J. Cent. South Univ. (2017) 24: 489-495
DOI: 10.1007/s11771-017-3451-7

Effect of ferrite content on dissolution kinetics of gibbsitic bauxite under atmospheric pressure in NaOH solution
YANG Hui-bin(杨会宾)1, 2, PAN Xiao-lin(潘晓林)1, YU Hai-yan(于海燕)1,
TU Gan-feng(涂赣峰)1, SUN Jun-min(孙俊民)2
1. School of Metallurgy, Northeastern University, Shenyang 110004, China;
2. Datang International High Aluminum Coal R&D Center
(National Energy Sources High Aluminum Coal Development and Utilization Key Laboratory), Ordos 010321, China
Central South University Press and Springer-Verlag Berlin Heidelberg 2017
Abstract: The dissolution property of high-ferrite gibbsitic bauxite and the effect of ferrite content on the dissolution kinetics of gibbsitic bauxites in sodium hydroxide solution under atmospheric pressure from 50 to 90 °C were systematically investigated. The dissolution property of high-ferrite gibbsitic bauxite is increased by increasing the dissolution temperature and the NaOH concentration or decreasing the particle size of bauxite, which is easy to dissolve under atmospheric pressure. The kinetic equations of gibbsitic bauxites with different ferrite contents during the dissolution process at different temperatures for different times were established, and the corresponding activation energies were calculated. The ferrite in the gibbsitic bauxite reduces the dissolution speed and increases the activation energy of dissolution, the diffusion process of which is the rate-controlling step.
Key words: gibbsitic bauxite; dissolution; kinetics; ferrite; Bayer process
1 Introduction
More than 90% of the alumina output is produced by the Bayer process, which is the major production technology in the alumina industry. Depending on the dissolution temperature, the Bayer process can be classified into three kinds, i.e. low-temperature digestion Bayer process, medium-temperature digestion Bayer process and high-temperature digestion Bayer process. In the industry, lower dissolution temperature would reduce the equipment specifications [1], and then the investment will be reduced. It is very important to strengthen the research on the low temperature or atmospheric pressure during the Bayer process. The selection of Bayer process depends on the kinds of bauxite. Low temperature Bayer process only can deal with gibbsitic bauxite [2-4]. Goethite and hematite are commonly found together with gibbsite in the gibbsitic bauxite, because their parent rocks of pyroxene and plagioclase are associated minerals. Ferrite is the main impurity in gibbsitic bauxite. CHEN et al [5] studied the lateritic gibbsitic bauxite and confirmed the shapes of gibbsite, goethite and hematite. Gibbsite crystal presents hexagonal-platelet morphology or flaky, goethite is kidney shape, crusty or bean oolitic and hematite presents granular aggregates. The crystal structure of goethite and hematite as well as the way mixed with gibbsite will affect the dissolution of gibbsite.
The dissolution process can be improved easily by rising digestion temperature or increasing the caustic soda concentration in the alumina industry. Little attention was paid to the dissolution step and the theory research on the gibbsite dissolution kinetics until 1970. There is no consensus about its limiting step during the dissolution process in the literatures so far. Chemical reaction was considered to be the limiting step during the dissolution of gibbsite by PEREIRA et al, GLASTONBURY (1967), ROACH (1985) and LI et al (1995) [6]. BAO et al and HERMANN (1953) found the rate-controlling step is diffusion [7]. BOMTRAGER et al (1974) and YIN (1988) considered that both the chemical reaction and diffusion are important. YIN et al (1991) reported that chemical reaction is the rate-controlling step when the temperature is under 80 °C, while the diffusion is the limiting step when the temperature is above 115 °C [7]. As shown in the literatures, most of the samples used in the kinetic experiments are aluminum hydroxide or pure gibbsite. However, the raw gibbsitic bauxite contains various impurities including goethite,hematite, quartz, kaolinite etc. Pure gibbsite and gibbsitic bauxite are different in several aspects, such as the crystal growth process, crystal structure and the doping way of impurity minerals and the gibbsite. Therefore, the dissolution property of gibbsitic bauxite in caustic solution can be affected by those differences.
The dissolution activation energy of pure gibbsite and gibbsitic bauxite is affected by the growing process of the gibbsite crystal, which is difference in the crystal structure and impurities. PEREIRA et al [6] and ADDAI-MENSAH et al [8] calculated that activation energy of the gibbsite is 110 kJ/mol and 90 kJ/mol, respectively, using Al(OH)3 as the experiment sample. BAO et al [9], YIN et al [10] and LI et al [11] figured out that the activation energy values are (75±1) kJ/mol, 76.20-76.85 kJ/mol and 76.85 kJ/mol using pure gibbsite, respectively [9-11].
The dissolution property and kinetics of gibbsitic bauxites with different ferrite contents were investigated under the atmospheric pressure in this work. The effect of ferrite content on the rate-controlling step and the kinetic equation of gibbsitic bauxite were also discussed.
2 Materials and methods
2.1 Materials
The raw gibbsitic bauxite (named Bauxite 1) used in this research was exploited from West Kalimantan of Indonesia. The bauxite samples were dried at 105 °C for 6 h, and then crushed by the jaw crusher and crushing roller. Three samples with different particle sizes were sieved by standard sieves, the average particle sizes of which are 2.00 mm, 0.75 mm and 0.20 mm (named Sample 1, Sample 2 and Sample 3). All these three samples are raw bauxite and the chemical and mineral compositions are the same. The main chemical compositions of the raw bauxite are given in Table 1. The XRD pattern of the bauxite is shown in Fig. 1, and the mineral components of bauxite are given in Table 2. As shown in Table 2, the main minerals of the bauxite are gibbsite, goethite and hematite.
Table 1 Main chemical compositions of raw bauxite (Bauxite 1) (mass fraction, %)

The samples with lower ferrite contents were obtained by the magnetic method to remove part of the ferrite. The ferrite content in the samples was controlled by adjusting the intensity of the magnetic field. First, the raw gibbsitic bauxite was ground to a particle size of 0.10-0.50 mm. Then, the magnetic selector was used to separate the high-ferrite parts and the low-ferrite parts,and the intensities of magnetic field are 0.6 and 0.9 T, respectively. Wet magnetic separation was selected, and the high-ferrite parts were discarded and the low ferrite parts were dried for dissolution experiments. Compared with the raw bauxite, the separated samples were named Bauxite 2 and Bauxite 3, which corresponds to the parts separated by 0.6 and 0.9 T, respectively. The main chemical compositions of the samples are given in Table 3.
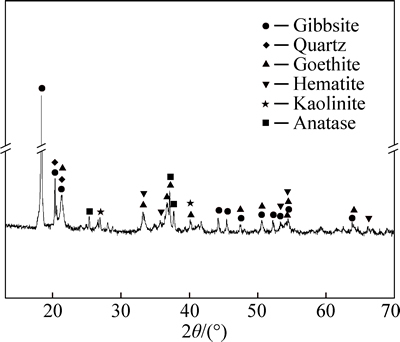
Fig. 1 XRD pattern of raw bauxite
Table 2 Main mineral compositions of raw bauxite (mass fraction, %)

Table 3 Chemical compositions of Bauxite 2 and Bauxite 3 (mass fraction, %)
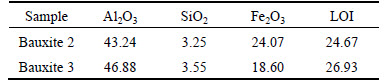
2.2 Experimental methods
The dissolution experiments were conducted in an isothermal batch reactor. The reaction temperature (T) and the sodium hydroxide concentration (CNaOH) vary from 50 °C to 90 °C and from 1 mol/L to 5 mol/L, respectively. The stirring speed (R) is between 250 and 450 r/min. The liquid-to-solid ratio for dissolution (L/S) is 5-30.
The bauxite residues were washed by hot distilled water, and then dried for the analyses of XRD. The alumina dissolution efficiency (μA) was calculated according to
μA=(cAc0V0)/(CAW0c1) (1)
where cA is the alumina concentration in sodium aluminate solution after dissolution, g/L; c0 and c1 are the NaOH concentration before and after dissolution, g/L; V0 is the volume of the initial solution, L; CA is the alumina content in the bauxite, %; W0 is the addition of the bauxite, g.
As given in Table 1, the silica content in the bauxite is relatively low, which makes the estimated error brought by the SiO2 is 0.05% for the alumina dissolution efficiency and 0.04% for the changes of caustic soda concentration. Therefore, the presence of the silica is ignored in this work.
2.3 Analysis methods
The particle size of the gibbsitic bauxite was detected by standard screens, while the bauxite residue was detected by a laser nanometer size analyzer. The chemical compositions of the bauxite were detected by national or industry standards methods. The alumina is analyzed by the EDTA titration method. The silicon oxide is analyzed by the molybdenum blue photometric method. The iron oxide is analyzed by the orthophenanthrolene spectrophotometry method and the titanium dioxide was detected by the diantipyrylmethane spectrophotometry method. The sodium oxide concentration of the solution was detected by acid-base neutralization method and the alumina concentration was detected by EDTA complexometry.
The mineral phases were analyzed by the BRUKER X-ray diffraction using Cu target cathode. The voltage is 30 kV and the current is 15 mA. The scanning angle is from 3° to 80° with the step size of 0.02°.
3 Results and discussion
3.1 Dissolution property of high-ferrite gibbsitic bauxite
The particle size of bauxite is one of the main factors to affect the alumina dissolution speed. As shown in Fig. 2, the alumina dissolution speed of Sample 3 is the fastest. The dissolution efficiency is closed to 80% when the dissolution time is 10 min. The dissolution speed of Sample 1 is the slowest and becomes more stable after 40 min. The dissolution efficiency of Sample 2 is 77.01% for 20 min, which is between that of Sample 1 and Sample 3. The dissolution efficiencies of the three samples increase slowly after 20 min. The dissolution efficiencies of Sample 1 and Sample 2 have little difference when the dissolution time is 40 min, which are 82.26% and 81.40%, respectively. The dissolution efficiency of the Sample 3 is higher than that of the other samples obviously. When the bauxite particles are fully ground, the gibbsite crystal are fully exposed, which increases the contact area in caustic solution. Meanwhile, fully grinding also makes large damage to the crystal surfaces, which enhances the reactivity of the bauxite particle and increases the gibbsite dissolution in caustic solution.
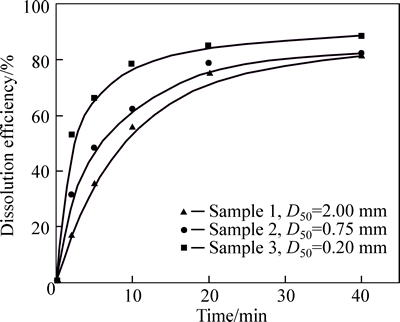
Fig. 2 Dissolution efficiencies of samples with different particle sizes (T=85 °C; L/S=10; CNaOH=3 mol/L; R=350 r/min)
The dissolution results of Sample 2 with different stirring speeds are shown in Fig. 3. As shown in Fig. 3, the alumina dissolution efficiency is affected by the stirring speed obviously. The dissolution speed is increased with increasing the stirring speed, which indicates that diffusion may affect the dissolution speed. The relative motion of the particles and liquid is increased as the stirring speed increases, which enhances the diffusion velocity of the liquid reactants and products. But the stirring speed does not affect the shape of the dissolution efficiency curves. All the curves are similar to the parabola shape.
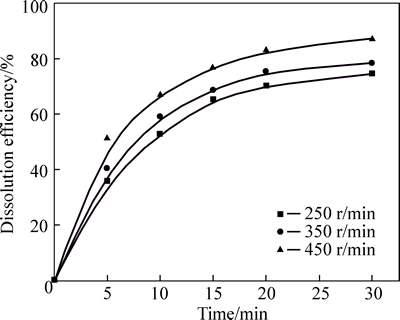
Fig. 3 Dissolution efficiencies of Sample 2 with different stirring speeds (T=85 °C; L/S=10; CNaOH=3 mol/L)
During dissolution process, the liquid-to-solid ratio is very important. The higher the liquid-to-solid ratio is, the higher the αk is, which makes the driving force of the dissolution stronger and the dissolution speed faster. The dissolution efficiencies of Sample 2 under different liquid-to-solid ratios are shown in Fig. 4. As shown in Fig. 4, the alumina dissolution efficiency is the lowest when the liquid-to-solid ratio is 5, which is only 67.00% for 30 min. The increase of the dissolution efficiency is obvious when the liquid-to-solid ratio increases to 10,which increases by 18.40% compared with the liquid-to- solid of 5 for the same dissolution time, but it does not change much when the liquid-to-solid ratio is over 10.
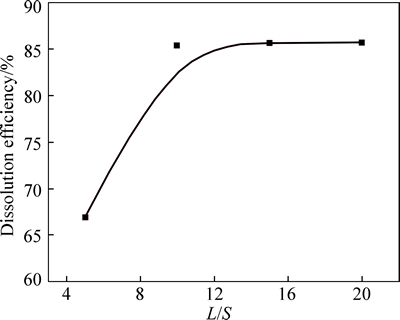
Fig. 4 Dissolution efficiencies of Sample 2 with different liquid-to-solid ratios (T=85 °C; R=350 r/min; CNaOH=3 mol/L; t=30 min)
The NaOH concentration is one of the most important factors during the dissolution process. The dissolution results of various NaOH concentrations are shown in Fig. 5. As shown in Fig. 5, the alumina dissolution efficiency is low when the NaOH concentration is low, which is only 44.40% for 60 min when the NaOH concentration is 1 mol/L. The alumina dissolution efficiency increases with the increase of the NaOH concentration and higher NaOH concentration shortens the dissolution time. The dissolution curves of NaOH concentrations with 3-5 mol/L are very close, indicating that the proper NaOH concentration is 3 mol/L.
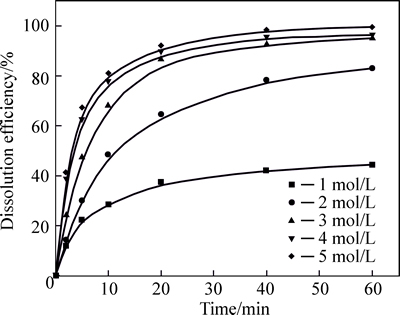
Fig. 5 Dissolution efficiencies of Sample 2 with different NaOH concentrations (T=90 °C; L/S=10; R=350 r/min)
The results of different dissolution temperatures are shown in Fig. 6. As shown in Fig. 6, the dissolution temperature affects the dissolution efficiency and dissolution speed obviously. The alumina dissolution efficiency increases slowly when the temperature is below 70 °C, which is less than 72.00% for 40 min. The gibbsite dissolution speed is faster when the temperature is above 80 °C. The alumina dissolution efficiency is higher than 90% at 90 °C for 20 min, and it does not increase with the dissolution time, indicating that the dissolution process has almost finished when the dissolution time is 20 min.
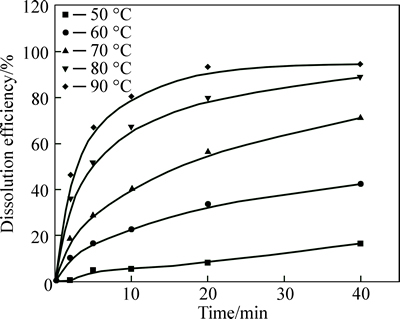
Fig. 6 Dissolution efficiencies of Sample 2 with different temperatures (CNaOH=3 mol/L; L/S=10; R=350 r/min)
3.2 Dissolution kinetics of high-ferrite gibbsitic bauxite
The pure gibbsite dissolving in the caustic solution is simple liquid-solid reaction and no new solid product appears during the dissolution process, which accords to the shrinking nonporous models [12]. The chemical reaction is given as
Al2O3·3H2O(s)+2OH-(aq)=
(aq) (2)
The digestion process of the gibbsite involves basically three steps. The first step corresponds to the diffusion of liquid reactants towards the liquid-solid reaction surface, which is external diffusion. Next step is the reaction at the interface. The final step is the diffusion of products from the interface towards the liquid, which is internal diffusion. When the limiting step is chemical reaction or external diffusion, the equation of the dissolution efficiency is calculated by [12-15]
1-(1-α)1/3=kt (3)
where α is the dissolution efficiency of alumina, k is the apparent efficiency constant and t is the dissolution time.
The gibbsitic bauxite is not a pure crystal, which contains impurity minerals such as goethite, hematite, quartz and kaolinite. The gibbsite dissolution process may be influenced by the impurity minerals, resulting in the change of the dissolution equation. When diffusion is the limiting step, the dissolution equation is as shown as [12, 13]
1-2α/3-(1-α)2/3=kt (4)
where α is the dissolution efficiency of alumina, k is the apparent efficiency constant and t is the dissolution time.
The curves of dissolution efficiency with dissolution time according to Eqs. (3) and (4) are shown in Fig. 7. The linear relationship between 1-2α/3- (1-α)2/3 and t are much better compared with that of 1-(1-α)1/3 and t. The values of R2 (the correlation coefficient) shown in Fig. 7(b) are higher than those in Fig. 7(a). This suggests that the alumina dissolution efficiency is affected by the impurity minerals attached on the gibbsite crystals and the diffusion is the rate- controlling step.
The slopes of k, the apparent rate constants of the straight lines in Fig. 7(b), are calculated, as given in Table 4.
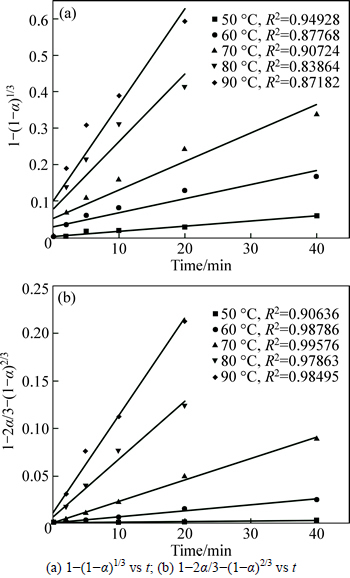
Fig. 7 Relation curves of dissolution efficiencies with time for Sample 2:
Table 4 Values of k of bauxite

The Arrhenius equation is shown as Eq. (5) and the Arrhenius curve of the dissolution process is shown in Fig. 8. The slop of the straight line (k′) is -14.13:
lnk=-E/(RT)+lnA (5)
where k is the efficiency constant, E is the activation energy and A is the preexponential factor or frequency factor.
The activation energy was calculated to be 117.485 kJ/mol according to the Eq. (6), which is closeto the data calculated by PEREIRA et al [6]
E=-k'×R (6)
According to the Arrhenius equation, A can be figured out, which is 1.32×1015 min-1. The Equation of rate constants and dissolution efficiency equation can be obtained:
k=1.32×1015exp[-117485/(RT)] (7)
1-2α/3-(1-α)2/3=1.32×1015exp[-117485/(RT)]t (8)
The rate equation suggests that the limiting step is diffusion. It is because the impurity minerals affect the dissolution process obviously. The impurity minerals mix with or wrap the gibbsite crystal, which prevent the diffusion of the products and reduce the gibbsite dissolution speed. The limiting step is internal diffusion.
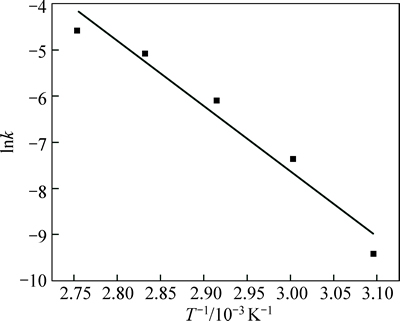
Fig. 8 Arrhenius curve of dissolution for Sample 2
3.3 Effects of ferrite content on dissolution kinetics
Goethite and hematite are the main impurities in the gibbsitic bauxite. The two kinds of ferrite-bearing mineral can influence the dissolution process of the gibbsite [16, 17].
Bauxite 2 and Bauxite 3 have the lower ferrite content, as given in Table 3. The dissolution tests of Bauxite 2 and Bauxite 3 were carried out at different temperatures varying from 50 °C to 90 °C. The liquid-to- solid ratio is 10 and the NaOH concentration is 3 mol/L. The dissolution efficiency curves of the Bauxite 2 and Bauxite 3 are shown in Fig. 9.
The dissolution equations are selected by the same method as that of Sample 2. The two relationship curves of the equation 1-2α/3-(1-α)2/3 and t can be obtained. The relationship curves are shown in Fig. 10 and the corresponding Arrhenius figures are shown in Fig. 11. The diffusion is also the limiting step, which indicates that the rest ferrites in the bauxite still influence the dissolution process.
The apparent activation energies of Bauxite 2 and Bauxite 3 were calculated to be 99.14 kJ/mol and 98.93 kJ/mol, respectively. The corresponding preexponential factors A are 2.42×1012 min-1 and 1.75×1012 min-1. The dissolution efficiency equations of Bauxite 2 and Bauxite 3 were obtained:
1-2α/3-(1-α)2/3=2.42×1012exp[-99144/(RT)]t (9)
1-2α/3-(1-α)2/3=1.75×1012exp[-98928/(RT)]t (10)
By comparing Eq. (8) with Eqs. (9) and (10), the activation energy and the corresponding preexponential factors during dissolution decrease with decreasing the ferrite content. The correlation coefficients of main chemical compositions and the activation energies were calculated by the Excel function (CORREL), as given in Table 5. CE-S, CE-F and CE-A are the correlation coefficients of silica content, ferrite content and alumina content with the activation energy, respectively.
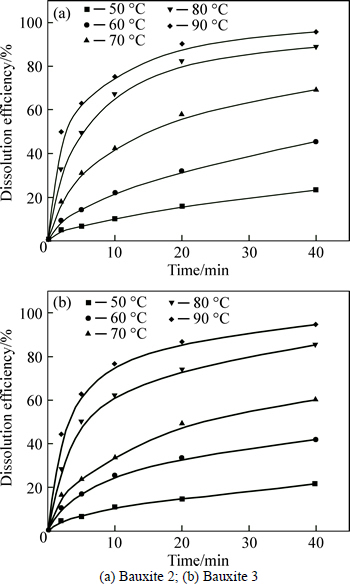
Fig. 9 Dissolution efficiencies of Bauxite 2 and Bauxite 3 at different dissolution temperatures (R=350 r/min):
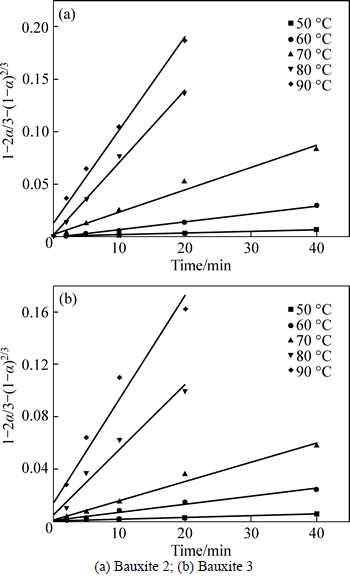
Fig. 10 Relation curves of dissolution efficiency vs time for Bauxite 2 and Bauxite 3:
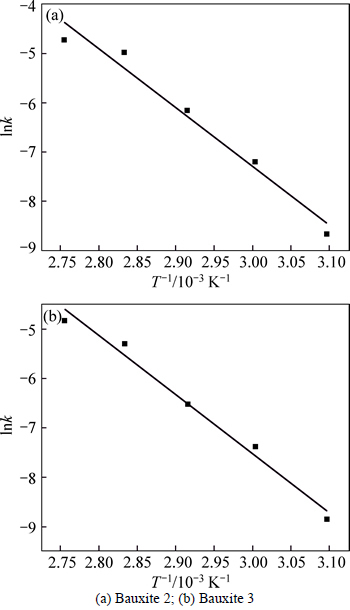
Fig. 11 Arrhenius curves of Bauxite 2 and Bauxite 3:
As given in Table 5, the value of CE-F is 0.90, which is the biggest correlation coefficient. This indicates that the ferrite content is the main reason for the change of activation energy. The activation energy decreases when the ferrite content decreases, which benefits to thedissolution process and makes the gibbsite dissolution easier. ADDAI-MENSAH et al [8] estimated that the value of activation energy is 90 kJ/mol, which is close to 98.93 kJ/mol (Bauxite 3, ferrite content is relatively low). In addition, BAO et al [9], YIN et al [10] and LI et al [11] calculated the activation energy between 74.00 kJ/mol and 76.85 kJ/mol using pure gibbsite. This also suggests that the less the impurity minerals in the gibbsitic bauxite are, the less the value of activation energy is. For the Bauxite 1, the activation energy is comparatively higher because of the more goethite and hematite. The dissolution performance of the gibbsitic bauxite is improved when some ferrite is removed.
Table 5 Correlation coefficients of main chemical compositions vs activation energies

The effect of ferrite content on the dissolution kinetics is only a surface phenomenon, and it actually reflects the mix or wrap status of gibbsite and ferrite in the gibbsitic bauxite. The mix or wrap status of them is higher while the ferrite content is higher, so the dissolution activation energy of gibbsite is higher and the dissolution is difficult. While partial ferrite is removed, the mix or wrap status of them is reduced and the dissolution activation energy correspondingly becomes smaller. But, the mix or wrap status of the gibbsite and ferrite do not disappear completely in the three kinds of gibbsitic bauxite, so the dissolution rate equation types of them are the same.
4 Conclusions
1) The gibbsite dissolution process of the high- ferrite gibbsitic bauxite is influenced by the impurity minerals, which prevents the diffusion of products and reduces the gibbsite dissolution speed. Diffusion is the limiting step during the dissolution process. Increasing dissolution temperature and NaOH concentration or decreasing the bauxite particle size increases the dissolution speed of bauxite. The dissolution efficiency of gibbsite is over 90% for 20 min under atmospheric pressure.
2) The kinetic equations of Bauxite 1, Bauxite 2 and Bauxite 3 with different ferrite contents were established as 1-2α/3-(1-α)2/3=1.32×1015exp[-117485/(RT)]t, 1- 2α/3-(1-α)2/3=2.42×1012exp[-99144/(RT)]t and 1-2α/3- (1-α)2/3=1.75×1012exp[-98928/ (RT)]t, respectively.
3) The activation energy and the preexponential factor of gibbsitic bauxite during the dissolution process are reduced by the ferrite. Decreasing the ferrite content reduces the activation energy and accelerates the dissolution process.
References
[1] BI Shi-wen, YU Hai-yan. Alumina production technology [M]. Beijing: Chemical Industry Press, 2006: 40-41. (in Chinese)
[2] CERTINI G, WILSON M J, HILLIER S J, FRASER A R, DELBOS E. Mineral weathering in trachydacitic-derived soils and saprolites involving formation of embryonic halloysite and gibbsite at Mt. Amiata, central Italy [J]. Geoderma, 2006, 133(3, 4): 173-190.
[3] MULYANTO B, STOOPS G, VAN R E. Precipitation and dissolution ofgibbsiteduring weathering of andesitic boulders in humid tropical West Java [J]. Geoderma, 1999, 89(3, 4): 287-305.
[4] HERRMANN L, ANONGRAK N, ZAREI M, SCHULER U, SPOHRER K. Factors and processes ofgibbsiteformation in northern Thailand [J]. Catena, 2007, 71(2): 279-291.
[5] CHEN Jian-guo, LIU Yun-hua, XU Jun-wen. Differences of mineralization of two gibbsitic bauxites in Guangxi province [J]. Earth Science Frontiers, 1999, 6(S): 251-256. (in Chinese)
[6] PEREIRA J A M, SCHWAAB M, DELL'ORO E, PINTO J C, MONTEIRO J L F, HENRIQUES C A. The kinetics ofgibbsitedissolution in NaOH [J]. Hydrometallurgy, 2009, 96(1, 2): 6-13.
[7] BAO Li, NGUYEN A V. Developing a physically consistent model forgibbsiteleaching kinetics [J]. Hydrometallurgy, 2010, 104(1): 86-98.
[8] ADDAI-MENSAH J, DAWE J, RALSTON J. The dissolution and interactions ofgibbsiteparticles in alkaline media [J]. Developments in Mineral Processing,2000, 13: C6-1-C6-7.
[9] BAO Li, ZHANG Ting-an, LIU Yan, DOU Zhi-he, LU Guo-zhi, WANG Xiao-min, MA Jia, JIANG Xiao-li. The most probable mechanism function and kinetic parameters ofgibbsitedissolution in NaOH [J]. Chinese Journal of Chemical Engineering,2010, 18(4): 630-634.
[10] YIN Ai-jun, CHEN Qi-yuan, ZHANG Ping-min. Studies on the kinetics of digestion process of synthetic gibbsite by DSC [J]. Chemical Journal of Chinese Universities, 1991, 12(11): 1507-1509. (in Chinese)
[11] LI Chao-qun, ZHANG Ping-min, CHEN Qi-yuan, CHEN Xin-min. Investigation of digestion process kinetics of gibbsite [J]. Nonferrous Metals, 1991, 43(4): 52-55. (in Chinese)
[12] HUA Yi-xin. Introduction to metallurgical process dynamics [M]. Beijing: Metallurgical Industry Press, 2004: 188-198. (in Chinese)
[13] LI Hong-gui. Hydrometallurgy [M]. Changsha: Central South University Press, 2005: 74-79. (in Chinese)
[14] LIU Zhi-xiong, YIN Zhou-lan, CHEN Yi-guang, XIONG Li-zhi. Leaching kinetics of molybdenum from Ni-Mo ore in sulfuric acid solution with sodium peroxodisulfate as oxidant [J]. Journal of Central South University, 2015, 22(3): 874-879.
[15] LIU Zhi -xiong, YIN Zhou- lan, HU Hui -ping, CHEN Qi -yuan. Leaching kinetics of low -grade copper ore with high- alkality gangues in ammonia- ammonium sulphate solution [J]. Journal of Central South University, 2012, 19(1): 77-84.
[16] WEBSTER N A S, LOAN M J, MADSEN I C, KNOTT R B, KIMPTON J A. An investigation of the mechanisms ofgoethite, hematite and magnetite-seeded Al(OH)3precipitation from synthetic Bayer liquor [J]. Hydrometallurgy, 2011, 109(1, 2): 72-79.
[17] LIU Hai-bo, CHEN Tian-hu, FROST R L. An overview of the role ofgoethitesurfaces in the environment [J]. Chemosphere, 2014, 103: 1-11.
(Edited by YANG Bing)
Cite this article as: YANG Hui-bin, PAN Xiao-lin, YU Hai-yan, TU Gan-feng, SUN Jun-min. Effect of ferrite content on dissolution kinetics of gibbsitic bauxite under atmospheric pressure in NaOH solution [J]. Journal of Central South University, 2017, 24(3): 489-495. DOI: 10.1007/s11771-017-3451-7.
Foundation item: Projects(51104041, 51174054, 51374065) supported by the National Natural Science Foundation of China; Project(N130402010) supported by the Fundamental Research Funds for the Central Universities of China
Received date: 2015-12-14; Accepted date: 2016-05-11
Corresponding author: PAN Xiao-lin, PhD; Tel: +86-24-83686460; E-mail: panxl@smm.neu.edu.cn