
Quantum chemical aided molecular design of ionic liquids as
green electrolytes for electrodeposition of active metals
TIAN Guo-cai(田国才), ZHOU Xue-jiao(周雪娇), LI Jian(李 坚), HUA Yi-xin(华一新)
Faculty of Materials and Metallurgical Engineering, Kunming University of Science and Technology,
Kunming 650093, China
Received 10 August 2009; accepted 15 September 2009
Abstract: Quantum chemical calculation was used to estimate the reduction potentials of 25 organic cations and the oxidation potentials of 11 anions. This information was used to select promising cations and anions for the preparation of ionic liquids as green electrolytes for electrodeposition of active metals. The reasonable linear correlations between the lowest unoccupied molecular orbital (LUMO) energies and the reduction potentials of cations, and the linear relationships between the oxidation potentials and the highest occupied molecular orbital (HOMO) energies of anions were obtained. The orders of electrochemical stability for cations and anions being obtained agree well with the experimental measurements. The suitable ionic liquids with sufficiently wide electrochemical windows for electrodeposition of active metals are suggested to be [Emim]NTf2, [Bmim]NTf2, [Bmim]BF4, [Bmim]PF6, [Bmim]CTf3, [Emim]BF4, [Emim]PF6, [Emim]CTf3..
Key words: ionic liquid; quantum chemical calculation; green electrolyte; electrodeposition; active metals; molecular design; electrochemical stability; electrochemical window
1 Introduction
The production value of active metals (such as aluminum, and magnesium) accounts for half that of the nonferrous metal, only lower than that of the steel, but its energy consumption is very high. The common method applied to obtaining active metals is the electrolysis of molten salts of active metals, which has many disadvantages such as high temperature, high energy consumption, serious equipment corrosion and high environmental pollution. Therefore, the development of new effective, low-temperature and environmentally- benign technologies for active metals processing to reduce energy consumption and to lower investment costs and the greenhouse gas emissions is an industrial demand and challenge for the 21st century[1]. The “green solvent” ionic liquids may offer potential as environmentally sensitive media and alternatives to active metals production processes.
Ionic liquids, also called room-temperature ionic liquids (ILs), are liquids composed completely of organic cations and inorganic/organic anions[2-5]. They have been widely applied to many fields such as organic synthesis, catalysis, electrochemistry, bio-catalysis, material science, and separation processes [2-5] due to their unique properties such as very low or negligible vapor pressure, wide electrochemical window, high electrochemical stability and ion conductivity[3-5]. Preliminary studies have shown that ionic liquids have potential as solvents for metal recovery[6-8]. It was found that almost all of the metals can be electrodeposited in ionic liquids, principally Li, Na, Al, Mg and Ti[9-10]. It was shown that the electro- deposition of Al from ionic liquids can save 30%-50% energy consumption compared with the traditional process, and this technology has been strongly supported as an industrial technology project by the partners of University of Alabama, Albany Research Center, Century Aluminum Company, Secat Inc. and University of Kentucky, USA.
Variations in cations and anions can produce different kinds of ionic liquids and the properties of ionic liquids depend on the structure of ions. Therefore, ILs are often referred as “designer solvents”[4]. However, in recent years, the number of possible cation and anion combinations has increased significantly so that a large number (1018) of ionic liquids could possibly exist[3-5]. The synthetic problem of being able to rationally design ambient temperature ionic liquids through variation of the anion and cation still remains to be thoroughly investigated, despite attempts to correlate structure with melting point. An important question is how to do and how we start from for a specific research? It is thus essential to develop a systematic method of selectively choosing a specific ionic pair, to be used as a predictive tool for rational design of specific ionic liquids.
A high electrochemical stability of the ionic liquid is very important for its electrochemical applications. The electrochemical stability of an ionic liquid is always manifested by the width of the electrochemical window[11]. The electrochemical window of a substance is the voltage range in which the substance doesn’t get oxidized or reduced. In general, for a specific ionic liquid, the oxidation potential is determined by the voltage of the anion oxidation and the reduction potential is determined by the voltage of the cation reduction. It was shown that electrochemical oxidation potentials of several anions can be correlated with their respective highest occupied molecular orbital (HOMO) energies and electrochemical reduction potentials (reductive stability) can be correlated with the lowest unoccupied molecular orbital (LUMO) energy level for many organic molecules [12-15] and anions[16]. So, we can design the specific ionic liquids with the largest electrochemical windows for active metals electro-depositions.
In this work, quantum chemical calculations were used to predict the electrochemical stability of several cations and anions of ionic liquids. The correlations between the electrochemical oxidation potentials of several anions with their respective HOMO energies and the relationships between the electrochemical reduction potentials of various anions with their respective LUMO energy levels were studied. On basis of these quantum chemical calculations and correlations, some suitable ionic liquids were suggested as electrolytes for the elcetrodeposition of active metals.
2 Computational methods
All of the stable structures of cations and anions were optimized and all of calculations of the conformation energies,HOMO and LUMO levels were performed with the GAUSSIAN 03 program[17]. On the basis of the famous Hohenberg and Kohn theorems, the density functional theory (DFT) provides a sound basis for the development of computational strategies for obtaining information about the energetics, structure, and electron properties of atoms, molecules or ions at much lower costs than traditional ab initio wave function techniques (for example, Hartree-Fock, M?ller-Plesset, configuration interaction, and coupled cluster theory) without accuracy loss[18-20].
The well-established Becke’s three-parameter hybrid function coupled with the correlation function of Lee, Yang, and Parr (B3LYP)[21], which is known to be reliable, particularly for calculations of closed-shell stable molecules and ions system, was chosen in this work. The reasonably large basis sets 6-311 G**[22] was used in all calculations. All of the stable structures of the cations and anions were obtained by the Berny analytical gradient optimization routines[23] and the convergence criteria were the same as the defaults in GAUSSIAN 03. All of the stable structures were verified carefully that there does not exist imaginary frequencies in their frequency calculations.
3 Results and discussion
It was previously found that a high energy level of the HOMO results in a less stable anion with respect to oxidation (it is easier to remove the electron from this orbital)[11, 24]. And it was assumed that a low energy level of the LUMO results in a less stable cation with respect to oxidation (it is easier to put an additional electron in this orbital). Therefore, it is expected that ionic liquids exhibit a large electrochemical window when the anion possesses a low HOMO energy level and the cation possesses a high LUMO energy level. In this case, the difference between the LUMO energy level of the cation and the HOMO energy level of the anion can be a good measure of the width of the electrochemical window.
Several widely used cations for preparation of ionic liquids such as pyridinium-, guanidinium-, formamidinium-, pyridazinium-, pyridinium-, imidazolium- and amidinium-based cations were selected to study the relationships between the reduction potentials and the LUMO energies of cations. The cations being considered are all similarly sized, and the differences in solving energies are neglected, so a direct correlation between LUMO energies and reduction potentials φred can be made. Fig.1 shows the optimized stable structure of 25 selected cations at B3LYP/6-311G** level in density functional theory. The structure parameters such as bond length and angle can be obtained by requesting from the authors. The conformation energies with zero-point correction and the LUMO energies of these cations calculated from the stable structure in Fig.1 are listed in Table 1. The experiment reduction potentials of various cations are also listed in Table 1.
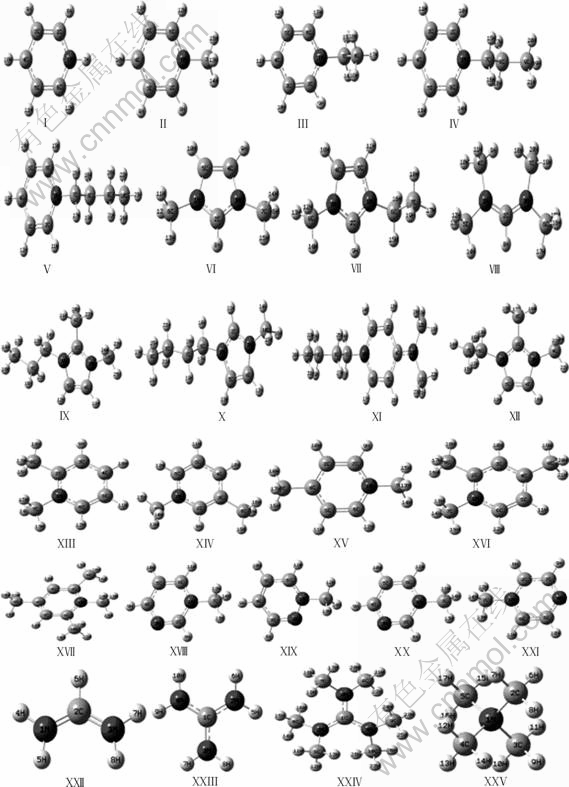
Fig.1 Optimized stable structures of various cations obtained at B3LYP/6-311G** level: Ⅰ Pyridinium; Ⅱ 1-methylpyridinium; Ⅲ 1-ethylpyridinium; Ⅳ 1-propylpyridinium; Ⅴ 1-butyl-pyridinium; Ⅵ 1, 3-dimethylimidazolium; Ⅶ l-methyl-3-ethyl- imidazolium; Ⅷ Tetramethylformamidinium; Ⅸ 1-propyl-2, 3-dimethylimidazolium; Ⅹ 1-methyl-3-butyl-imidazolium; Ⅺ l- butyl-4-(dimethylamino)pyridinium; Ⅻ 1, 2, 3-trimethylimidazolium; ⅩⅢ Tetramethylammonium; ⅩⅣ l, 2-dimethylpyridinium; ⅩⅤ 1, 3-dimethylpyridinium; ⅩⅥ 1, 4-dimethylpyridinium; ⅩⅦ 1, 2, 4-trimethylpyridinium; ⅩⅧ l, 2, 4, 6- tetramethylpyridinium; ⅩⅨ 1-methylpyridazinium; ⅩⅩ 1-methylpyrimidinium; ⅩⅪ 1-methylpyrazinium; ⅩⅫ 1, 2, 3- trimethylimidazo1ium; ⅩⅩⅢ Formamidinium; ⅩⅩⅣ Guanidinium; ⅩⅩⅤ Hexamethylguanidinium
Table 1 Conformation energies with zero-point correction and LUMO energies of 25 cations obtained at B3LYP/6-311G** level
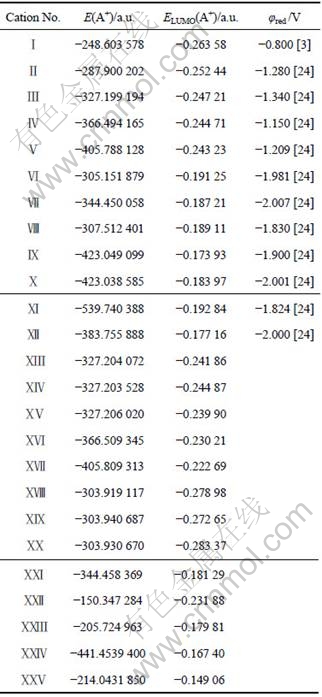
From Table 1, one can find that the aromatic 6- membered heterocycles having two ring nitrogens (ⅩⅦ -ⅩⅩ ) were predicted to be more easily reduced than the alkylpyridinium cations; thus, they were poor candidates for providing melts with a lower cathodic limit. The aromatic 5-membered heterocycles having two ring nitrogens (Ⅶ-Ⅸ, ⅩⅪ ) have LUMO energies significantly lower than the pyridinium based cations. The LUMO energies for the amidine (ⅩⅫ , Ⅹ) and guanidine (ⅩⅩⅢ , ⅩⅩⅣ ) based cations are also low. The order of the LUMO energies for various cations is: ⅩⅩⅤ>ⅩⅩⅣ >Ⅸ>Ⅻ>ⅩⅩⅢ >ⅩⅪ >Ⅹ>Ⅶ>Ⅷ>Ⅵ>Ⅺ>ⅩⅦ >ⅩⅥ >ⅩⅫ >ⅩⅤ>ⅩⅢ >Ⅴ>Ⅳ>ⅩⅣ>Ⅲ>Ⅱ>Ⅰ>ⅪⅩ>ⅩⅧ >ⅩⅩ. As mentioned above, a low energy level of the LUMO results in a less stable cation with respect to oxidation (it is easier to put an additional electron in this orbital). Thus, one can infer that the order in electrochemical stability of these cations is 1-alklylpyrazinium<pyridinium<amidine<imidazolium< guanidinium<quaternary ammonium, which agrees well with the experimental result (pyridinium<pyrazole<phosphonium<quaternary ammonium).
Fig.2 illustrates the correlation between the calculated LUMO energies ELUMO and measured reduction potentials φred for some anions in Table 1. It is shown in Fig.2 that a good linear correlation relationship between LUMO energies ELUMO and measured reduction potentials φred can be found although the scatter is quite substantial and the correlation equation is given by φred (V) = -4.1385-11.913 76 ELUMO (a.u.) with a calculated correlation coefficient of 0.917 83. It is found that the reduction potentials decrease with the increasing of LUMO energies of cations. From Table 1 and Fig.2, one can infer that the good cations for forming the ionic liquids with largest electrochemical windows may be ⅩⅩⅤ, ⅩⅩⅣ, Ⅸ, Ⅻ, ⅩⅩⅢ , ⅩⅫ , Ⅹ, Ⅶ, Ⅷ, Ⅵ, Ⅺ, etc.
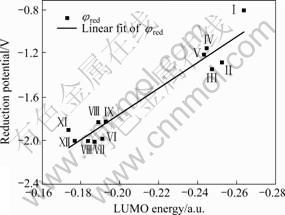
Fig.2 Correlation of LUMO energies (ELUMO) and reduction potentials (φred) of various cations
Various widely used anions for forming ionic liquids such as BF4-, NO3-, HSO4-, CF3COO-, CF3SO3-, C3F7COO-, NTf2-, CTf3-, PF6-, CF3BF3- and C2F5BF3- were selected to study the relationship between the oxidation potentials and the HUMO energies of anions. The optimized stable structures of 11 selected anions at B3LYP/6-311G** level in density functional theory are given in Fig.3. The structure parameters such as bond length and angle can be obtained by requesting from the authors. Table 2 lists the conformation energies with zero-point correction, the HOMO energies of these anions calculated from the stable structure, and the experiment oxidation potentials of several anions are also listed in.
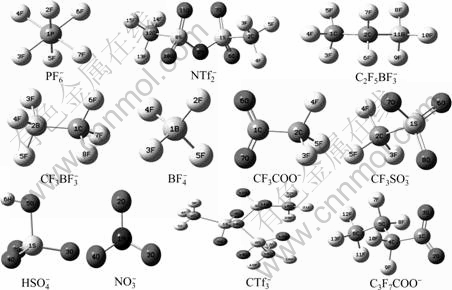
Fig.3 Optimized equilibrium stable structures of various anions obtained at B3LYP/6-311G** level
Table 2 Conformation energies with zero-point correction and HOMO energies of 11 anions obtained at B3LYP/6-311G** level
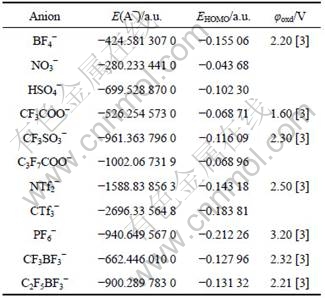
From Table 2, one can find that the order of the HOMO energies for various anions is as follows: NO3->F3COO->C3F7COO->HSO4->CF3SO3->CF3BF3->C2F5BF3->NTf2->BF4->CTf3->PF6-. As it is known that a high energy level of the highest occupied molecular orbital (HOMO) results in a less stable anion with respect to oxidation (it is easier to remove the electron from this orbital), the inferred order of electrochemical stability for anions is NO3-<CF3COO-<C3F7COO-<HSO4-<CF3SO3-<CF3BF3-<C2F5BF3-<NTf2-<BF4-<CTf3-<PF6-, which agrees well with the experimental result (Cl-, F-, Br-<AlCl4-, Al2Cl7-<BF4-, PF6-, AsF6-<CF3SO3-, NTf2-, (C2F5SO2)2N-, CTf3-).
The calculated HOMO energies EHOMO and measured oxidation potentials φoxd for several anions in Table 2 are shown in Fig.4. From Fig.4, one can build a reasonable linear correlation relationship between HOMO energies EHOMO and measured oxidation potentials φoxd. The correlation equation is given by φoxd(V)=0.932 3-10.270 36 EHOMO(a.u.) with a calculated correlation coefficient of 0.897 5. It is shown that the reduction potentials increase with the decreasing of HOMO energies of cations. From Table 2 and Fig.4, one can infer that the good anions for forming the ionic liquids with largest electrochemical windows may be CF3SO3-, CF3BF3-, C2F5BF3-, NTf2-, BF4-, CTf3-, PF6-, etc.
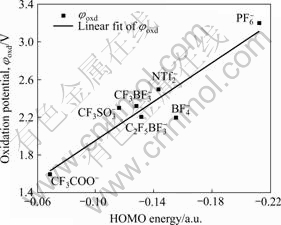
Fig.4 Correlation of HOMO energies and oxidation potentials of various anions
In general, for a specific ionic liquid, the oxidation potential is determined by the voltage of the anion oxidation, the reduction potential is determined by the voltage of the cation reductions, and the electrochemical windows of the ionic liquid is determined by the absolute value of the difference between the oxidation potential and reduction potential. As mentioned above, the anions with higher oxidation potentials are NTf2-, BF4-, CTf3-, PF6- etc. and the cations with sufficiently low reduction potentials are ⅩⅩⅤ , ⅩⅩⅣ , Ⅸ, Ⅻ, ⅩⅩⅢ , ⅩⅫ , Ⅹ, Ⅶ, Ⅷ, Ⅵ, and Ⅺ etc. On the basis of these quantum chemical calculations, the suitable ionic liquids for electrodepostion of active metals can be inferred to be [Emim]NTf2, [Bmim]NTf2, [Bmim]BF4, [Bmim]PF6, [Bmim]CTf3, [Emim]BF4, [Emim]PF6, [Emim]CTf3, and so on.
4 Conclusions
1) Quantum chemical molecular orbital calculations may be used to estimate the reduction potentials of a variety of cations and the oxidation potentials of various anions. This information may be used to select promising cations and anions for the preparation of ionic liquids for electrodepostion of active metals.
2) The reasonable linear correlations between the LUMO energy and the reduction potentials for cations are obtained. The inferred order in electrochemical stability is 1-alklylpyrazinium<pyridinium<amidine<imidazolium<guanidinium<quaternary ammonium, which agrees well with the experimental result.
3) The linear relationships between the HOMO energy and the oxidation potentials of anions is found and the inferred order of electrochemical stability for anions is NO3-<CF3COO-<C3F7COO-<HSO4-<CF3SO3-<CF3BF3-<C2F5BF3-<NTf2-<BF4-<CTf3-<PF6-, which agrees well with the experimental result.
4) The suitable ionic liquids with sufficiently wide electrochemical windows which are attractive candidates for electrodeposition of active metals are [Emim]NTf2, [Bmim]NTf2, [Bmim]BF4, [Bmim]PF6, [Bmim]CTf3, [Emim]BF4, [Emim]PF6, [Emim]CTf3, and so on.
References
[1] CHERGINETS V. Handbook of solvents [M]. WYPYCH G. Toronto: Chem Tec Publishing House, 2001: 1484-1496.
[2] WELTON T. Room-temperature ionic liquids: Solvents for synthesis and catalysis [J]. Chem Rev, 1999, 99(8): 2071-2083.
[3] LI Ru-xiong. Green solvents-synthesis and application of ionic liquids [M]. Beijing: Chemical Industry Press, 2004: 10-16. (in Chinese)
[4] ZHANG Suo-jiang, L? Xing-mei. Ionic liquids—from fundamental study to industrial application [M]. Beijing: Science Press,2006: 12-16. (in Chinese)
[5] DENG You-quan. Ionic liquids—properties, preparation and application [M]. Beijing: China SINOPEC-Press, 2006: 10-16. (in Chinese)
[6] WHITEHEAD J A, LAWRENCE G A, MCCLUSKEY A. Green leaching: Recyclable and selective leaching of gold-bearing ore in an ionic liquid [J]. Green Chem, 2004, 6: 313-318.
[7] THIED R C, SEDDON K R, PITNER W R, ROONEY D W. Nuclear fuel reprocessing, WO9941752[P]. 1999.
[8] ABBOTT A P, KATY J, MCKENZIE T. Application of ionic liquids to the electrodeposition of metals [J]. Phys Chem Chem Phys, 2006, 8: 4265-4279.
[9] ZHANG M, KAMAVARAM V, REDDY R G. Aluminum electrowinning in ionic liquids at low temperature [J]. Light Metals, 2005: 583-588.
[10] WU B, REDDY R G, ROGERS R D. Production refining and recycling of lightweight and reactive metals in ionic liquids: US2002070122 [P]. 2002.
[11] KROON M C, BUIJS W, PETER C J, WITKAMP G J. Decomposition of ionic liquids in electrochemical processing [J]. Green Chem, 2006, 8: 241-245.
[12] DEWAR M J S, KOLLMAR H W. Vertical ionization potentials of radicals by the MINDo/3 method [J]. Theoret Chim Acta, 1975, 36: 237-239.
[13] DEWAR M J S, KOLLMAR H W. Ground states of conjugated molecules. ⅩⅫ . Polarographic reduction potentials of hydrocarbons [J]. J Am Soc, 1970, 23: 5555-5559.
[14] MILLER L, SIMPLE L. Comprehensive correlation of organic oxidation and ionization potentials [J]. J Org Chem, 1972, 37: 916-918.
[15] WINGET P. Computational electrochemistry: Aqueous one-electron oxidation potentials for substituted anilines [J]. Phys Chem Chem Phys, 2000, 2: 1231-1239.
[16] KOCH V R. The intrinsic anodic stability of several anions comprising solvent-free ionic liquids [J]. J Electrochem Soc, 1996, 143: 798-803.
[17] FRISCH M J, TRUCKS G W, SCHLEGEL H B, SCUSERIA G E, POPLE J A. GAUSSIAN 03 Revision E01 [Z]. Gaussian, Inc: Wallingford, CT, 2008.
[18] GEERLINGS P, de PROFT F, LANGENAEKER W. Conceptual density functional theory [J]. Chem Rev, 2003, 103: 1793-1810.
[19] TALATY E R, RAJA S, STORHAUG V J, DOLLE A, ROBERT C W. Raman and infrared spectra and ab initio calculations of C2-4MIM imidazolium hexafluorophosphate ionic liquids [J]. J Phys Chem B, 2004, 108: 13177-13186.
[20] MENG Z, DOLLE A, CARPER W R. Gas-phase model of an ionic liquid: Semiempirical and ab initio bonding and molecular structure [J]. J Mol Struct (THEOCHEM), 2002, 585: 119-124.
[21] LAGROST C, GMOUH S, VAULTIER M, HAPIOT P. Specific effects of room-temperature ionic liquids on cleavage reactivity: Example of the carbon-halogen bond breaking in aromatic radical anions [J]. J Phys Chem A, 2004, 108: 6175-6180.
[22] LEE C, YANG W, PARR R G. Development of the Colle-Salvetti correlation energy formula into a functional of the electron density [J]. Phys Rev B, 1988, 37: 785-792.
[23] FRISCH M J, POPLE J A, BINKLEY J S. Self-consistent molecular orbital methods 25. Supplementary functions for gaussian basis sets [J]. J Chem Phys, 1984, 80: 3265-3280.
[24] WILKES JS, HUSSEY C L. Selection of cations for ambient temperature Choolmnt molten orbital calculations [R]. FRANK J. Seiler Research Laborath, FJSRL-TR-82-0002, 1982.
Foundation item: Projects(50564006, 50904031) supported by the National Natural Science Foundation of China; Projects(2005E0004Z, 2008E0049M) supported by the Natural Science Foundation of Yunnan Province, China; Project(07Z40082) supported by the Science Foundation of the Education Department of Yunnan Province, China; Project(2007-16) supported by the Science Foundation of Kunming University of Science and Technology, China
Corresponding author: TIAN Guo-cai; Tel: +86-871-5162008; E-mail: tiangc@iccas.ac.cn; tiangc@kmust.edu.cn
DOI: 10.1016/S1003-6326(09)60082-0
(Edited by YANG Bing)