
Chromium accumulation, microorganism population and
enzyme activities in soils around chromium-containing slag heap of
steel alloy factory
HUANG Shun-hong(黄顺红), PENG Bing(彭 兵), YANG Zhi-hui(杨志辉),
CHAI Li-yuan(柴立元), ZHOU Li-cheng(周理程)
School of Metallurgical Science and Engineering, Central South University, Changsha 410083, China
Received 15 January 2008; accepted 15 July 2008
Abstract: The environmental risk of chromium pollution is pronounced in soils adjacent to chromate industry. It is important to investigate the functioning of soil microorganisms in ecosystems exposed to long-term contamination by chromium. 45 soil samples obtained from different places of the slag heap in a steel alloy factory were analyzed for chromium contamination level and its effect on soil microorganisms and enzyme activities. The results show that the average concentrations of total Cr in the soil under the slag heap, adjacent to the slag heap and outside the factory exceed the threshold of Secondary Environmental Quality Standard for Soil in China by 354%, 540% and 184%, respectively, and are 15, 21 and 9 times higher than the local background value, respectively. Elevated chromium loadings result in changes in the activity of the soil microbe, as indicated by the negative correlations between soil microbial population and chromium contents. Dehydrogenase activity is greatly depressed by chromium in the soil. The results imply that dehydrogenase activity can be used as an indicator for the chromium pollution level in the area of the steel alloy factory.
Key words: chromium contamination; soil microorganism; enzymes activities; total Cr; water soluble Cr(Ⅵ)
1 Introduction
In recent years, contamination of the environment by Cr, especially hexavalent Cr, has become a major area of concern. Chromium is the 10th abundant element in the earth’s mantle and persists in the environment as either Cr(Ⅲ) or Cr(Ⅵ). Cr(Ⅵ) is toxic to both plants and animals, being a strong oxidizing agent, corrosive, and potential carcinogen[1-2]. In contrast, the trivalent species is not toxic to plants and necessary in animal nutrition. Elevated soil concentrations of this potentially harmful element principally result from industrial wastes or spills, especially chromium-containing slag. Ore refining, production of steel and alloys, pigment manufacture, corrosion protection, leather tanning, wood preservation, and combustion of coal and oil are mostly responsible for release of chromium to the environment. Lacking of appropriate disposal facilities leads to serious groundwater and soil pollution. The steel alloy industry is a large contributor of Cr pollution to soil resources. Chromium-containing slag is one of the most hazardous solid wastes because of its high content of dissolvable Cr(Ⅵ). The accumulated chromium in soil can also cause acute and long term toxic effects on soil ecosystems[3].
Microbes play significant roles in recycling plant nutrients, maintenance of soil structure, detoxification of noxious chemicals, and the control of plant pests and plant alterations. Heavy metals exhibit toxic effects on soil biota, and they can affect key microbial processes and decrease the number and activity of soil microorganisms[4]. Microbial population has often been proposed to be an easy and sensitive indicator of anthropogenic effects on soil ecology. Cr(Ⅵ) has been reported to cause shifts in the composition of soil microbial populations, and known to cause detrimental effects on microbial cell metabolism at high concentrations[5].
Soil enzymes are synthesized by microorganisms and act as biological catalysts to facilitate different reactions and metabolic processes to decompose organic pollutants. They are also intimately involved in the cycling of nutrients, affecting on the fertilizer efficiency. Furthermore, they reflect the microbiological activity in soil and act as indicators of soil change[6-7]. Soil enzyme assays have been considered as one of the cheapest and easiest techniques and used as sensors for measuring the degree of soil pollution. Soil enzyme inhibition depends on the nature and concentration of heavy metals, Heavy metals can inhibit enzymatic activities by interacting with the enzyme substrate complexes, denaturing the enzyme protein and interacting with its active sites. Metals indirectly affect soil enzymatic activities by altering the microbial community which synthesizes enzymes[8].
Quite a few studies on soil contamination of heavy metal from industrial sites were reported[9-10], however, no literature was found to investigate the degree of chromium contamination in soils immediately under the chromium-containing slag heap and its effects on soil microorganisms and soil enzymes. This study was undertaken to assess the transverse enrichment levels of total Cr and water soluble Cr(Ⅵ) in soil environment and the effects of chromium on soil biota and enzyme activities. The field investigation was carried out at chromium-containing slag sites in a iron alloy factory in Hunan Province, China. The selected enzymes included soil catalase, dhydrogenase and polyphenol oxidase and alkaline phosphatases.
2 Experimental
2.1 Soil sampling
45 soil samples were collected from the upper 20 cm of the four selected sites, namely, three from location A (under the chromium-containing slag heap), steel alloy factory in China, nine from location B (adjacent to the slag heap), twenty-three from location C (in the vicinity of factory) and ten from control site (far away from chromate slag site), respectively. Random soil samples were collected at each sampling site, placed in sealed plastic bags and transported to the laboratory. The moist soil was divided into two portions. One portion was kept in a refrigerator and temperature was maintained at 4 ℃ for determining soil enzymes and microorganisms. Another portion was air-dried, ground and sieved through a 0.16 mm mesh sieve for determining total chromium and water soluble Cr(Ⅵ).
2.2 Soil analytical methods
Total chromium was determined using the flame atomic absorption spectrometry(FAAS) method. 1 g of soil sample was digested with 10 mL of nitric acid + 5 mL of 1?1 sulfuric acid + 5 mL of hydrofluoric acid. The total chromium in the digested solution was determined by atom absorption spectrometry. Water soluble Cr(Ⅵ) was determined by adding 50 mL of deionized water to 10 g soil samples and shaking for 1 h. The amounts of water soluble Cr(Ⅵ) in the soil extracts were determined using 1, 5-Diphenylcarbohydrazide spectrophotometric method.
All the samples were analyzed in three replicates. The quality control of analytical accuracy was also analyzed by reagent blanks and reference (China Standard ESS-3).
2.3 Microbial population
10 g of soil was diluted in 90 mL of Na4P2O7 (1 g/L, pH 7.0). Soil suspensions were shaken at 120 r/min for 30 min and then rest for 10 min to allow settling of the soil. Standard serial dilutions followed and 0.1 mL aliquots of dilution were spread on plates. Enumeration of soil microorganisms was performed using the spread plate counting method. Nutrient agar (NA) medium was explored for bacterial enumeration, while potato dextrose agar(PDA) medium and ammonium chloride-starch agar were used for fungal and actinomycetes enumeration, respectively. Streptomycin and penicillin (25 mg/mL each) were added to PDA. The microbial populations were enumerated as colony-forming units(CFU) from a serial dilution of the soil suspensions. The colonies were counted after incubation for 3, 3 and 5 d at 30 ℃ for bacteria, fungi and actinomycetes, respectively.
2.4 Soil enzyme activity
The activities of soil enzymes were assayed according to the procedures of GUAN et al[11].
Catalase activity in soils was measured using the KMnO4 titrimetric method. In brief, 5.0 g fresh soil was placed in 100 mL measuring flask and added with 0.5 mL of toluene, shaken thoroughly, and then placed in refrigerator (4 ℃) for 30 min. 25 mL of 3% H2O2 solution was added into flask which was placed in refrigerator (4 ℃) for another 30 min. 25 mL of 1 mol/L H2SO4 was mixed and the suspension was filtered. 2 mL filtrate was treated with 4 mL of 0.5 mol/L H2SO4 .The residual H2O2 was determined by titration with 0.1 mol/L KMnO4 at the presence of H2SO4. A control without soil was treated identically at the same time. Catalase activity is expressed in mL/(g?h).
Dehydrogenase activity was determined with the following modification. 2 g of soil was placed in 18 mm× 20 mm glass tube and incubated with 2 mL of 3% 2, 3, 5 tri-phenyl tetrazolium chloride(TTC) for 24 h at 30 ℃. After incubation, 10 mL acetone was added, and the suspension was homogenized with intermittent agitation for 2 h (once every half an hour) and then filtered in darkness. Reactive products were measured at 485 nm using a spectrophotometer (VIS-7220). A sample without soil containing 2 mL buffer, which is instead of TTC, was used as a control. Dehydrogenase activity is expressed in mg/(kg?d).
Polyphenol oxidase activity was measured by UV–Visible spectrophotometer. Briefly, 1 g fresh soil samples were carefully transferred into a 18 mm×20 mm glass tube and mixed with 10 mL of 1% pyrogallol solution. After incubation at 30 ℃ for 1 h, 12.5 mL of 0.5 mol/L HCl was added to the mixture, then shaken manually. The gallic acid produced was extracted with 10 mL ether and measured spectrophotometrically at 430 nm. Activity was quantified by reference to a calibration curve constructed using gallic acid standards, and it is expressed in mg/(h?g) gallic acid.
Alkaline phosphatase activity was determined by the following procedure. 1.0 g of fresh soil was mixed with 0.2 mL of toluene, 4 mL of modified universal buffers(MUB) of pH 11.0 and 1.0 mL of 0.025 mol/L p- nitrophenol phosphate and incubated for 1 h at 37 ℃. After incubation, the mixture was mixed well with 1.0 mL of 0.5 mol/L CaCl2 and 4.0 mL of 0.5 mol/L NaOH and then filtered through a filter paper. The concentration of product (p-nitrophenol) in the filtrate was determined colorimetrically at 400 nm. Activity was quantified by reference to a calibration curve constructed using Phenol standards under the same conditions described above, and it is expressed in mg/(g?h).
2.5 Statistical analysis
An analysis of variance was carried out by the General Linear Model(GLM) procedure of the SAS software package (SAS, 2001). Significant differences microbial population between means for different treatments were compared by means of the LSD test at ρ<0.05.
3 Results and discussion
3.1 Total Cr concentration in tested soil
Soil samples were collected from the upper 20 cm of four locations (A, B and C and control site) for total Cr. The total Cr concentrations in the soils of the study areas except for control site are generally high. The concentrations of total Cr concentration in the soil samples vary significantly (Table 1). The concentration of total Cr in soil varies greatly according to the distance from the chromium slag heap. The total Cr concentration ranges from 1 248.4 to 2 130.3 mg/kg (mean value 1 589.3 mg/kg) at location A. The total Cr concentration is in the range of 656.1-3 500.1 mg/kg, with the mean value of 2 239.5 mg/kg at location B. The mean concentration of total Cr is found to be 995.3 mg/kg in the soil at location C (208.6-6 207.6 mg/kg). The lowest total Cr concentration (208.6 mg/kg) is found in the soil sample far away from the main source of metal chromium such as chromium slag heap.
In comparison with local background values (Table 1) in the soils at the control site, the mean value of total Cr in the analyzed soils is much higher. The concentrations of total Cr in the soils at locations A, B and C are 15, 21 and 9 times higher than the local background value at the control site, respectively. In comparison with the threshold of Secondary Environmental Quality Standard for Soil in China (total Cr≤350 mg/kg) (National Environmental Protection Agency of China, 1995, which applies to soils in farmland, orchards and rangeland), concentrations of total Cr in the soils at locations A, B and C exceed the standard limit by 354%, 540% and 184%, respectively. Total Cr concentrations in all the samples at locations A and B exceed the standard limit. Among the 23 samples at location C, the content of total Cr in 22(95%) samples exceeds the standard limit and the highest value is 18 times of the standard limit. The results show that there are serious pollutions by chromium from the chromium-containing slag in the study areas. On the selected three locations, location B adjacent to the slag heap has the highest contaminant levels, followed by location A (directly under the slag heap), and location C has the lowest contaminant level.
Table 1 Content of total Cr and water soluble Cr in tested soils at four sites (mg/kg)
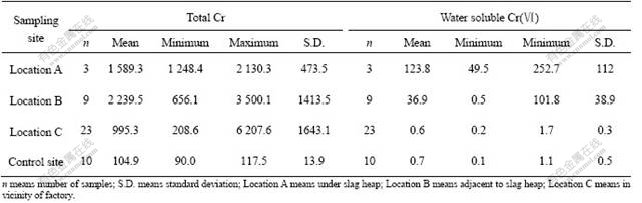
Most researches focus on the comparison of the contamination degree among the soils around the contaminant sources and coincident results that total Cr concentrations decrease with distance from the contaminating sources were reported in previous literatures[12-13], which was in agreement with our study. Opposite observation that no direct relationship was apparent between the soil chromium content and the distance from the slag heap was also reported by LOYAUX et al[14]. To our knowledge, however, no literature was found to investigate the contaminant level directly under the slag heap due to the difficulty of sampling. In this study, total Cr concentration in soils under the slag heap (location A) was slightly lower than that at location B (around the slag heap). This is due to the total Cr in surface soils at location A mainly from natural leaching and mobility from the chromium- containing slag, however, total Cr enriching in the topsoil at location B can be attributed to atmospheric fallout, leaching and diffusing when transporting the slag.
Generally, high Cr load of soils may increase absorption by plants and cause adverse effects on ecosystems, animal and human health if it consumed through foodstuff[15]. Because soil samples from location C were paddy soils, it is reasonable to assume that the large amount of total Cr in this farmland is likely to lead to food chain contamination. These facts revealed that the metal chromium discharged from the steel alloy factory posed a significant risk to soil environment.
3.2 Water soluble Cr (Ⅵ) concentration in tested soils
Water soluble Cr(Ⅵ) contents are also listed in Table 1. The highest water soluble Cr(Ⅵ) concentration is observed in soil at location A, with the mean value of 252.7 mg/kg. The lowest content in soil at location A also reaches 49.5 mg/kg. Average soluble Cr(Ⅵ) concentration is 123.8 mg/kg in the soils at location A. The average water soluble Cr(Ⅵ) in the soils at location B is 101.8 mg/kg, ranging from 0.5 mg/kg to 36.9 mg/kg. Water soluble Cr(Ⅵ) contents in the soils at location C are all low, close to local background value. However, the concentrations of water soluble Cr(Ⅵ) at locations A and B are 175 times and 52 times higher than the local background value, respectively. The results further show that the areas inside the steel alloy factory are heavily polluted by chromium from the chromium- containing slag heap. Moreover, it has been noted that the concentration of water soluble Cr(Ⅵ) is the highest in the soils directly under the slag heap. Degree of contamination in soils outside the steel alloy factory is relatively lower than that inside the steel alloy factory.
Chromium compounds are highly toxic to plants and are detrimental to their growth and development, and Cr(Ⅵ) is considered the most toxic form of Cr[16] because of its strong oxidation with a high redox potential in the range of 1.33-1.38 eV accounting for a rapid and high generation of ROS[17]. Furthermore, water soluble Cr(Ⅵ) is extremely irritating and toxic to human body tissue owing to its oxidizing potential and easy permeation of biological membranes[18]. Although the water soluble Cr(Ⅵ) content in the soil at location C is low, the total Cr concentration is high. Cr(Ⅲ) can be converted to Cr(Ⅵ) in the presence of MnO2 and molecular oxygen in soils[19]. Other forms of Cr(Ⅵ) such as exchangeable-Cr(Ⅵ), carbonate-bonded Cr(Ⅵ) can also be transformed to water soluble Cr(Ⅵ) when soil characteristics (pH, organic matter and so on) changed[20]. It should be pointed out here that low concentration of water soluble Cr(Ⅵ) in soils at location C may still pose potential health risks.
3.3 Population of microorganisms
The population of microorganisms in soil from location A (under the slag heap) is very low, only 12.053×103 CFU/g (Fig.1). However, microbial number is great in soil from control site, with the value of 19 787×103 CFU/g. Microbial number decreases by as much as three orders of magnitude, and also reveals that microbial number has been severely depressed in the heavy contaminated site. Furthermore, with the increase of distance from the chromium-containing slag heap, the number of soil microbes increases. Population of soil microorganisms shows the order of control site>location C>location B>location A. No significant difference in microbial population was found between location C and control site. The number of micro- organisms in soil from location A shows significant difference from that of the other three sites. The statistical analysis also shows that microbial number is negatively correlated with total Cr and water soluble Cr(Ⅵ) (data not shown). This suggests that the very high chromium concentrations in the contaminated sites have been primarily responsible for the decrease in the microbial number.
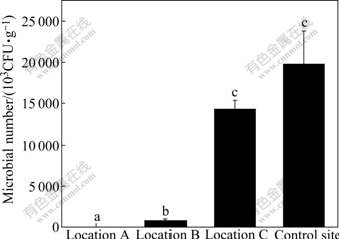
Fig.1 Total microbial population at four sampling sites (Vertical bars are SEs. Row number followed by different letters is significantly different at p=0.05. Location A (n=3): under slag heap; Location B (n=9): adjacent to slag heap; Location C (n=23): in vicinity of factory; Control site (n=10): far from factory)
3.4 Catalase activity
Catalases scavenge H2O2 and protect cells from damage are caused by reactive oxygen species. Catalase is related to active soil biomass and has been suggested as sensitive indicators of soil quality. The mean value of catalase activity is the highest at location A, a heavily contaminated site (Fig.2).
Significant differences in catalase activity were found between location A and other sites. The mean values of catalase activity in soil samples at location B, location C and control site are very near. No significant difference is found in catalase activity among these three different sampling sites (Fig.2). These facts show that catalase activity does not increase with the distance from the chromium-containing slag heap. Positive and significantly positive correlations between catalase activity and total Cr and water soluble Cr respectively indicate that heavy chromium stimulates catalase activity (Table 2). Adequate studies on the effect of heavy metal on catalase activity in soil were found and different results and even contrary findings were set in previous literatures. It was reported by BELYAEVA et al[8] that catalase activity was not markedly inhibited by heavy metals. Contrast results were elucidated by KHAN et al[21] who noted that heavy metals Cd and Pb slightly inhibited the catalase activity and the extent of inhibition increased significantly with increasing level of heavy metals.
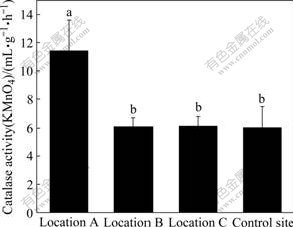
Fig.2 Catalase activities in soils at four sampling sites (Vertical bars are SEs. Row number followed by same letter is not significantly different at p=0.05. Location A (n=3): under slag heap; Location B (n=9): adjacent to slag heap; Location C (n=23): in vicinity of factory; Control site (n=10): far from factory)
The observed inhibition or activation of catalase in the soil in the presence of heavy metal probably is a result of the reaction between the metal ion in soil and the functional groups of catalase. The enhancement effect of heavy metal on catalase in this study might also be explained by the fact that catalase may be metallo- enzymes[22].
3.5 Dehydrogenase activity
Dehydrogenase activities in the soils from four sites are shown in Fig.3 and Table 3. The highest dehydrogenase activity was observed in location C, reaching 312.875 mg/(kg?d). The lowest value of dehydrogenase activity was found in location B, a site polluted severely with chromium, only 26.233 mg/(kg?d). The mean value of dehydrogenase activity of soils from four different sampling sites presents the order of location C>control site>location A>location B (Fig.3). No significant difference was found in dehydrogenase activity between location A and location B, between location C and control site, however, dehydrogenase activities in the soils of location A and location B significantly differed with location C and control site. The results show that heavy metal chromium inhibited dehydrogenase activity and soil health is impacted markedly by chromium from chromium slag. Negative correlation between dehydrogenase activity and water soluble Cr(Ⅵ) (Table 2) shows that water soluble Cr(Ⅵ) is responsible for the inhibition of dehydrogenase activity. This is associated with the inhibition of microbial growth as dehydrogenase is an intracellular enzyme[23]. Inhibition of higher heavy metal concentration on dehydrogenase might be due to reduced microbial number and activity in soils because the activity is derived from the intracellular enzyme. The results illustrate that dehydrogenase activity is one of the best indicators of microbial activity because dehydrogenase only occurs within living cells, which can reflect the ability of microorganism taking part in redox reaction in soil. Dehydrogenase activity is very sensitive to chromium contamination.
Table 2 Correlation coefficient (r) among contents of total Cr, water soluble Cr and enzymatic activities in tested soils at four sites (n=45)
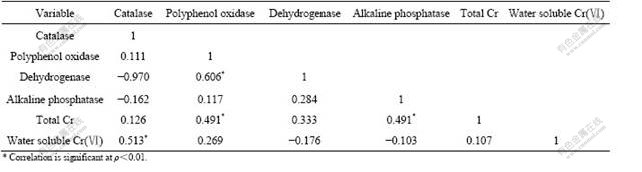
Table 3 Range of enzymatic activities in tested soils at four sites
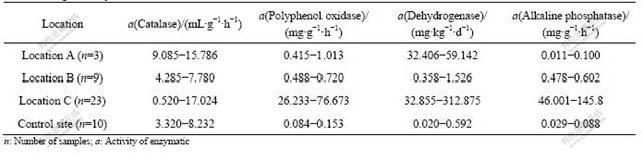
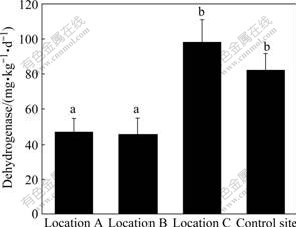
Fig.3 Dehydrogenase activities in soils at four sampling sites (Vertical bars are SEs; Row number followed by same letter is not significantly different at p=0.05; Location A (n=3): immediately under slag heap; Location B (n=9): adjacent to slag heap; Location C (n=23): in vicinity of factory; Control site (n=10): far from factory)
3.5 Polyphenol oxidase activity
Polyphenol oxidase activities of soils from four sampling sites are exhibited in Table 3 and Fig.4. The highest and lowest values of polyphenol oxidase activity were all found in location C. The mean value of polyphenol oxidase activity in the soils of the four locations is very near, presenting no significant differences. Moreover, positive correlation between polyphenol oxidase activity and chromium content was observed. This indicates that chromium does not influence polyphenol oxidase activity.
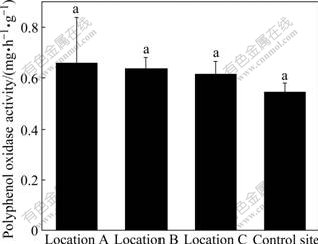
Fig.4 Polyphenol oxidase activities in soils at four sampling sites (Vertical bars are SEs; Row number followed by same letter is not significantly different at p=0.05; Location A (n=3): immediately under slag heap; Location B (n=9): adjacent to slag heap; Location C (n=23): in vicinity of factory; Control site (n=10): far from factory)
3.6 Alkaline phosphatase activity
Alkaline phosphatase catalyses the hydrolysis of various organic phosphorus compounds and acts as transphosphorylases at alkaline pH[24]. Activity of alkaline phosphatase in the soils varies with the distance from chromium slag sites (Table 3 and Fig.5). Alkaline phosphatase activity reaches the highest levels (0.592 mg/(g?h), 37 ℃) in soils at location C and the lowest (0.011 mg/(g?h). 37 ℃) at location A (Table 3). The order of the mean value of alkaline phosphatase activity is location C>location B>location A>control site. Alkaline phosphatase activity in soils does not significantly differ among the four sampling sites. This shows that the distance from the chromium slag heap does not significantly affect alkaline phosphatase activity. Significantly positive correlation was observed between alkaline phosphatase activity and total Cr (Table 2), indicating that total Cr stimulates alkaline phosphatase activity. However, weak negative correlation between alkaline phosphatase activity and water soluble Cr(Ⅵ) shows that mobile Cr(Ⅵ) slightly inhibits alkaline phosphatase activity.
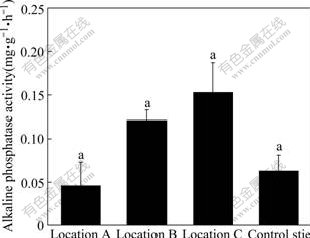
Fig.5 Alkaline phosphatase activity in soils at four sampling sites (Vertical bars are SEs; Row number followed by same letter is not significantly different at p=0.05; Location A (n=3): immediately under slag heap; Location B (n=9): adjacent to slag heap; Location C (n=23): in vicinity of factory; Control site (n=10): far from factory)
4 Conclusions
1) Soils adjacent to the slag heap has the highest mean total Cr concentration, reaching 2 239.5 mg/kg, exceeding the threshold of Secondary Environmental Quality Standard for Soil in China by 540% and being 21 times higher than local background value. Under the slag heap the average concentration of total Cr reaches 1 589.3 mg/kg, 15 and 4 times higher than the standard limit and local background value, respectively. Even outside the factory the chromium concentration in soil is as high as 959.3 mg/kg, 9 times higher than local background value. Average concentrations of water soluble Cr(Ⅵ) in the tested soils under the slag heap and adjacent to the slag heap are 175 times and 52 times higher than the background values, respectively. However, the contents in the vicinity of the factory are all low, close to local background value.
2) The chromium slag heap from the steel alloy factory may pose a significant risk to soil environment. Microbial number and dehydrogenase activity are severely depressed by chromium from the chromium- containing slag. Chromium pollution exhibits no inhibiting effect on catalase, polyphenol oxidase and alkaline phosphatase activity. Dehydrogenase and microbial population can be used as an indicator for the chromium pollution level in the area of steel alloy factory.
References
[1] BANERJEE M, MISHRA S, CHATTERJEE J. Scavenging of nickel and chromium toxicity in Aulosira fertilissima by immobilization: Effect on nitrogen assimilating enzymes [J]. Electronic Journal of Biotechnology, 2004, 7(3): 302-309.
[2] MEGHARAJ M, AVUDAINAYAGAM S, NAIDU R. Toxicity of hexavalent chromium and its reduction by bacteria isolated from soil contaminated with tannery waste [J]. Current Microbiology, 2003, 47(1): 51-54.
[3] VITI C. Response of microbial communities to different doses of chromate in soil microcosms [J]. Applied Soil Ecology, 2006, 34(2/3): 125-139.
[4] OBBARD P. Ecotoxicological assessment of heavy metals in sewage sludge amended soils [J]. Appl Geochem, 2001, 16: 1405-1411.
[5] SHI W, BISCHOFF M, TURCO R, KONOPKA A. Long-term effects of chromium and lead upon the activity of soil microbial communities [J]. Applied Soil Ecology, 2002, 21: 169-177.
[6] DICK W A, CHENG L, WANG P. Soil acid and alkaline phosphatase activity as pH adjustment indicators [J]. Soil Biology and Biochemistry, 2000, 32(13): 1915-1919.
[7] GILLER K E, WITTER E, MCGRATH S P. Toxicity of heavy metals to microorganisms and microbial processes in agricultural soils: A review [J]. Soil Biology and Biochemistry, 1998, 30(10/11): 1389-1414.
[8] BELYAEVA O N, HAYNES R J, BIRUKOVA O A. Barley yield and soil microbial and enzyme activities as affected by contamination of two soils with lead, zinc or copper [J]. Biology and Fertility of Soils, 2005, 41(2): 85-94.
[9] AL-KHASHMAN O A, SHAWABKEH R A. Metals distribution in soils around the cement factory in southern Jordan [J]. Environmental Pollution, 2006, 140(3): 387-394.
[10] SCHULIN R. Heavy metal contamination along a soil transect in the vicinity of the iron smelter of Kremikovtzi (Bulgaria) [J]. Geoderma, 2007, 140(1/2): 52-61.
[11] GUAN Song-ying, ZHANG Deng-sheng, ZHANG Zhi-ming. Soil enzymes and its methodology [M]. Beijing: Agriculture Press, 1986: 274-338. (in Chinese)
[12] AGBENIN J O. The distribution and dynamics of chromium and nickel in cultivated and uncultivated semi-arid soils from Nigeria [J]. Science of the Total Environment, 2002, 300(1/3): 189-199.
[13] ZAGURY G J, SAMSON R, DESCHENES L. Occurrence of metals in soil and ground water near chromated copper arsenate-treated utility poles [J]. J Environ Qual, 2003, 32(2): 507-514.
[14] LOYAUX L S, LECOMTE P, EHRHARDT J J. Behavior of hexavalent chromium in a polluted groundwater: Redox processes and immobilization in soils [J]. Environ Sci Technol, 2001, 35(7): 1350-1357.
[15] OLIVER M A. Soil and human health: A review [J]. European Journal of Soil Science, 1997, 48(4): 573-592.
[16] HANKER A K. Chromium toxicity in plants [J]. Environment International, 2005, 31(5): 739-753.
[17] SHANKER A K, PATHMANABHAN G. Speciation dependant antioxidative response in roots and leaves of sorghum (Sorghum bicolor(L) Moench cv CO 27) under Cr(Ⅲ) and Cr(Ⅵ) stress [J]. Plant and Soil, 2004, 265(1): 141-151.
[18] MELAKU S, CORNELIS R,VANHAECKE F, DAMS R, MOENS L. Method development for the speciation of chromium in river and industrial wastewater using GFAAS [J]. Microchimica Acta, 2005, 150(3): 225-231.
[19] FENDORF S E, ZASOSKI R J. Chromium(Ⅲ) oxidation by δ-MnO2 (I): Characterization [J]. Environmental Science and Technology, 1992, 26(1): 79-85.
[20] KOLELI N. Speciation of chromium in 12 agricultural soils from Turkey [J]. Chemosphere, 2004, 57(10): 1473-1478.
[21] KHAN S, CAO Q, HESHAM A E L, YUE X, HE J Z. Soil enzymatic activities and microbial community structure with different application rates of Cd and Pb [J]. Journal of Environmental Sciences, 2007, 19(7): 834-840.
[22] YANG Zhi-xin, LIU Shu-qin, ZHENG Da-wei, FENG Sheng-dong. Effects of cadium, zinc and lead on soil enzyme activities [J]. Journal of Environmental Sciences, 2006, 18(6): 1135-1141.
[23] BAATHE E. Effects of heavy metals in soil microbial processes and populations (a review) [J]. Water, Air and Soil Pollution, 1989, 47(3/4): 335-379.
[24] MADEJON E, BURGOS P, LOPEZET R, CABRERA F. Soil enzymatic response to addition of heavy metals with organic residues [J]. Biology and Fertility of Soils, 2001, 34(3): 144-150.
Foundation item: Project(K0802144-31) supported by the Program of Science and Technology of Changsha, China; Projects(2006AA06Z374, 2007AA021304) supported by the National Hi-tech Research and Development Program of China
Corresponding author: YANG Zhi-hui; Tel: +86-731-8830875; E-mail: yangzhihui65@yahoo.com
DOI: 10.1016/S1003-6326(08)60259-9
(Edited by LI Xiang-qun)