
Structure and electrochemical properties of LiMn2O4
YU Ze-min(俞泽民), ZHAO Lian-cheng(赵连城)
School of Materials Science and Engineering, Harbin Institute of Technology, Harbin 150001, China
Received 26 March 2007; accepted 6 June 2007
Abstract: LiMn2O4, a cathode material of lithium ion battery, was prepared by the citric acid complexing method using lithium acetate and manganese acetate as raw materials. The type of atom location confused degree, the confused degree and judgement method in LiMn2O4 were analyzed. The effect of sintering temperature on structure and electrochemical properties of LiMn2O4 was also investigated. The results show that the atom location confused degree increases with the decrease of the X-ray diffraction peak intensity ratio of LiMn2O4, I111/I311. The type of atom location confused degree depends on the variation tendency of I111/I311 and I311/I400 value. If the variation tendency is the same, it belongs to the 16c type location confusion, however, if the variation tendency is contrary, it belongs to the anti-spinel type location confusion. When the sintering temperature is low, it is apt to produce the anti-spinel location confusion in LiMn2O4. With the increase of sintering temperature, the confused degree with the anti-spinel type gradually reduces, however, the confused degree with 16c type increases to some extent. When the atom location confusion with the anti-spinel type appears in LiMn2O4, both the initial discharging capacity and cycling properties of LiMn2O4 reduce. However, the atom location confusion with 16c type does not affect the charge and discharge properties of LiMn2O4.
Key words: Li-ion battery; cathode; LiMn2O4; confused degree; electrochemical property
1 Introduction
Lithium ion batteries possess a series of advantages such as single high voltage, large specific capacity, long cycling life and no memory effect, which makes them have good application prospects in the fields of portable electric apparatus, electric tools and electric automobiles. The properties of cathode material determine the comprehensive properties of the lithium ion battery to a great extent[1]. So, the researches and developments become hot topics and people pay more attention to them[2-4]. In the already commercialized cathode material at present, more than 90% is LiCoO2. Co resources are deficient and toxicant, which makes the lithium ion batteries have high cost and produces pollution to the environment, so people have kept looking for green cathode material with low costs and good properties since the lithium ion battery comes out[5-8]. LiMn2O4 with low cost, abundant resources and no environmental pollution, will be considered as the ideal cathode material. However, LiMn2O4 with spinel structure has Jahn-Teller effect, which can cause the capacity to decay very fast during charge and discharge [9], and the dissolution of Mn can cause further deterioration of cycling properties at high temperature (≥55 ℃)[10-11]. For this reason, many methods, such as doping and surface coating method, have been adopted to improve the cycling properties of LiMn2O4[12-13]. In the present study, the type of atom location confused degree, the confused degree and judgement method in LiMn2O4 were analyzed. The effect of preparation process on structure and electrochemical properties of LiMn2O4 was also investigated.
2 Experimental
The xerogel precursor was prepared by citric acid complexing method using lithium acetate and manganese acetate as raw materials. Then, the obtained xerogel precursor was sintered at 300 ℃ for 6 h. After that, it was sintered at various temperatures for 6 h.
The structure of the LiMn2O4 was analyzed by D/max-rB X-ray diffractometer with the radiation of Cu Kα, the tube voltage of 50 kV, the tube current of 50 mA and the scanning ratio of 5 (?)/min.
LiMn2O4, conductive carbon black, plasticizer DBP and polymer according to mass ratio of 78?4?10.2?7.8 and dispersant acetone were mixed to prepare cathode film with a thickness of 0.2 mm on a TB-160-ZE type instrument. Cathode film, polypropylene film, graphite anode film were assembled into a battery in a vacuum glove box and electrolytic liquid was EC+DMC+1 mol/L LiPF6.
The charge and discharge experiment was carried out on a BFGS-9256 type instrument. The charge and discharge rate was 0.3C and voltage range was from 3.0 to 4.3 V.
3 Results and discussion
3.1 Effect of sintering temperature on atom location in LiMn2O4
Fig.1 shows XRD patterns of LiMn2O4 prepared at various temperatures. It can be seen that the prepared products are mainly composed of LiMn2O4 with spinel structure sintered at various temperatures and a small amount of manganese oxides can be found after being sintered at 450-650 ℃. When the sintering temperature changes, peak intensity ratio of LiMn2O4 (I111/I311, I311/I400) is different to some extent. Impurities and their contents in LiMn2O4 are listed in Table 1. It can be found that more Mn2+ exist in LiMn2O4 when the xerogel precursor is sintered at low temperature.
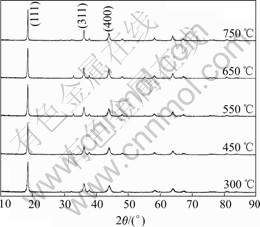
Fig.1 XRD patterns of LiMn2O4 sintered at various temperatures
Table 1 Impurities and their contents in LiMn2O4 sintered at various temperatures
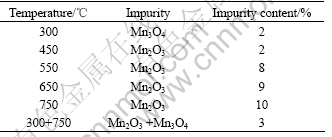
According to the crystal X-ray diffraction principle, for powder samples, the relative intensity of crystal face (HKL), IHKL, can be expressed as

where PHKL is the multiplicity factor,
is the structure factor,
is the angle factor, A(θ) is the absorbing factor and exp(-2M) is the temperature factor.
If the influence of the absorbing factor and temperature factor is neglected, the following formula can be obtained:

where C is a constant.
It is obvious that the change of peak intensity ratio is caused by the change of the structure factor. The value of structure factor |FHKL|2 depends on the atom kind, quantity and position in the cell. |FHKL|2 can be written as

where FHKL is the structure amplitude.
The expression formula of FHKL is

where fj is the atom scattering factor of j atom in cell, Xj, Yj and Zj are the coordinate of j atom in cell.
There are 4 lattice points in the cell of face center cubic lattice and their coordinates are as follows:

According to Eqn.(3),
,
and
can be respectively calculated as follows:
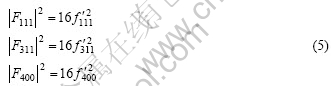
where
is the scattering factor of face center cubic lattice.
Fig.2 shows the schematic diagram of lattice atom group of LiMn2O4. The structure of LiMn2O4 belongs to the face center cubic lattice and each lattice is made up of 2 lithium atoms, 4 manganese atoms and 8 oxygen atoms.
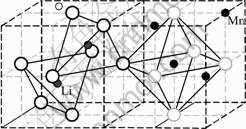
Fig.2 Schematic diagram of lattice atom group
If the coordinate origin is at the line centre of two lithium atoms, the coordinates of 8 oxygen atoms are respectively:
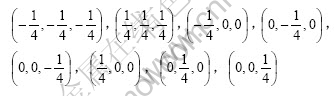

The coordinates of 2 lithium atoms are
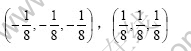
According to Eqn.(4), the following formulae can be obtained:
The corresponding atom scattering factors of (111) diffraction peak of Mn and Li (2θ=18.6?, λ=0.154 06 nm, sinθ/λ=1 nm-1) is respectively

The corresponding atom scattering factors of (311) diffraction peak of Mn and Li (2θ=36?, λ=0.154 06 nm, sinθ/λ=2 nm-1) are respectively

The corresponding atom scattering factors of (400) diffraction peak of Mn and Li (2θ=44?, λ=0.154 06 nm, sinθ/λ=2.4 nm-1) are respectively

Putting them into Eqn.(6 ), we can obtain:

According to Eqns.(2) and (5), the following equations can be obtained:
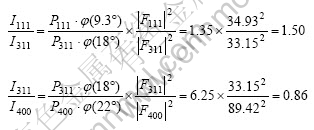
However, if the atom location confusion appears in LiMn2O4, for example, some lithium atoms and manganese ions are exchanged to form anti-spinel structure, then the structural formula can be written as [Li1-γMnγ]8a[Mn2-γLiγ]16dO4, where γ can be regarded as the confused degree. Thus, the scattering factor of lattice
in Eqn.(6) can be written as
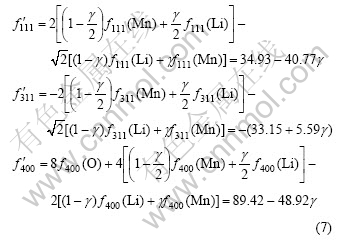
Fig.3 shows the relationship of I111/I311, I311/I400 and γ value obtained from Eqns.(2), (5) and (7). It can be found that I311/I400 value increases, but I111/I311 value reduces with the increase of γ value. Therefore, the characteristic of the curves is that I311/I400 value increases, and I111/I311 value reduces with increasing confused degree γ value when lithium ion and manganese ion exchange to form atom location confusion with the anti-spinel structure in LiMn2O4.
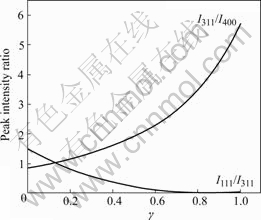
Fig.3 Relationship between peak intensity ratio and γ value with atom location confusion by anti-spinel type
If the exchange between lithium and manganese atoms does not appear in atom location confusion, the most possibility is that the lithium ion occupies idle 16c position because tetrahedron interval of 48f position is relatively less and the distance to high spin manganese ion is relatively shot. At this moment, the coordinates of the lithium ion that occupies 16c position are

Supposing the lithium ion amount occupying 16c position is γ (0<γ<1), the following equation can be obtained according to Eqn.(4):
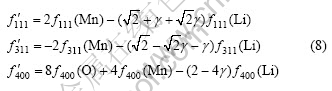
Similarly, From Eqns.(2), (5) and (8) the relationship between I311/I400, I111/I311 and γ value can be respectively obtained, as shown in Fig.4. It can be found that both I311/I400 and I111/I311 values reduce with the increase of γ value, however, the change trend is little. Therefore, the characteristic of the curves is that both I311/I400 and I111/I311 values reduce with increasing confused degree γ when 16c atom location confusion appears in LiMn2O4.
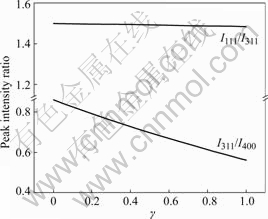
Fig.4 Relationship between peak intensity ratio and γ value with atom location confusion by 16c type
Table 2 lists the effect of sintering temperatures on peak intensity ratios I111/I311 and I311/I400 according to the experimental result in Fig.1. It can be found that the confused degree with anti-spinel type decreases, but that with 16c type increases with the increase of sintering temperatures.
Table 2 Effect of sintering temperature on peak intensity ratio
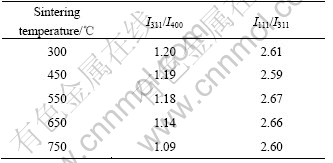
Local structure of LiMn2O4 was studied by YAN et al[14] by adopting synchronous radiation XAFS. The results indicate that the coordinate number of the second-story of Mn—Mn is smaller than 6 in LiMn2O4 when the sintering temperature is low (400 ℃), while the coordinate number of the second-story of Mn—Mn equals 6 in LiMn2O4 when the sintering temperature is high (700 ℃). This conclusion is identical with our above- mentioned results. When the atom location confusion with anti-spinel type appears in LiMn2O4, some manganese ions occupy the 8a location far away from the 16d location. At this time, the coordinate number of the second-story of Mn—Mn will decline.
It can be found from Tables 1 and 2, Mn2+ exists in LiMn2O4 and it is apt to produce atom location confusion with anti-spinel type when LiMn2O4 is sintered at the low temperature. According to the crystal field theory, manganese ion and oxygen ion whether to form coordinate tetrahedron or coordinate octahedra depends on the crystal field steady energy(CFSE) of manganese ion in these two coordinate polyhedrons. For Mn3+ ion, its CFSE is 136.08 J/mol[15] and 40.32 J/mol when it forms coordinate octahedra and tetrahedron with oxygen, respectively. Namely, the selection location energy of Mn3+ to form octahedra is 95.76 J/mol. So Mn3+ is easy to form coordinate octahedra with oxygen. For Mn2+, its CFSE equals zero when it forms the coordinate tetrahedron or octahedra with oxygen, so Mn2+ can enter either octahedra or tetrahedron interval. When LiMn2O4 is prepared at the low temperature, Mn2+ resolved out from the precursor may enter the tetrahedron interval position in LiMn2O4, meanwhile, Li+ is forced to enter other positions, which produces atom location confusion with anti-spinel type. The result of TREUIL et al[16] indicates that it helps to produce Mn3+ with high content when sintering Li-Mn-O compound at the high temperature, which supports the above-mentioned viewpoint.
3.2 Effect of atom location on electrochemical pro- perties of LiMn2O4
It can be found from Table 2 that the value of I311/I400 prepared at 450 ℃ is relatively large but the value of I111/I311 is relatively small in LiMn2O4 compared with those of LiMn2O4 prepared at 750 ℃, which indicates that LiMn2O4 prepared at 450 ℃ has bigger atom location confusion with anti-spinel type. Two kinds of samples are prepared into the test battery to carry out the charge and discharge test and the test results are shown in Fig.5. It can be seen that the initial discharge capacity of LiMn2O4 prepared at 450 ℃ is relatively lower and the capacity fading is faster during discharge.
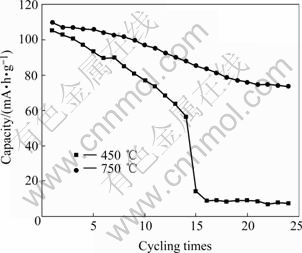
Fig.5 Curves of capacity vs cycling times of LiMn2O4 sintered at different temperatures
In LiMn2O4 with spinel structure, the oxygen ion occupies the 32e location of face centre cubic, the lithium ion lies in the 8a location of its tetrahedron interval and the manganese ion lies in the 16d location of its octahedra interval. In addition, there are idle tetrahedron interval 48f location and octahedra interval 16c location. Octahedra in 16c location and tetrahedron in 8a location are on the same face to form the three- dimensional path of the lithium ion, that is, 8a-16c-8a, as shown in Fig.6[17].
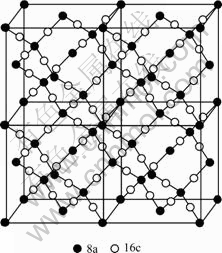
Fig.6 Diffusion passway of lithium ion in spinel structure
When the atom location confusion appears in LiMn2O4, the manganese ion occupies 8a position and the lithium ion enters 16d position. It locally forms the anti-spinel structure. Then, the lithium in 16d position can not be moved without the diffusion path, so the capacity reduces. Meanwhile, the diffusion path of the manganese ion occupying 8a position will stop the diffusion of the lithium ion and reduce diffusion rate of the lithium ion, which increases the density of the lithium ion on the particle surface of LiMn2O4, aggravates Jahn-Teller effect and accelerates capacity fading. So, LiMn2O4 prepared at 750 ℃ has relatively high initial capacity and cycling properties.
4 Conclusions
1) The atom location confused degree increases with the decrease of the X-ray diffraction peak intensity ratio of I111/I311 in LiMn2O4. The type of confused degree depends on the variation tendencies of I311/I400 and I111/I311. If their variation tendency is the same, it belongs to the 16c type and if the variation tendency is contrary, it belongs to the anti-spinel type.
2) When sintering temperature is low, it is apt to produce the atom location confusion with anti-spinel type in LiMn2O4. With the increase of sintering temperature, the atom location confused degree with anti-spinel type gradually reduces, however, the atom location confused degree with 16c type increases to some extent.
3) Initial discharge capacity reduces and cycling properties drop when there occurs atom location confusion with anti-spinel type in LiMn2O4. However, the atom location confusion with 16c type does not affect the charge and discharge properties of LiMn2O4.
References
[1] SCROSATI B. Recent advances in lithium ion battery materials [J]. Electrochimica Acta, 2000, 45(15/16): 2461-2466.
[2] Liu Han-san, Yang Yong, Zhang Jiu-jun. Investigation and improvement on the storage property of LiNi0.8Co0.2O2 as a cathode material for lithium-ion batteries [J]. J Power Sources, 2006, 162(1): 644-650.
[3] EINELI Y, URIAN R C, WEN W, MUKERJEE S. Low temperature performance of copper/nickel modified LiMn2O4 spinels [J]. Electrochimica Acta, 2005, 50(9): 1931-1937.
[4] HU Guo-he, Wang Xiao-bing, Chen Fang, Zhou Jian-yin, Li Ren-gui, Deng Zheng-hua. Study of the electrochemical performance of spinel LiMn2O4 at high temperature based on the polymer modified electrode [J]. Electrochemistry Communications, 2005, 7(4): 383-388.
[5] YOON W S, CHUNG K Y, BALASUBRAMANIAN M, HANSON J, MCBREEN J, Yang Xiao-qing. Time-resolved XRD study on the thermal decomposition of nickel-based layered cathode materials for Li-ion batteries [J]. J Power Sources, 2006, 163(1): 219-222.
[6] AMDOUNI N, GENDRON F, MAUGER A, ZARROUK H, JULIEN C M. LiMn2-yCoyO4 (0≤y≤1) intercalation compounds synthesized from wet-chemical route [J]. Materials Science and Engineering B,2006, 129(1/3):64-75.
[7] CHAN H W, DUH J G, SHEEN S R. Microstructure and electrochemical properties of LBO-coated Li-excess Li1+xMn2O4 cathode material at elevated temperature for Li-ion battery [J]. Electrochimica Acta, 2006,51(18): 3645-3651.
[8] WANG Zhi-xing, CAO Si-hai, LI Xin-hai, GUO Hua-jun, PENG Wen-jie. Effect of initial Li/(Mn+Ni) mole ratio on electrochemical performance of LiNi0.5Mn0.5O2 [J]. The Chinese Journal of Nonferrous Metals, 2006, 16(8): 1434-1438. (in Chinese)
[9] THACKERAY M M, MANSUETTO M F, BATES J B. Structural stability of LiMn2O4 electrodes for lithium batteries [J]. J Power Sources, 1997, 68(1): 153-158.
[10] AMATUCCI G G, SCHMUTZ C N, BLYR A. Materials’ effects on the elevated and room temperature performance of C/LiMn2O4 Li-ion batteries [J]. J Power Sources, 1997, 69(1/2): 11-25.
[11] Su Yu-chang, Xiao Dai-hong, Chen Ding, Du Ruo-xin. LiMn2O4 special capacity attenuation in high temperature [J]. Guangzhou Chemistry, 2000, 25(3): 5-21. (in Chinese)
[12] LU Shi-gang, LI Ming-xun, HUANG Song-tao, CAI Zhen-ping. Studies on process of LiMn2O4 modified by LiCoO2 [J]. Chinese Journal of Inorganic Chemistry, 2004, 20(4): 455-458. (in Chinese)
[13] YU Li-hong, Qiu Xin-ping, XI Jing-yu, Zhu Wen-tao, Chen Li-quan. Enhanced high-potential and elevated-temperature cycling stability of LiMn2O4 cathode by TiO2 modification for Li-ion battery [J]. Electrochimica Acta, 2006, 51(28): 6406-6411.
[14] Yan Wen-sheng, Wang Wen-lou, WU Min-chang, Wei Shi-qiang. Local structure of spinel LiMn2O4 cathode material studied by X-ray absorption fine structure [J]. Acta Physica Sinica, 2002, 51(10): 2303-2307. (in Chinese)
[15] LUO Gu-feng. Crystallography Discussion [M]. Beijing: Geologic Publishing Company, 1985. (in Chinese)
[16] TREUIL N, LABRUGERE C, MENETRIER M. Relationship between chemical bonding nature and electrochemical property of LiMn2O4 spinel oxides with various particle sizes: “Electrochemical grafting” concept [J]. J Physical Chemistry B, 1999, 103(12): 2100-2106.
[17] SHEN Pei-zhi. Preparation and Properties of Lithium Ion Battery Cathode Materials LiMn2O4 [D]. Xinjiang University, 2005.
Corresponding author: YU Ze-min; Tel: +86-451-86392566; E-mail: yuzem000@yahoo.com.cn
(Edited by YANG Bing)