decreases greatly with the decrease of reaction temperature.
As well known, cerium is the most abundant element in rare earth family [7], and cerium oxide (CeO2) is one of the most reactive rare earth metal oxides [8]. Due to its ability to store/release oxygen as an oxygen reservoir via the redox shift between Ce4+ and Ce3+ under oxidizing and reducing conditions, CeO2 has attracted much attention in the field of catalysis [9-11]. However, being poorly thermostable, pure CeO2 will undergo rapid sintering at high temperatures, thus greatly decreasing its oxygen storage capacity. A common measure to overcome this problem is to introduce other metal ions into the ceria cubic structure, which can increase the temperature stability and oxygen storage capacity (OSC) of CeO2.
In addition, CeO2 can form solid solutions with ZrO2, MnO2, TiO2, SiO2 and PbO2 [12]. Among them, TiO2 has attracted strong attention due to its outstanding mechanical, thermal, electrical and photocatalytic properties [13-16]. The CeO2-TiO2 nanopowders have been used in not only the photocatalyst field [17-19], but also other catalytic applications [20-26].
However, there are few references about the application of CeO2-TiO2 mixed oxides in the simultaneous catalytic reduction of SO2 and NO by CO. Compared with NO, SO2 is usually more difficult to reduce, yet it is easy to cause the poisoning of catalyst. Therefore, the catalytic desulfurization activity of CeO2-TiO2 mixed oxides was studied.
2 Experimental
2.1 Preparation of catalyst
The catalyst was prepared via sol-gel method [27]. In a typical procedure, a certain amount of cerium nitrate (Ce(NO3)2·6H2O) solid was dissolved in 20 mL of anhydrous ethanol, then stoichiometric butyl titanate (Ti(OC4H9)4) was dropped into alcohol solution under stirring to form a mixed solution with a total cation concentration of 0.02 mol/L. In the following process, acetic acid (CH3COOH) was added to adjust the pH to 0.8 and 5 mL of deionized water was added to support a hydrolysis condition. After 30 min of reaction, the sol was aged at 40 °C to form gel. The obtained wet gel was dried at 105 °C for 12 h, and finally roasted in subsection at 600 °C for 4 h to obtain the TiO2-CeO2 mixed oxides catalyst.
2.2 Measurement of catalytic activity
A packed-bed reactor made of quartz (10 mm in inner diameter and 14 mm in outer diameter) was used for the activity test. 0.5 g of catalyst powder was put in the middle of the reactor. The reactor was laid in a tube experimental electrical furnace of which the temperature could be controlled automatically, and the temperature difference of the catalyst bed was controlled within the range of ±2 °C. The activity testing temperature was fixed at 500 °C, slightly higher than the boiling point of sulfur. After the catalyst was heated in air at 500 °C for 30 min, a gas mixture of 0.3% SO2 and 0.6% CO (both in volume fraction, gas mixture supplied by Changsha Gao-Ke Gas Co. Ltd.), diluted with N2, was fed to the reactor as reactants at a constant flow rate of 200 mL/min. The inlet and outlet gases were analyzed by an on-line flue gas analyzer (MRU VARIO-plus) and the conversion rate of SO2 was calculated based on the intensity difference between the inlet SO2 and outlet SO2. The data for steady-state activity of the catalysts were collected after 2 h of testing.
2.3 Characterization of catalyst
The catalyst structure was determined by X-ray diffractometry using a Rigaku D/max 2000 XRD analyzer. Conditions of analysis were as follows: target Cu (0.15415 nm); scanning speed 10 (°)/min; scanning range (2θ) 10°-80°. In addition, the X-ray photoelectron spectra (XPS) were acquired on a VG ESCALAB5 electron spectrometer equipped with a Mg Kα radiation source (hv=123.6 eV). The main C 1s peak (Binding energy=284.6 eV), was chosen as an internal standard to calibrate the energy scale.
3 Results and discussion
3.1 XRD analysis of catalysts
Figure 1 shows the XRD patterns of CeO2-TiO2 oxide composites with different mole ratios of cerium to titanium. Pure TiO2 and CeO2 display the pure anatase phase structure (JCPDS 21-1272) and cubic fluorite phase structure (JCPDS 34-0394), respectively. All CeO2-TiO2 oxide composites with Ce to Ti mole ratio ≥5:5 exhibit the pure cubic fluorite phase structure, indicating the formation of CeO2-like solid solutions. Their diffraction peaks become broader and weaker with the increase of TiO2 content. The CeO2-TiO2 oxide composites (5:5>n(Ce):n(Ti)>1:9) are mixtures of anatase TiO2 and cubic CeO2. With the further decrease of Ce to Ti mole ratio (n(Ce)/n(Ti)≤1:9), the diffraction peaks of cubic CeO2 disappear and the CeO2-TiO2 oxide composites are all pure anatase TiO2, indicating the formation of TiO2-like solid solutions. However, the diffraction peaks of a monoclinic phase mentioned in Ref. [28] have not been found, which may be attributed to the different preparation methods and procedures.
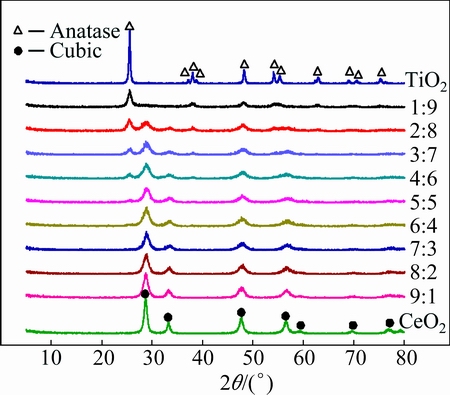
Fig. 1 XRD patterns of CeO2-TiO2 oxide composites with different Ce to Ti mole ratios
3.2 Activity of catalysts under different Ce to Ti mole ratios
CeO2-TiO2 mixed oxides with different Ce to Ti mole ratios were heated in air at 500 °C for 30 min. Then, a gas mixture of CO and SO2 with a molar ratio of 2:1 (0.6% CO, 0.3% SO2, in volume fraction) was fed into the reactor without any pretreatment. The weight-hourly- space velocity (WHSV) was fixed at 24000 mL/(h·g). The catalytic activation of CeO2-TiO2 mixed oxides with different Ce to Ti mole ratios in the CO+SO2 reaction is shown in Fig. 2. The conversion rate of SO2 over pure TiO2 is only 48%, and the value increases with the increase of Ce to Ti mole ratios. When n(Ce):n(Ti) changes from 5:5 to 8:2, all of the conversion rates of SO2 exceed 90 %, with the highest conversion rate of 93% when the n(Ce):n(Ti) ratio is equal to 7:3. After n(Ce):n(Ti) ratio reaches 9:1, the catalytic activity reduces obviously, and the catalytic activity of pure CeO2 is about 80%, lower than that of n(Ce):n(Ti) ratio between 5:5 and 8:2. The high activity of catalyst when n(Ce):n(Ti) ratio is between 5:5 and 8:2 is mainly due to the formation of a solid solution. In the solid solution, Ce4+ and Ti4+ enter into the crystal lattice of each other, forming lattice distortion and thus resulting in various defect structures. These defect structures can not only enhance the adsorption capacity of surface (adsorb more SO2), but also produce more oxygen vacancies (increase the oxygen storage capacity and the mobility of lattice oxygen). Under this condition, the catalytic activity of the mixed oxides is greatly improved. When the molar ratio of one ion to the other is too large (e.g. n(Ce):n(Ti)=9:1), some lattice defects may be associated in a certain way, leading to the healing of defects and the decrease of catalytic activity. Therefore, the most suitable molar ratio of Ti to Ce is determined as 7:3.
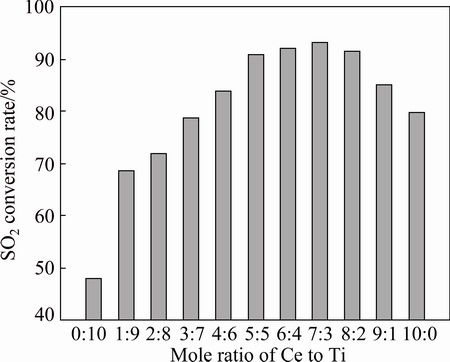
Fig. 2 Steady catalytic activity of CeO2-TiO2 mixed oxides with different Ce to Ti mole ratios in CO+SO2 reaction
3.3 Characteristics of activity testing curve
The catalytic activity test of reducing SO2 by CO over Ce0.7Ti0.3O2 was carried out at 500 °C without any pretreatment and its catalytic desulfurization curve is shown in Fig. 3. It is found that the output of SO2 in the effluent increases in the first 10 min, then decreases and stabilizes at the lowest platform after about 60 min. When the reactive gas mixture flows into the reactor, SO2 may be greatly absorbed by the catalyst in the beginning (Fig. 3(a)). With the increase of the absorbed gas on the surface of the catalyst, the adsorption ability of the catalyst on SO2 is reduced; hence, the output of SO2 gradually increases. After about 10 min, the catalyst is activated, leading to the gradual decrease of the SO2 output. Once the catalyst activation is completed (after about 60 min), the catalytic activity will reach a steady state, and the output of SO2 will be stabilized at a platform.
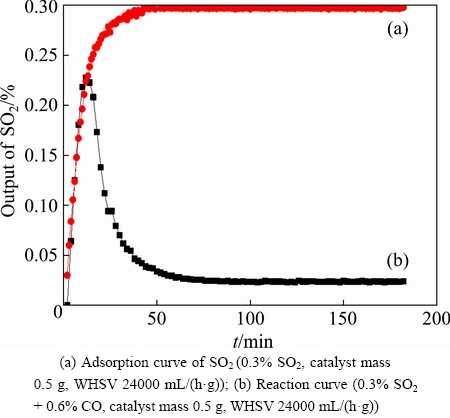
Fig. 3 Output of SO2 in effluents over Ce0.7Ti0.3O2 catalyst at 500 °C
Certain quantity of CO2 is found in the gas outlet and elemental sulfur is also observed in the receiving flask after the reaction begins for a while. When the SO2+CO reaction achieves its stability, the yield of sulfur, which is obtained by measuring the change of sulfur mass and the amount of removed SO2 within a certain period of time, reaches about 99%.
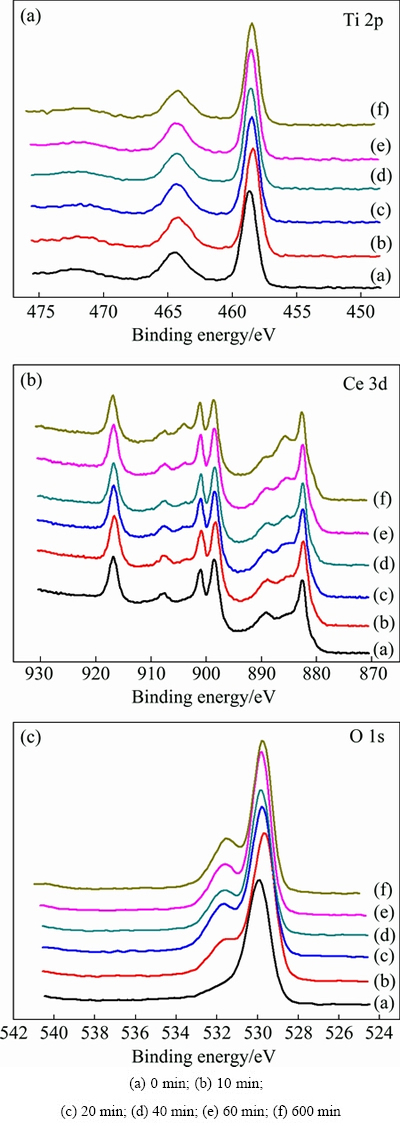
Fig. 4 XPS spectra of Ti 2p, Ce 3d and O 1s for catalyst Ce0.7Ti0.3O2 with different reaction time
3.4 Characteristics of catalytic reaction process
After different catalytic reaction time, the oxide catalysts were analyzed by XPS. The XPS spectra of Ce 3d, Ti 2p and O 1s are shown in Fig. 4. Ti 2p spectra for the catalysts with different reaction time are similar to the fresh one, which only has peaks of Ti4+ at 464.4 eV (Ti 2p1/2) and 458.7 eV (Ti 2p3/2), without other states of titanium ions [29,30], illustrating that Ti4+ is not the redox species during the reaction process and is not the active substance. On the fresh catalyst, Ce 3d spectra have peaks at 916.5 eV (Ce 3d3/2) and 882.59 eV (Ce 3d5/2), which are the values of CeO2. After about 10 min of reaction, the peaks at about 904 eV (Ce 3d3/2) and 885.6 eV (Ce 3d5/2) begin to appear in the Ce 3d XPS spectra. As the time of catalytic reaction increases, the two peaks are strengthened gradually. These changes in the Ce 3d XPS spectra indicate the increase of Ce3+ concentration on the surface of the catalyst [31,32]. In addition, the peaks of Ce4+ weaken with the increase of reaction time, suggesting that Ce4+ is reduced into Ce3+ gradually during the catalytic reaction and forms a high oxygen deficiency state. This assumption is also confirmed by O 1s spectra, which are broad and complicated because of the nonequivalence of surface oxygen ions. The Ce 3d XPS spectra can be also used to verify the variation of desulfurization curve.
3.5 Stability of catalyst
The phase compositions of Ce0.7Ti0.3O2 powder before the catalytic reaction and after 48 h of catalytic reaction at 500 °C were analyzed by XRD, and the results are shown in Fig. 5.
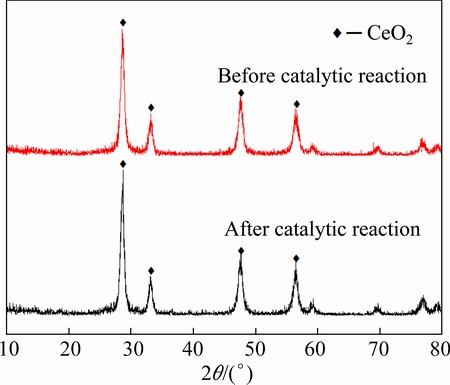
Fig. 5 XRD patterns before and after catalytic reaction
The structure of Ce0.7Ti0.3O2 before and after the catalytic reaction does not change much, and only the main diffraction peak intensity of CeO2 in cubic type has trace levels of enhancement after the catalytic reaction. This is possibly because that the Ce0.7Ti0.3O2 crystal gets further perfect during the testing process at 500 °C. Thus, it can be seen that the catalyst Ce0.7Ti0.3O2 maintains a stable structure without being vulcanized after 48 h of catalytic reaction.
The XPS survey spectra of the fresh and used (after de-SO2) Ce0.7Ti0.3O2 catalysts are shown in Fig. 6. There are mainly Ti, Ce and O on the surface of the catalyst, and C is the pollution source introduced by the test system. No obvious peak of S 2p is observed for the catalyst after desulfurization reaction. Both the XRD and XPS analysis results suggest that Ce0.7Ti0.3O2 has good anti-sulfur properties.
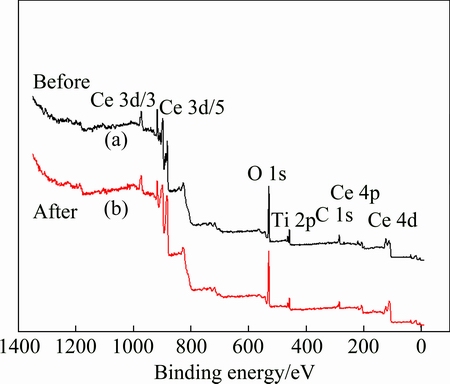
Fig. 6 XPS survey spectra of fresh (a) and used (b) Ce0.7Ti0.3O2 catalysts
4 Conclusions
1) CeO2-TiO2 solid solution is formed when the mole ratio of cerium to titanium (n(Ce)/n(Ti)) is 5:5 or greater, and the most suitable n(Ce)/n(Ti) ratio is determined as 7:3, over which the SO2 conversion rate and the stable yield of sulfur at 500 °C can reach 93% and 99%, respectively.
2) Ce0.7Ti0.3O2 without any pretreatment may effectively absorb SO2 at first, it is gradually activated after about 10 min, and reaches a steady activation status 60 min later. During the catalytic process, CeO2 is the active component that offers the redox couple Ce4+/Ce3+ and labile oxygen vacancies, and TiO2 functions as a catalyst structure stabilizer.
3) Both the XRD and XPS results show that after 48 h of catalytic reaction at 500 °C, Ce0.7Ti0.3O2 still maintains a stable structure without being vulcanized, demonstrating its good anti-sulfur poisoning performance.
References
[1] ZHUANG S X, MAGARA H, YAMAZAKI M, TAKAHASHI Y, YAMADA M. Catalytic conversion of CO, NO and SO2 on the supported sulfide catalyst: I. Catalytic reduction of SO2 by CO [J]. Applied Catalysis B: Environmental, 2000, 24(2): 89-96.
[2] ZHUANG S X, YAMAZAKI M, OMATA K, TAKAHASHI Y, YAMADA M. Catalytic conversion of CO, NO and SO2 on the supported sulfide catalyst: II. Catalytic reduction of NO and SO2 by CO [J]. Applied Catalysis B: Environmental, 2001, 31(2): 133-143.
[3] ZHANG Zhao-liang, MA Jun, YANG Xi-yao. Separate/simultaneous catalytic reduction of sulfur dioxide and/or nitric oxide by carbon monoxide over TiO2-promoted cobalt sulfides [J]. Journal of Molecular Catalysis A: Chemical, 2003, 195(1-2): 189-200.
[4] ZHANG Zhao-liang, MA Jun, YANG Xi-yao. Separate/simultaneous catalytic reduction of sulfur dioxide and/or nitric oxide by carbon monoxide over titanium-tin solid solution catalysts [J]. Chemical Engineering Journal, 2003, 95(1): 15-24.
[5] ZHANG Zhao-liang, GENG Hao-ran, ZHENG Li-sheng, DU Bin. Resistance to sulfidation and catalytic performance of titanium-tin solid solutions in SO2+CO and NO+SO2+CO reactions [J]. Applied Catalysis A: General, 2005, 284(1-2): 231-237.
[6] HU Hui, WANG Shu-xia, ZHANG Xiao-ling, ZHAO Quan-zhong, LI Jin. Study on simultaneous catalytic reduction of sulfur dioxide and nitric oxide on rare earth mixed compounds [J]. Journal of Rare Earths, 2006, 24(6): 695-698.
[7] ZHU Yi, ZHANG Sheng-yi, SONG Ji-ming, MAO Chang-jie, NIU He-lin, JIN Bao-kang, TIAN Yu-peng. Synthesis and electrochemiluminescence of the CeO2/TiO2 composite [J]. Electrochimica Acta, 2011, 56(22): 7550-7554.
[8] FANG Jun, BI Xin-zhen, SI De-jun, JIANG Zhi-quan, HUANG Wei-xin. Spectroscopic studies of interfacial structures of CeO2-TiO2 mixed oxides [J]. Applied Surface Science, 2007, 253(22): 8952-8961.
[9] LOPEZ T, ROJAS F, ALEXZNDER-KATZ R, GALINDO F, BALANKIN A, BULJAN A. Porosity, structural and fractal study of sol-gel TiO2-CeO2 mixed oxides [J]. Journal of Solid State Chemistry, 2004, 177(6): 1873-1885.
[10] SANGEETHA P, CHEN Yu-wen. Preferential oxidation of CO in H2 stream on Au/CeO2-TiO2 catalysts [J]. International Journal of Hydrogen Energy, 2009, 34(17): 7342-7347.
[11] LU Xiao-wang, QIAN Jun-chao, CHEN Feng, LI Xia-zhang, CHEN Zhi-gang. Synthesis, characterization and antibacterial property of Ag/mesoporous CeO2 nanocomposite material [J]. Transactions of Nonferrous Metals Society of China, 2012, 22: 1418-1422.
[12] ZHU Zhi-hui, HE De-hua. CO hydrogenation to iso-C4 hydrocarbons over CeO2-TiO2 catalysts [J]. Fuel, 2008, 87(10-11): 2229-2235.
[13] YANG Xi-jia, WANG Shu, SUN Hai-ming, WANG Xiao-bing, LIAN Jian-she. Preparation and photocatalytic performance of Cu-doped TiO2 nanoparticles [J]. Transactions of Nonferrous Metals Society of China, 2015, 25: 504-509.
[14] CAO Guo-jian, CUI Bo, WANG Wen-qi, TANG Guang-ze, FENG Yi-cheng, WANG Li-ping. Fabrication and photodegradation properties of TiO2 nanotubes on porous Ti by anodization [J]. Transactions of Nonferrous Metals Society of China, 2014, 24: 2581-2587.
[15] LUO Qiang, CAI Qi-zhou, LI Xin-wei, PAN Zhen-hua, LI Yu-jie, CHEN Xi-di, YAN Qing-song. Preparation and characterization of ZrO2/TiO2 composite photocatalytic film by micro-arc oxidation [J]. Transactions of Nonferrous Metals Society of China, 2013, 23: 2945-2950.
[16] TAO Jie, DENG Jie, DONG Xiang, ZHU Hong, TAO Hai-jun. Enhanced photocatalytic properties of hierarchical nanostructured TiO2 spheres synthesized with titanium powders [J]. Transactions of Nonferrous Metals Society of China, 2012, 22: 2049-2056.
[17] HAO Chun-jing, LI Jing, ZHANG Zai-lei, JI Yong-jun, ZHAN Han-hui, XIAO Fang-xing, WANG Dan, LIU Bin, SU Fa-bing. Enhancement of photocatalytic properties of TiO2 nanoparticles doped with CeO2 and supported on SiO2 for phenol degradation [J]. Applied Surface Science, 2015, 331: 17-26.
[18] AMEEN S, AKHTAR M S, SEO H K, SHIN H S. Solution-processed CeO2/TiO2 nanocomposite as potent visible light photocatalyst for the degradation of bromophenol dye [J]. Chemical Engineering Journal, 2014, 247: 193-198.
[19] MUNOZ-BATISTA M J, FERRER M, FERNANDEZ-GARCIA M, KUBACKA A. Abatement of organics andEscherichia coliusing CeO2-TiO2 composite oxides: Ultraviolet and visible light performances [J]. Applied Catalysis B: Environmental, 2014, 154-155: 350-359.
[20] ZHANG Rui, ZHONG Qin, ZHAO Wei, YU Le-meng, QU Hong-xia. Promotional effect of fluorine on the selective catalytic reduction of NO with NH3 over CeO2-TiO2 catalyst at low temperature [J]. Applied Surface Science, 2014, 289: 237-244.
[21] ZHANG Lei, LI Lu-lu, CAO Yuan, YAO Xiao-jiang, GE Cheng-yan, GAO Fei, DENG Yu, TANG Chang-jin, DONG Lin. Getting insight into the influence of SO2 on TiO2/CeO2 for the selective catalytic reduction of NO by NH3 [J]. Applied Catalysis B: Environmental, 2015, 165: 589-598.
[22] CHAISUK C, WEHATORANAWEE A, PREAMPIYAWAT S, NETIPHAT S, SHOTIPRUK A, PANPRANOT J, JONGSOMJIT B, MEKASUWANDUMRONG O. Preparation and characterization of CeO2/TiO2 nanoparticles by flame spray pyrolysis [J]. Ceramics International, 2011, 37(5): 1459-1463.
[23] LI Hai-long, WU Chang-yu, LI Li-qing, LI Ying, ZHAO Yong-chun, ZHANG Jun-ying. Kinetic modeling of mercury oxidation by chlorine over CeO2-TiO2 catalysts [J]. Fuel, 2013, 113: 726-732.
[24] ZHAO Bin-xia, SHI Bin-chu, ZHANG Xiao-li, CAO Xin, ZHANG Yao-zhong. Catalytic wet hydrogen peroxide oxidation of H-acid in aqueous solution with TiO2-CeO2 and Fe/TiO2-CeO2 catalysts [J]. Desalination, 2011, 268(1-3): 55-59.
[25] LI Shu-na, ZHU Hua-qing, QIN Zhang-feng, WANG Guo-fu, ZHANG Ya-gang, WU Zhi-wei, LI Zhi-kai, CHEN Gang, DONG Wei-wen, WU Zhong-hua, ZHENG Li-rong, ZHANG Jing, HU Tian-dou, WANG Jian-guo. Morphologic effects of nano CeO2-TiO2 on the performance of Au/CeO2-TiO2 catalysts in low-temperature CO oxidation [J]. Applied Catalysis B: Environmental, 2014, 144: 498-506.
[26] CHEN Bu-ming, GUO Zhong-cheng, XU Rui-dong. Electrosynthesis and physicochemical properties of α-PbO2-CeO2-TiO2 composite electrodes [J]. Transactions of Nonferrous Metals Society of China, 2013, 23: 1191-1198.
[27] CHEN Chun-liang, WENG Hung-shan. Nanosized CeO2-supported metal oxide catalysts for catalytic reduction of SO2 with CO as a reducing agent [J]. Applied Catalysis B: Environmental, 2005, 55: 115-122.
[28] LUO Meng-fei, CHEN Jun, CHEN Lin-shen, LU Ji-qing, FENG Zhao-chi, LI Can. Structure and redox properties of CexTi1-xO2 solid solution [J]. Chemistry of Materials, 2000, 13(1): 197-202.
[29] DUTTA G, WAGHMARE U V, BAIDYA T, HEGDE M S, PRIOLKAR K R, SARODE P R. Origin of enhanced reducibility/ oxygen storage capacity of Ce1-xTixO2 compared to CeO2 or TiO2 [J]. Chemistry of Materials, 2006, 18(14): 3249-3256.
[30] FANG Jun, BAO Hui-zhi, HE Bo, WANG Fang, SI De-jun, JIANG Zhi-quan, PAN Zhi-yun, WEI Shi-qiang, HUANG Wei-xin. Interfacial and surface structures of CeO2-TiO2 mixed oxides [J]. The Journal of Physical Chemistry C, 2007, 111(51): 19078-19085.
[31] REDDY B M, KHAN A. Structural characterization of CeO2-TiO2 and V2O5/CeO2-TiO2 catalysts by Raman and XPS techniques [J]. The Journal of Physical Chemistry B, 2003, 107(22): 5162-5167.
[32] WATANABE S, MA Xiao-liang, SONG Chun-shan. Characterization of structural and surface properties of nanocrystalline TiO2-CeO2 mixed oxides by XRD, XPS, TPR, and TPD [J]. The Journal of Physical Chemistry C, 2009, 113(32): 14249-14257.
铈钛复合氧化物催化CO还原SO2
张 丽,秦毅红,陈白珍,彭亚光,何汉兵,袁 依
中南大学 冶金与环境学院,长沙 410083
摘 要:利用X射线衍射(XRD),X射线光电子能谱(XPS)及活性测试对铈钛复合氧化物的结构及催化脱硫特性进行了研究。结果表明:当铈-钛摩尔比(n(Ce)/n(Ti))≥5:5时,铈钛复合氧化物形成固溶体;当n(Ce)/n(Ti)=7:3(即Ce0.7Ti0.3O2)时,具有最佳的催化脱硫效果;500 °C下SO2的转化率达到93%,单质硫的产率达到99%。根据Ce0.7Ti0.3O2的活性测试曲线发现:未经预处理的Ce0.7Ti0.3O2约10 min后开始逐渐被反应气体活化,并在60 min后达到稳定活化状态。通过对不同反应时间下所得Ce0.7Ti0.3O2进行XPS分析发现: CeO2为活性物质,在反应过程中形成Ce4+/Ce3+氧化还原电对和活性氧空位,而TiO2仅起到稳定催化剂结构的作用。Ce0.7Ti0.3O2催化SO2+CO反应48 h后未出现硫化现象,始终保持结构的稳定,表现出较好的抗硫中毒性能。
关键词:铈钛复合氧化物;固溶体;催化还原;一氧化碳;二氧化硫
(Edited by Xiang-qun LI)
Corresponding author: Yi-hong QIN; Tel:+86-731-88830216 ; E-mail: qinyihong@163.com
DOI: 10.1016/S1003-6326(16)64426-6