
Preparation and mechanism of calcium phosphate coatings on chemical modified carbon fibers by biomineralization
HUANG Su-ping(黄苏萍), ZHOU Ke-chao(周科朝), LI Zhi-you(李志友)
State Key Laboratory of Powder Metallurgy, Central South University, Changsha 410083, China
Received 7 March 2007; accepted 5 September 2007
Abstract: In order to prepare HA coatings on the carbon fibers, chemical modification and biomineralization processes were applied. The phase components, morphologies, and possible growth mechanism of calcium phosphate were studied by infrared spectroscopy(IR), X-ray diffractometry(XRD) and scanning electron microscopy(SEM). The results show that calcium phosphate coating on carbon fibers can be obtained by biomineralization. But the phase components and morphologies of calcium phosphate coatings are different due to different modification methods. Plate-like CaHPO4?2H2O (DCPD) crystals grow from one site of the active centre by HNO3 treatment. While on the para-aminobenzoic acid treated fibers, the coating is composed of nano-structural HA crystal homogeneously. This is because the —COOH functional groups of para-aminobenzoic acid graft on fibers, with negative charge and arranged structure, accelerating the HA crystal nucleation and crystallization on the carbon fibers.
Key words: calcium phosphate coating; carbon fibers; chemical modification; biomineralization
1 Introduction
Carbon fibers reinforced hydroxyapatite [Ca10(PO4)6(OH)2, HA] was considered one of the best candidates for bone substitution material, due to its exceptional mechanical properties (the elastic modulus close to that of human bone), good biocompatibility and biostability (such as no release of dissoluble products, not being easily stress corroded, and no degradation with time)[1-5]. However, the significant difference in surface properties and coefficients of heat expansion between HA and carbon fibers leads to the poor mechanical properties, which limits its application in field of bone replacing materials.
Studies[6-7] showed that the mechanical properties of fiber-reinforced composites could be improved by the use of ceramic coated fibers, because ceramic coatings can optimize the fiber-matrix bonding, protect from the oxidation of non-oxide fibers, prevent fiber/matrix interfacial degradation at elevated temperature; and as a mid-layer between HA and carbon fibers, reduce the gradient of thermal stress on its phase boundary with HA matrix. Therefore, the method for preparing calcium phosphate coatings on the carbon fibers is the key process to improve the properties of carbon fiber reinforced HA composite.
Many methods have been reported to prepare ceramic coatings on carbon fibers, such as sol-gel, and electro-deposition[8-12]. In this work, biomineralization process was introduced to prepare calcium phosphate coatings. The phase component, morphology and the possible growth mechanism of coatings were studied.
2 Experimental
Carbon fibers (Lanzhou Carbon Co. Ltd, with diameter of 6-8 μm, surface area of 0.78 g/m2) were manufactured from polyacrylonitrile precursor through a carbonization process. Analytic-grade Ca(NO3)2?4H2O, (NH4)2HPO4 (99.5%), NaCl and NaHCO3 were used to prepare the simulated body fluid(SBF). HCl and NH4OH were used to adjust pH to 7.4. The concentrations of ions are listed in Table 1.
Table 1 Concentrations of ions in simulated body fluid (10-4 mol/L)

Carbon fibers were divided into two groups. One group was treated by nitric acid (68%, mass fraction, analytical grade) at 115 ℃ for 3 h, and then the oxidized fibers were rinsed with distilled water until the pH of the resulting wash water maintained at a constant value. Subsequently, the oxidized fibers were dried in an oven at 75 ℃ for about 10 h.
The other group of carbon fibers was immerged into 2% (mass fraction) para-aminobenzoic acid solutions at 37 ℃ for 24 h, and then the carbon fibers were immerged into 2% acetimeter solution for 2 h. Subsequently, the modified fibers were rinsed with distilled water, alcohol and tetrahydrofuran(THF) in sequence, and then dried in an oven at 75 ℃ for about 10 h.
The single-tow tensile strengths of carbon fibers were measured according to ASTM standard D4018— 81[12]. These data are averages of more than ten measurements at each modification time, with the relative standard deviation around 10%. The functional groups on carbon fibers were determined by infrared spectroscopy(IR). The crystallized phase composition of coatings was quantitatively identified by X-ray diffractometry(XRD). The morphology and structure of the coatings were observed by Scanning Electron Microscope(SEM, JEOL-5600LV, Noran Instrument) after coating with gold by ion beam sputtering.
3 Results
The creation of a large functional group concentration on fiber surfaces is essential in order to induce the growth of calcium phosphate coatings on fibers through interfacial chemical bonding. The higher the density of functional surface groups was, the larger the possibility of surface reactions could be; as a result, the interfacial chemical bonding strength of coatings to fiber surfaces could be promoted.
Many methods have been reported to create functional groups on fibers. Electrochemical methods do not result in a sufficient number of functional groups per unit of surface area to play a significant role in fiber resin adhesion[11]. Treating fibers with plasma yields identical surface functional densities. But compared with nitric acid oxidation or para-aminobenzoic acid modify- cation and plasma oxidation, carbon fibers resulted in low surface functional group densities[12]. Fig.1 shows the IR spectra of carbon fibers before and after treatment. As shown in Fig.1, the spectrum of untreated carbon fiber was a smooth curve without any peaks. However many obvious absorption peaks appeared on those of treated carbon fibers. On the spectrum of HNO3 treated fibers, the peaks at 3 650 cm-1, 3 000 cm-1 and 1 690 cm-1 represent the —OH peak of phenolic, the —OH stretching peak of carboxyl group and the C=O stretching peak of carbonyl group, respectively, which indicates that there are several functional groups (such as carboxylic acid groups and phenolic hydroxyl groups) have appeared on carbon fiber surface after HNO3 oxidization. On the spectrum of para-aminobenzoic acid treated fibers, the peaks at 3 030-2 910 cm-1, 1 051- 1 382 cm-1 and 2 978 cm-1 respectively represent the phenyl stretching peak, the C—H stretching peak and the —OH stretching peak of carboxyl group, while the characteristic peak of —NH2 at 3 368.07 cm-1 dis- appears, which demonstrates that the para-aminobenzoic acid has been grafted on carbon fiber surface by chemical reaction.
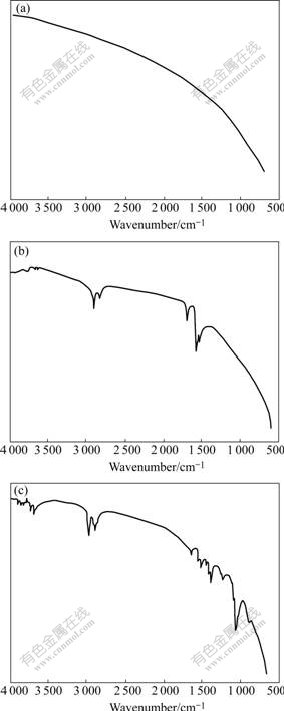
Fig.1 IR spectra of carbon fibers: (a) Untreated; (b) HNO3 treated; (c) Para-aminobenzoic acid treated
The tensile strengths of carbon fibers, before and after modification, were tested by the single-tow method according to ASTM D4018—81. The results are listed in Table 2. Compared with the untreated carbon fibers, the tensile strengths of treated fibers decreased little. This is because nitric acid and para-aminobenzoic acid selectively react with the less crystallized regions. The high-modulus carbon fibers, graphitized at higher temperature, exhibit larger crystallites, fewer microvoids, and a larger area fraction of basal planes at the surface. Thus, PAN-based high-modulus fibers aren’t easier to be oxidized, causing a littler tensile strength loss.
Table 2 Strengths of carbon fibers before and after modifica- tion
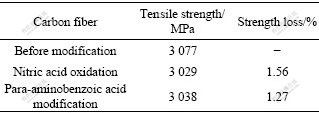
Fig.2 shows the XRD patterns of calcium phosphate grown on the carbon fibers. The resultant grown on the HNO3 treated carbon fibers is dicalcium phosphate dehydrate(CaHPO4?2H2O, DCPD), while the coatings by para-aminobenzoic acid treated carbon fibers is pure hydroxyapatite crystal.
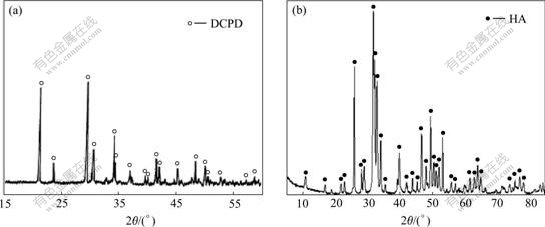
Fig.2 XRD patterns of calcium phosphate grown on carbon fibers: (a) HNO3 treated carbon fibers; (b) Para-aminobenzoic acid treated carbon fibers
Fig.3 shows the crystalline morphologies of calcium phosphate on carbon fibers. It can be seen that DCPD grown on HNO3 treated carbon fibers was plate-like, and all the plates crystallized from one active point of the fibers (as shown in Fig.3(a)). Magnified image showed that DCPD crystals grew directly from the fibers, and all the crystals adhered tightly to the fibers (as shown in Fig.3(b)). Fig.3(c) and Fig.3(d) show the crystal morphologies of HA crystals on para-aminobenzoic acid treated carbon fibers. It can be seen that the HA crystals grew homogeneously, and the size of crystal was 50 nm.
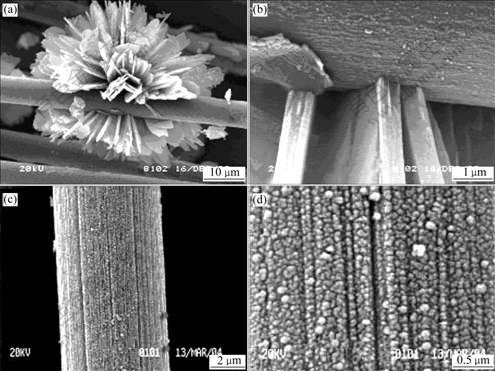
Fig.3 Morphologies of calcium phosphate grown on carbon fibers: (a, b) HNO3 treated carbon fibers; (c, d) Para-aminobenzoic acid treated carbon fibers
4 Discussion
Evidence has been presented that the structure of the functional groups (including the size of the nucleation sites, the number of active sites and the functional group arrangement) and interactions with the crystal at the crystal/fiber interface are the key factors to the regulation of crystal nucleation during biomineralization. Studies[13-16] have reported that —COOH functional group can not only accelerate the crystal growth rate of hydroxyapatite, but also orientate the crystallographic (0001) face. In this work, two kinds of treated fibers all can induce the growth of calcium phosphate on the fibers. This is because the functional groups on fibers, acted as nucleation center for hydroxyapatite crystal growth, can attract and react with ions (such as Ca2+ and
) of SBF, which can lower the activation energy barrier of nucleation by lowering interfacial energy and increasing the supersaturation of the range nearby groups, and result in the formation of the calcium phosphate coatings. But the structure and morphology of coatings are different. This is mainly due to the difference in the number and structure of functional groups on the fibers. On the HNO3 treated fibers, there are many kinds of functional groups, such as —COOH, —OH and —C=O (as shown in Fig.4). Due to the fact that the number of functional groups on HNO3 treated fibers is few and the type of functional groups is different, only few DCPD crystals grow from the site of —COOH active nucleation center. While on the para-aminobenzoic acid treated carbon fibers, the functional groups are only —COOH (as shown in Fig.5). The —COOH, with arranged and ordered structure, is attributed to the HA crystal nucleation and growth, resulting in homogeneous structure.
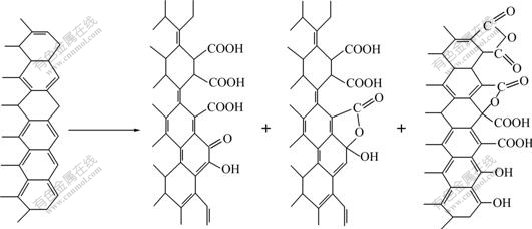
Fig.4 Surface construction of carbon fibers before and after HNO3 oxidization
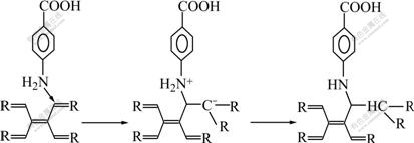
Fig.5 Reaction mechanism between para-aminobenzoic acid and carbon fibers
5 Conclusions
1) Carbon fibers after chemical modification can induce calcium phosphate crystal growing on its surface by the process of biomineralization. But different modification methods cause different phase components and morphologies of calcium phosphate coatings.
2) Resultants on HNO3 treated fibers are plate-like DCPD crystals grown from one site of the active center; while on the para-aminobenzoic acid treated fibers, the coating is homogeneous nano-structured HA crystals. The reasons are that the functional groups grafted onto the carbon fibers treated by para-aminobenzoic acid is only —COOH group, with negative charge and arranged structure, which can accelerate the HA crystal nucleation and crystallization on the carbon fibers. While on the HNO3 treated fibers, there are several kinds of functional groups with disordered structure, which can only induce the DCPD crystal on the active sites.
References
[1] FU Tao, ZHAO Jun-liang, XU Ke-wei. The designable elastic modulus of 3-D fabric reinforced biocomposites [J]. Materials Letters, 2007, 61(2): 330-333.
[2] PARK K, VASILOS T. Characteristics of carbon fiber-reinforced calcium phosphate composites by hot pressing [J]. J Mater Sci Lett, 1997, 16(5): 985-987.
[3] SUCHANEK W, YASHIMA M, KAKIHANA M. Processing and mechanical properties of hydroxyapatite reinforced with hydroxyapatite whiskers [J]. Biomaterials, 1996, 17(17): 1715-1723.
[4] SLOSARCZYK A, KLISCH M, BLAZEWICZ M. Hot press hydroxyapatite-carbon fibre composites [J]. J Euro Cer Soc, 2000, 20(7): 1397-1402.
[5] RUJS A J, MILTHORPE B K, SORRELL C C. Preparation of fibre-reinforced hydroxyapatite [J]. Intercerm, 1994, 43(1): 7-9.
[6] DORNER-REISELA A, BERROTHB K, NEUBAUERB R, NESTLERC K. Unreinforced and carbon fibre reinforced hydroxyapatite: Resistance against microabrasion [J]. Journal of the European Ceramic Society, 2004, 24(7): 2131-2139.
[7] HUANG Su-ping, HUANG Bai-yun, ZHOU Ke-chao, LI Zhi-you. Effects of coating on the mechanical properties of carbon fiber reinforced HAP composites [J]. Materials Letters, 2004, 58(27/28): 3582-3585.
[8] SUI Jin-ling, LI Mu-sen, L? Yu-peng, YIN Long-wei, SONG Yun-jing. Plasma-sprayed hydroxyapatite coatings on carbon/carbon composites [J]. Surface and Coatings Technology, 2004, 176(2,1): 188-192.
[9] ZHAO Jun-liang, FU Tao, HAN Yong, XU Ke-wei. Reinforcing hydroxyapatite/thermosetting epoxy composite with 3-D carbon fiber fabric through RTM processing [J]. Materials Letters, 2003, 58(1/2): 163-168.
[10] ZHITOMIRSKY I. Electrophoretic hydroxyapatite coatings and fibers [J]. Materials Letters, 2000, 42(4): 262-271.
[11] ZHITOMIRSKY I. Electrophoretic and electrolytic deposition of ceramic coatings on carbon fibers [J]. Journal of the European Ceramic Society, 1998, 18(7): 849-856.
[12] SUI Jin-ling, LI Mu-sen, L? Yu-peng, BAI Yun-qiang. The effect of plasma spraying power on the structure and mechanical properties of hydroxyapatite deposited onto carbon/carbon composites [J]. Surface & Coatings Technology, 2005, 190(2/3): 287-292.
[13] LIU Yong, HUANG Su-ping. Growth of hydroxyapatite crystal in the presence of organic film [J]. Materials Science & Technology, 2004, 20(2): 223-226.
[14] HUANG Su-ping, ZHOU Ke-chao. Controlled crystallization of hydroxyapatite under hexadecylamine self-assembled monalayer [J]. Trans Nonferrous Met Soc China, 2003, 13(3): 595-599.
[15] HUANG Su-ping, HUANG Bai-yun, ZHOU Ke-chao. The effect of organic groups and inorganic ions on the crystallization of hydroxyapatite [J]. Trans Nonferrous Met Soc China, 2004, 14(9): 1604-1608.
[16] HUANG Su-ping, ZHOU Ke-chao. Controlled crystallization of hydroxyapatite by the hexadecylamine organic film [J]. Chinese Journal of Materials Research, 2004, 18(1): 66-70.
Foundation item: Project(2006CB600904) supported by the National Basic Research Program of China; Project(07JJ3105) supported by the Natural Science Foundation of Hunan Province, China
Corresponding author: ZHOU Ke-chao; Tel: +86-731-8836264; Fax: +86-731-8710855; E-mail: huangsp1@mail.csu.edu.cn
(Edited by YANG Bing)