
Synthesis of Mg-Cu-Gd amorphous alloy by mechanical alloying and its thermal stability
XU Chun-xia(许春霞), PAN Fu-sheng(潘复生) , WANG Jing-feng(王敬丰),
TANG Ai-tao(汤爱涛), HU Yao-bo(胡耀波)
Chongqing Engineering Research Center for Magnesium Alloys, Chongqing University, Chongqing 400044, China
Received 28 July 2006; accepted 15 September 2006
Abstract: Mg65Cu25Gd10 amorphous alloy powders were synthesized by mechanical alloying (MA). The most suitable process is that the milling velocity is 600 r/min and the mass ratio of ball to powder is 20?1, so the shortest forming time of the amorphous is about 40 h. The thermal stability of the powders was analyzed by differential scanning calorimetry (DSC). The glass transition temperature, (Tg), onset temperature of crystallization(Tx), width of supercooled liquid region, were determined to be 444.8, 531.1 and 86.3 K, respectively. Contrasting them with the parameters of samples produced by traditional copper-mold casting, it is found that the thermal stability of the former is better than that of the latter. At the same time, the crystallization kinetics of those amorphous powders was researched by Kissinger equation and the apparent activation energy at Tg and Tx was calculated.
Key words: Mg65Cn25Gd10 alloy; magnesium amorphous alloys; mechanical alloying; thermal stability; apparent activation energy
1 Introduction
Although crystal magnesium alloy has high specific strength and good damping properties, its strength and corrosion-resisting properties are still low. At the same time, amorphous magnesium alloy has higher mechanical properties, its strength, ductibility and corrosion-resisting properties are better than those of crystal magnesium alloy[1]. Up to recent decade, a series of multicomponent metallic alloys such as Mg65Cu25Gd10[2], Mg65Cu15Y10-
Ag5Pd5[3], Mg65Cu20Ag5Gd10[4], Mg54Cu28Ag7Y11[5], Mg65Cu20Ni5Gd10[6] and so on[7-13] with superior glass-forming ability and high thermal stability against crystallization were discovered. Among these new bulk glasses forming metallic alloys, Mg54Cu26.5Ag8.5Gd11[5] is by far the best glass former, with size of 25 mm.
MgCuGd alloys with high glass-forming ability were fabricated by copper-mold casting generally. Whereas the critical cooling rate makes the size of the amorphous alloy and the industrial application limited. So, it is necessary for researchers to find a better way to synthesize bigger bulk metallic glass (BMG). They found subsequently that if amorphous powders prepared by ball milling can be got, bigger bulk amorphous alloy produced by compaction of the glassy powders can be acquired. At the same time, it is seldom reported that the amorphous magnesium alloys are fabricated by mechanical alloying. Hence the experiment’s purpose is to prepare Mg65Cu25Gd10 amorphous powders, explore the technics of ball milling and analyze the thermal stability. Finally, bigger bulk amorphous alloy and composites can be fabricated by compression forming in subsequent work and industrialization of amorphous magnesium alloy will be promoted.
2 Experimental
The mechanical alloying started from elemental Mg, Cu and Gd powders with nominal purities of 99% for Mg, 99.8% for Cu and 99.9% for Gd. All powders handing was done in a glove box under argon atmosphere. For the composite samples the elements were mixed to give a composition of Mg65Cu25Gd10. The powders were milled in a FRITSCH planetary ball mill at rotational velocity of 600 r/min for different time with mass ratio of ball to powder of 20?1. The microstructure was characterized by X-ray diffractometry(XRD) using Rigaku D/max-3A diffractometer with Cu Ka radiation. The amorphous nature of the powders was approved with the XRD
The differential scanning calorimetry(DSC) Measure-
ments were employed under purified argon atmosphere in the Perkin Elmer DSC 7 at the heating rate of 20, 30, 40 and 50 K/min, respectively. The calorimeter was calibrated for temperature with high purity indium and zinc with an accuracy of ±0.1 K. The values of the glass transition temperature (Tg), the crystallization temperatures (Tx) and the crystallization peak temperatures (Tp) were determined from the DSC traces with an accuracy of ±1 K. The apparent activation energy E for the glass transition and the crystallization transition can be evaluated by Kissinger equation:
= 
(1)
where T stands for Tg, Tx or Tp, F is the scanning rate in DSC, R is the gas constant. The apparent activation energy E can be deduced from the slope in curve of ln(Φ/T2) vs 1/T.
3 Results and discussion Fig.1 shows the XRD patterns for the specimens milled for different time. After milled for 33.5 h, partial crystallization of the alloy occurs progressively, starting at about 40 h with some crystalline peaks of remnant Gd2O3.
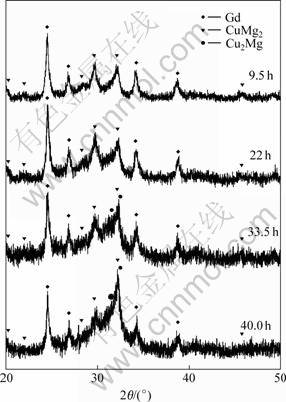
Fig.1 XRD patterns of Mg65Cu25Gd10 powders milled for different times
Fig.2 shows a comparison of DSC traces for the alloy at different heating rates. Two steps crystallizations occur on the curves and the second crystallization exothermic peak is stronger than the first one. When the heating rate is 20 K/min, the glass transition temperature Tg is 444.8 K, the onset temperature of the first crystallization event Tx1 is 531.1 K. ΔTx=Tx1-Tg, referring to as the supercooled liquid region, is 86.3 K. Contrast to the parameters (Tg=408 K, Tx1=478 K and ΔTx=70 K) of traditional copper-mold casting, the values of parameters of mechanical alloying were enhanced distinctly. The results indicate that the sample fabricated by mechanical alloying has better thermal stability and stronger glass-forming ability. The data in detail are listed in Table 1.
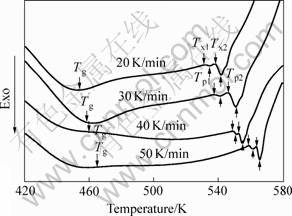
Fig.2 DSC curves of Mg65Cu25Gd10 under different heating rates
Table 1 Thermodynamics behavior of Mg65Cu25Gd10 amorphous powders
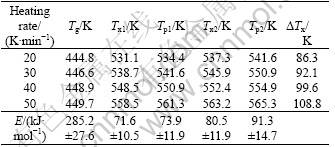
As shown in Fig.2, with the increase of heating rate F, Tg, Tx and Tp move to the area of high temperature and ΔTx becomes broader. The result indicates that the crystallization behavior and glass transition behavior correspond to the heating rate. Therefore it can be concluded that the glass transition and crystallization have remarkable dynamics effect.
Tx, Tg and ΔTx at a certain heating rate can be regarded as guide line to thermal stability of amorphous alloys commonly, however, the activation energy (E) for the glass transition reflects the energy barrier for glass transition.
Fig.3 shows Kissinger plots of Tg, Tx1, Tx2 and Tp1, Tp2 for Mg65Cu25Gd10 alloys, which indicates that there exists good linear relationship between ln(Φ/T2) and 1/T. The activation energy for the glass transition Eg=(285.2±27.6) kJ/mol can be calculated from the linear slope.
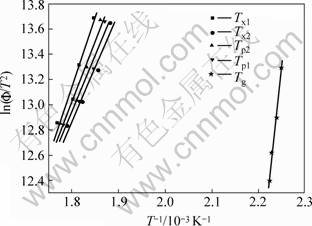
Fig.3 Kissinger plots of Tg, Tx1, Tx2, Tp1 and Tp2 for Mg65Cu25-Gd10 alloys
By Eqn.(1), the activation energy for Tx1, Tx2, Tp1 and Tp2 is shown in Fig.3 and listed in Table 1. The apparent activation energy (E) for the crystallization transition reflects the energy barrier for crystallization transition essentially.
In conclusion, the glass transition and the crystallization transition for bulk amorphous alloy have remarkable dynamic effect. With the increase of heating rate, the glass transition temperature (Tg) does not change observably, but the crystallization temperatures (Tx) increase obviously. The result is probably because the apparent activation energy (E) for the crystallization transition is far less than that for the glass transition.
4 Conclusions
1) Mg65Cu25Gd10 amorphous powders can be prepared by mechanical alloying, the most suitable process is suggested as follows: the rotational velocity is 600 r/min and mass ratio of ball to powder is 20?1.
2) The phenomenon that Mg65Cu25Gd10 amorphous powders produced by mechanical alloying have better thermal stability and broader extension of the supercooled liquid region (ΔTx) than those produced by Cu-mold casting deserves further research.
References
[1] PRYDS N H. Bulk amorphous Mg-based alloys[J]. Mater Sci Eng A, 2004, A375-377: 186-193.
[2] MEN H, KIM D H. Fabrication of ternary Mg-Cu-Gd bulk metallic glass with high glass-forming ability under air atmosphere[J]. Journal of Materials Research, 2003, 18(7): 1502-1504.
[3] XU Ying-kun, MA Han, XU Jian, MA En. Mg-based bulk metallic glass composites with plasticity and gigapascal strength[J]. Acta Mater, 2005, 53: 1857-1866.
[4] PARK E S, LEE J Y, KIM D H. Effect of Ag addition on the improvement of glass-forming ability and plasticity of Mg-Cu-Gd bulk metallic glass[J]. Journal of Materials Research, 2005, 20(9): 2379-2385.
[5] MA H, ShI L L, XU J, LI Y, MA E. Discovering inch-diameter metallic glasses in three-dimensional composition space[J]. Appl Phys Lett, 2005, 87(18): 181915.
[6] YUAN Guang-yin, INOUE A. The effect of Ni substitution on the glass-forming ability and mechanical properties of Mg-Cu-Gd metallic glass alloys[J]. J Alloys Compd, 2005, 387: 134-138.
[7] AMIYA K, INOUE A. Preparation of bulk glassy Mg65Y10- Cu15Ag5Pd5 alloy of 12 mm in diameter by water quenching[J]. Mater Trans, JIM, 2001, 42(3): 543-545.
[8] XI X K, ZHAO D Q, PAN M X, WANG W H. On the criteria of bulk metallic glass forming in MgCu-based alloys[J]. Intermetallics, 2005, 13: 638-641.
[9] PARK E S, KIM D H. Formation of Mg-Cu-Ni-Ag-Zn-Y-Gd bulk glassy alloy by casting into cone-shaped copper mold in air atmosphere[J]. Journal of Materials Research, 2005, 20(6): 1465-1469.
[10] LIU W Y, ZHANG H F, HU Z Q, WANG H. Formation and mechanical properties of Mg65Cu25Er10 and Mg65Cu15Ag10Er10 bulk amorphous alloy[J]. J Alloys Compd, 2005, 397: 202-206.
[11] MA H, ZHENG Q, XU J, LI Y, MA E. Doubling the critical size for bulk metallic glass formation in the Mg-Cu-Y ternary system[J]. Journal of Materials Research, 2005, 20(9): 2252-2255.
[12] BARTUSCH B, SCHURACK F, ECKERT J. High strength magnesium-based glass matrix composites[J]. Mater Trans, 2002, 43(8): 1979-1984.
[13] MA H, XU J, MA E. Mg-based metallic glass composites with plasticity and high strength[J]. Appl Phys Lett, 2003, 83(14): 2793-2795.
(Edited by CHEN Wei-ping)
Foundation item: Project (2006BB4199) supported by the Natural Science Foundation of Chongqing
Corresponding author: WANG Jing-feng; Tel: +86-23-65102821; E-mail: jfengwang@163.com