J. Cent. South Univ. Technol. (2009) 16: 0013-0017
DOI: 10.1007/s11771-009-0002-x
1High temperature cyclic oxidation behavior of Y2O3-ZrO2 thermal barrier coatings irradiated by high-intensity pulsed ion beam
2WANG Yi-qi(王一奇)1, 2, LEI Ming-kai(雷明凯)2, AFSAR A M1, SONG J I1
(1. Department of Mechanical Engineering, Changwon National University, Changwon 641-773, Korea;
2. School of Materials Science and Engineering, Dalian University of Technology, Dalian 116024, China)
Abstract: The high-temperature oxidation resistance behavior of 7% (mass fraction) Y2O3-ZrO2 thermal barrier coatings (TBCs) irradiated by high-intensity pulsed ion beam (HIPIB) was investigated under the cyclic oxidation condition of 1 050 ℃ and 1 h. The columnar grains in the TBCs disappear after the HIPIB irradiation at ion current densities of 100-200 A/cm2 and the irradiated surface becomes smooth and densified after remelting and ablation due to the HIPIB irradiation. The thermally grown oxide (TGO) layer thickness of the irradiated TBCs is smaller than that of the original TBCs. After 15 cycles, the mass gains of the original TBCs and those irradiated by ion current densities of 100 and 200 A/cm2 due to the oxidation are found to be 0.8-0.9, 0.6-0.7, and 0.3-0.4 mg/cm2, respectively. The inward diffusion of oxygen through the irradiated TBCs is significantly impeded by the densified top layer formed due to irradiation, which is the main reason for the improved overall oxidation resistance of the irradiated TBCs.
Key words: Y2O3; ZrO2; thermal barrier coating; high-intensity pulsed ion beam; electron beam physical vapor deposition; oxidation resistance; cyclic oxidation
1 Introduction
Thermal barrier coatings (TBCs) can effectively extend the service life and enhance the safety of heated parts. Therefore, TBCs have been widely used in different heated sections of aircraft engines for the last few decades to protect the substrates from adverse effects of thermal shock [1-3], high temperature [4-7], oxidation [5, 7-9], and corrosion [8, 10].
Although the TBCs show better performance in high temperature applications, they are prone to fail if they cannot be developed properly with desired characteristics. Therefore, many researchers are still searching for suitable methods of surface modification in an attempt to improve the characteristics of TBCs. The technique of high-intensity pulsed ion beam (HIPIB) has been found to be effective and extensively used as a new approach for surface modification and coating deposition. Scientists of the United States, Russia, Japan, and other countries have made extreme progress in the field of material surface modification by HIPIB technique. They have already extended the application of HIPIB from semiconductor annealing to surface irradiation of metallic/nonmetallic materials to others and found that this technique is superior to other conventional techniques [11-16]. In particular, HIPIB irradiation can transfer the ion energy to the target in a short period of time and with high efficiency, and the physical and chemical properties of the target surface may be changed dramatically [17]. Because of these changes, the wear resistance and oxidation resistance are improved significantly [12]. The other advantage of this technique was elaborately discussed by REMNEV et al [18]. Because of the above outstanding advantages of HIPIB, this technique was used in the present study to irradiate the surface of Y2O3-ZrO2 TBCs to investigate their cyclic oxidation behavior.
2 Experimental
2.1 Preparation of TBCs using electron beam physical vapor deposition
Electron beam physical vapor deposition (EB-PVD) [6-8, 10] technique was used to prepare the TBCs with a bonding layer of NiCoCrAlY having a thickness of 40 μm and a 7% (mass fraction) Y2O3-ZrO2 ceramic layer having a thickness of 150 μm. The EB-PVD technique can control the thickness and maintain the uniformity of the coating. Furthermore, the thermal load developed in the columnar grain structure of coatings owing to the difference of linear coefficients of thermal expansion can be released under high temperature condition. Consequently, the EB-PVD enhances the thermal fatigue resistance of the coatings.
2.2 Irradiation and cyclic oxidation of TBCs
Irradiation of TBCs was carried out using the TEMP-6 type HIPIB apparatus [11-13, 16]. The samples were placed inside the vacuum chamber and irradiated with HIPIB under a vacuum of 1 kPa. The irradiation parameters are shown in Table 1.
Table 1 Irradiation parameters of HIPIB
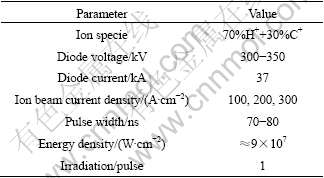
The high-temperature oxidation experiment was carried out in quiescent air of the tubular furnace, as shown in Fig.1. The cycle of the experiment consisted of placing the sample inside the tubular furnace, raising the temperature to 1 050 ℃ within 5 min, holding this temperature for 1 h, removing the sample from the furnace and cooling to the room temperature, and weighing the sample together with the quartz cuvette by a BS124S-type precision electronic balance having an accuracy of 0.1 mg.
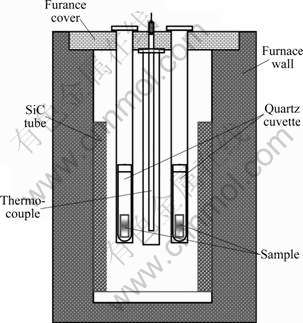
Fig.1 Schematic diagram of experimental apparatus for cyclic oxidation
2.3 Method of coating structure analysis
In order to analyze the effects of HIPIB irradiation on TBCs, the phase structures of the surface layers were examined by X-ray diffraction (XRD) method. The micrographs of the surfaces and the cross sections of the original and irradiated TBCs were analyzed by scanning electron microscopy (SEM).
3 Results and discussion
The ion current densities of 100 and 200 A/cm2 were used to irradiate the TBC surface. To ensure that the coating structure was not influenced after the irradiation by HIPIB with ion current densities of 100 and 200 A/cm2, a higher density of ion beam current (300 A/cm2) was used to irradiate the surface layer of the TBC of separate samples. The XRD patterns of these samples after irradiation are shown in Fig.2, which shows that the structure of single-phase tetragonal ZrO2 coating is not influenced by HIPIB with an ion beam current density of 300 A/cm2 after irradiation. Thus, it is obvious that the structures of TBC surface layer, irradiated by lower densities of ion beam currents (i.e. the ion current densities of 100 and 200 A/cm2), are also not influenced after irradiation.
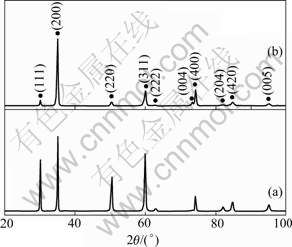
Fig.2 XRD patterns of original (a) and irradiated 7% Y2O3- ZrO2 TBC surface layers at current density of 300 A/cm2 (b)
The surface of original TBCs is full of ridgy defects that are produced by the process of EB-PVD. One ridgy defect corresponds to one ZrO2 columnar grain whose size is several microns. Although the columnar grains form dense clusters, the gaps among the grains are clear and considerably large, as shown in Fig. 3(a).
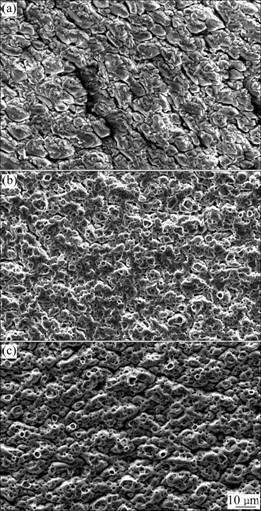
Fig.3 SEM images of EB-PVD TBCs: (a) Original; (b) Irradiated at 100 A/cm2; (c) Irradiated at 200 A/cm2
After irradiation by HIPIB, the irradiated surface is smoothed and densified in comparison with that of the original sample, as shown in Fig.3. This is achieved by the remelting and ablation of the surface as a result of irradiation by HIPIB. Because of this, the ridgy defects on the surface partially disappear. It is noted that the surface of TBCs irradiated at 200 A/cm2 has a worse roughness than that irradiated at 100 A/cm2, as shown in Figs.3(b) and (c).
Although the existing gaps among the grains of the original TBC shown in Fig.4(a) are helpful for matching the thermal expansions of the coating layers, oxygen can easily diffuse through the channels formed by the gaps to the bonding layer during high temperature application. As a result, the oxidation of the bonding layer is accelerated and thermally grown oxide (TGO) is generated in TBCs. After the irradiation at ion current densities of 100 and 200 A/cm2, the gaps among the ceramic layer columnar grains are filled to a certain extent (Figs.4(b) and (c)), while the grains are integrated together as a result of ablation and remelting.
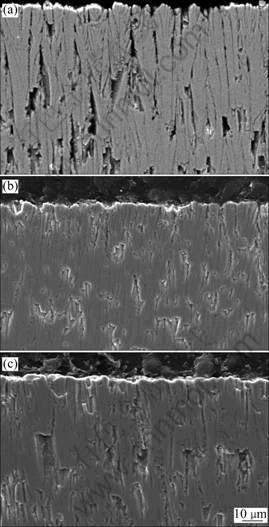
Fig.4 SEM images of x-section of EB-PVD TBCs: (a) Original; (b) Irradiated at 100 A/cm2; (c) Irradiated at 200 A/cm2
The 15 cycle oxidation kinetic curves for both the original and the irradiated TBCs approximately follow the parabolic law, as shown in Fig.5. In other words, during the first several cycles of the oxidation, the rate of mass gain of the coatings is high.
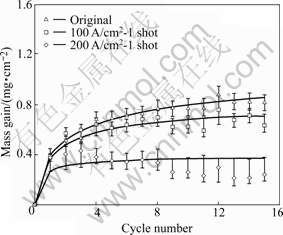
Fig.5 Oxidation kinetic curves of original and irradiated TBCs for 15 cycles
At the end of the cyclic oxidation experiment, the mass gain of the original TBCs is 0.8-0.9 mg/cm2.Compared with that of the original TBCs, the mass gain of the irradiated TBCs due to the oxidation is lower by some magnitudes, depending on the ion current intensity. After 15 cycles, the mass gain of the TBCs irradiated at 100 A/cm2 with 1 shot of HIPIB is 0.6-0.7 mg/cm2, which shows a little improvement in the oxidation behavior of the irradiated TBCs in comparison with that of the original TBCs. On the other hand, the TBCs irradiated at 200 A/cm2 with 1 shot of HIPIB show the mass gain of 0.3-0.4 mg/cm2, which indicates a remarkable improvement in the oxidation behavior of the irradiated TBCs, as shown in Fig.5.
A TGO layer is found between the ceramic and the bonding layers of TBCs after 15 cycles of oxidation at 1 050℃ for 1 h. For the original TBCs, the columnar grain structures of the ceramic layer become loose after 15 cycles, and the thickness of the TGO layer is about 2 μm. In many locations, fingerlike oxides irregularly extending towards the bonding layer are observed in Fig.6(a). However, the thickness of the TGO layers formed in the irradiated TBCs is reduced to a certain extent. The fingerlike oxide is found only in few locations of the bonding layer of TBCs irradiated at 100 A/cm2 with 1 shot, as shown in Fig.6(b). The thickness of the TGO layer of the TBCs irradiated at 200 A/cm2 with 1 shot is the minimum, and there is no fingerlike oxide in the bonding layer, as shown in Fig.6(c). The results of these observations are in good agreement with the cyclic oxidation kinetic curves shown in Fig.5. When the HIPIB current density reaches 200 A/cm2, the surface of the TBCs melts due to ablation, and the gaps among the columnar grains are filled and blocked. Therefore, the oxygen cannot diffuse towards the bonding layer and the oxidation is reduced. Consequently, the HIPIB irradiation improves the cyclic oxidation behavior of the TBCs.
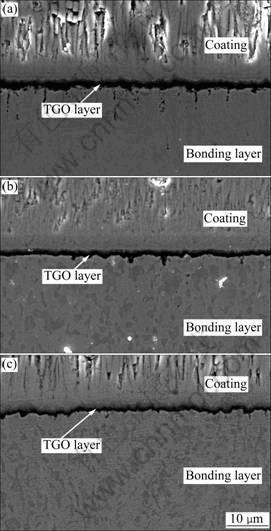
Fig.6 SEM images of TGO layer in TBCs after oxidation: (a) Original; (b) Irradiated at 100 A/cm2; (c) Irradiated at 200 A/cm2
4 Conclusions
(1) The irradiation of 7%Y2O3-ZrO2 TBCs by the HIPIB partially ablates the ridgy defects of the surface. Thus, the columnar grain characteristic disappears and a smoothed and densified structure is formed. The depth and the compactness of the remelted layer increase as the ion current density increases. However, the structures of the original and the irradiated TBCs still possess the structure of single-phase tetragonal ZrO2.
(2) After 15 cycles of oxidation at 1 050 ℃ for 1 h, the mass gain of the original TBCs is 0.8-0.9 mg/cm2. The mass gain of the TBCs irradiated at an ion current density of 100 A/cm2 with 1 shot is about 0.6-0.7 mg/cm2, which is a bit lower than that of the original TBCs. The TBCs irradiated at an ion current density of 200 A/cm2 with 1 shot exhibits a remarkable improvement in the oxidation behavior with a mass gain of only 0.3-0.4 mg/cm2.
(3) The underlying reason behind the improvement in the oxidation behavior of 7%Y2O3-ZrO2 TBCs is the remelted and densified layers formed by the HIPIB irradiation. This protects the oxygen diffusion towards the bonding layer and thus reduces the oxidation rate of the bonding layer.
References
[1] HAMACHA R, DIONNET B, GRIMAUD A, NARDOU F. Residual stress evolution during the thermal cycling of plasma-sprayed zirconia coatings [J]. Surface and Coatings Technology, 1996, 80(3): 295-302.
[2] LU An-xian, CHANG Ying, CAI Xiao-mei. Investigation on plasma-sprayed ZrO2 thermal barrier coating on nickel alloy substrate [J]. Journal of Central South University of Technology, 2002, 9(4): 225-234.
[3] TAYMAZ I. The effect of thermal barrier coatings on diesel engine performance [J]. Surface and Coatings Technology, 2007, 201(9/11): 5249-5252.
[4] BANSAL N P, ZHU D M. Thermal properties of oxides with magnetoplumbite structure for advances thermal barrier coatings [J]. Surface and Coatings Technology, 2008, 202(12): 2698-2703.
[5] SAREMI M, AFRASIABI A, KOBAYASHI A. Microstructural analysis of YSZ and YSZ/Al2O3 plasma sprayed thermal barrier coatings after high temperature oxidation [J]. Surface and Coatings Technology, 2008, 202(14): 3233-3238.
[6] OCHANDO M I, VILA M, PRIETO C. Optical and structural study of EB-PVD ZrO2 thin films [J]. Vacuum, 2007, 81(11/12): 1484-1488.
[7] LI He-fei, GUO Hong-bo, GONG Sheng-kai. Failure mechanism of EB-PVD thermal barrier coatings on NiAl substrate [J]. Trans Nonferrous Met Soc China, 2007, 17(4): 811-815.
[8] BARTSCH M, BAUFELD B, DALKILIC S, CHERNOVA L, HEINZELMANN M. Fatigue cracks in a thermal barrier coating system on a superalloy in multiaxial thermomechanical testing [J]. International Journal of Fatigue, 2008, 30(2): 211-218.
[9] PARK J H, KIM J S, LEE K H. Effects of the laser treatment and thermal oxidation behavior of CoNiCrAlY/ZrO2-8wt%Y2O3 [J]. Journal of Materials Processing Technology, 2008, 201(1/3): 331-335.
[10] MU Ren-de, SONG Xi-wen, TAO Chun-hu, HE Li-min. Measurement of thermal diffusivity of EB-PVD thermal barrier coatings and influence factors [J]. Journal of Central South University: Science and Technology, 2008, 39(2): 256-261. (in Chinese)
[11] ZHU Xiao-peng, LEI Ming-kai, MA Teng-cai. Surface morphology of titanium irradiated by high-intensity pulsed ion beam [J]. Nuclear Instruments and Methods in Physics Research B, 2003, 211(1): 69-79.
[12] WANG Xu, HAN Xiao-guang, LEI Ming-kai, ZHANG Jun-shan. Effect of high-intensity pulsed ion beams irradiation on corrosion resistance of 316L stainless steel [J]. Materials Science and Engineering A, 2007, A457(1/2): 84-89.
[13] ZHU Xiao-peng, XU Zhi-cheng, MIAO Shou-mou, LEI Ming-kai. Magnetically insulation of the ion diode used for high-intensity pulsed ion beam [J]. Surface and Coatings Technology, 2007, 201(9/11): 5264-5268.
[14] REJ D J, DAVIS H A, NASTASI M, OLSON J C, PETERSON E J, REISWIG R D, WALTER K C, STINNETT R W, REMNEV G E, STRUTS V K. Surface modification of AISI-4620 steel with intense pulsed ion beams [J]. Nuclear Instruments and Methods in Physics Research B, 1997, 127/128: 987-991.
[15] DAVIS H A, WOOD B P, MUNSON C P, BITTEKER L J, NASTASI M A, REJ D J, WAGANAAR W J, WALTER K C, COATES D M, SCHLEINITZ H M. Ion beam and plasma technology development for surface modification at Los Alamos National Laboratory [J]. Materials Chemistry and Physics, 1998, 54(1/3): 213-218.
[16] ZHU X P, SUEMATSU H, JIANG W H, YATSUI K. Structures and photoluminescence properties of silicon thin films prepared by pulsed ion-beam evaporation [J]. Materials Science and Engineering B, 2008, B149(1): 105-110.
[17] WU Di, LIU Chen, ZHU Xiao-peng, LEI Ming-kai. Numerical study on modification of ceramic coatings by high-intensity pulsed ion beam [J]. Vacuum, 2009, 83(1/4): 198-200.
[18] REMNEV G E, ISAKOV I F, OPEKOUNOV M S, MATVIENKO V M, RYZHKOV V A, STRUTS V K, GRUSHIN I I, ZAKOUTAYEV A N, POTYOMKIN A V, TARBOKOV V A, PUSHKARYOV A N, KUTUZOV V L, OVSYANNIKOV M Y. High intensity pulsed ion beam sources and their industrial applications [J]. Surface and Coatings Technology, 1999, 114(2/3): 206-212.
Foundation item: Projects supported by The 2nd Stage of Brain Korea and Korea Research Foundation
Received date: 2008-07-25; Accepted date: 2008-09-03
Corresponding author: SONG J I, Professor, PhD; Tel: +86-55-2133606; E-mail: jisong@changwon.ac.kr
(Edited by CHEN Wei-ping)