
Relationship between solid/liquid interface and crystal orientation for pure magnesium solidified in fashion of cellular crystal
CHANG Guo-wei(常国威), CHEN Shu-ying(陈淑英), ZHOU Cong(周 聪),
YUE Xu-dong(岳旭东), QI Yi-hui(齐义辉)
School of Materials and Chemical Engineering, Liaoning University of Technology, Jinzhou 121001, China
Received 23 September 2008; accepted 25 March 2009
Abstract: The relationship between the solid/liquid interface and the crystal orientation for pure magnesium, which grows in fashion of cellular crystal in unidirectional solidification, was investigated. The results show that the energy of the solid/liquid interface is the lowest during cellular crystal growth of pure magnesium; and the solid/liquid interface is covered by the basal face {0001} and by the crystal face made up of three atoms located at the orientation {0001}<0100> and two atoms located at the inner of magnesium crystal cell. The strongest bond is formed in the direction of 61.9? deviating from the growth direction, and the second strong bond is formed in the directions of 8.5? and 47.7?, respectively, deviating from the growth direction. The angle between the basal face {0001} and the growth direction is 61.9?. The theoretical analysis results are basically consistent with the experimental results from SUSUMU et al.
Key words: magnesium; solidification process; crystal growth; crystal orientation
1 Introduction
Pure magnesium is of the close-packed hexagonal structure, and its plastic deformation at room- temperature depends on the slipping of the basal face
and the twin of the pyramidal face
[1-2]. Two methods are generally applied to improving the plastic deformation ability of magnesium at room temperature. One is to decrease the axial ratio (c/a) of magnesium crystal through adding alloying agents, such as Li, In and Ag[3]; the other is grain refinement to accommodate the slipping of the basal face and the twin of the pyramidal face[4]. The brittle-to-ductile transition temperature (BDTT) can be reduced to room-temperature when the grain size of pure magnesium is 8 μm[5]. The superplasticity is obtained when grain size is 1 μm at room temperature[6]. As the non-crystal magnesium is not easily obtained[7-11], the new processes are expected.
Because of the particularity of crystal structure of magnesium and magnesium alloys, the intragranular plastic deformation at room temperature takes on the directivity. In order to display sufficiently the effect of basal face slipping on macroscopical plastic deformation at room temperature, the basal face of every grain should keep a suitable angle with the applied stress direction. It is very important to control grain orientation. For equiaxed crystal, it is impossible that the basal face of every grain is all parallel to each other. However, for columnar crystals obtained through unidirectional solidification, it is possible that the basal face of every grain is parallel to each other. So far, how to control definite relationship between the growth direction of columnar crystals and the grain orientation is less reported.
In this work, the growth processes of cellular crystal in the process of unidirectional solidification of pure magnesium were researched, and the relationship between growth direction of cellular crystal and crystal orientation was also investigated.
2 Relationship between growth direction and crystal orientation
Cellular or dendritic crystal can be obtained through unidirectional solidification. KURZ and FISAER[12] pointed out that the growth direction of cellular crystal depends only on heat-flow direction and has no relation to crystal orientation in the solidification. The dendritic growth is controlled by both heat current direction and crystal orientation. Main arbors of dendrite are preferential growth directions of crystal, which are close to heat current direction. The relationship between growth direction of dendrite and crystal orientation is quite clear. However, for cellular crystal, the result that crystal growth has no relation to crystal structure is not proper. The experimental results show that the growth direction of cellular crystal is parallel to and reverse to the heat-flow direction. However, it cannot be proven that there are no relations between growth direction and crystal orientation in the process of the cellular crystal growth. It can be affirmed that there exist corresponding relationships among the growth direction of cellular crystal, the solid-liquid interface energy, the crystal orientation and the crystal face in the solidification.
HARTMAN[13] pointed out that the crystal grew along the direction forming the strongest bond and was enclosed by low energy interface. According to the viewpoint, for cellular crystal growth, the positions forming the strongest bond in solid phase are firstly taken up when the atoms transfer from the liquid phase to the solid phase, and the solid/liquid interface must be the crystal face with the lowest energy. This request can be satisfied for certain crystals. For example, for simple cubic crystal, the bond of the orientation <001> is the strongest, and the energy of the crystal face which is normal to the orientation <001> is the lowest. It is very difficult that those two conditions are synchronously satisfied when the crystal structure is comparatively particular. For example, for the close-packed hexagonal structure, the strongest bond is the orientation
and the energy of prism face normal to the orientation
is the largest. Under this condition, it is a conflict for cellular crystal to grow along the strongest bond and to make the energy of the solid/liquid interface the lowest, so it is not likely to grow in either of two fashions. It is possible that two fashions are harmonized, i.e., the growth direction of crystal is not along the direction forming the strongest bond, and the energy of the solid/liquid interface is also not the lowest.
3 Structure of solid/liquid interface for magnesium growing in cellular crystal
3.1 Separation angle between typical crystal face and basal face for magnesium crystal
The crystal structure of magnesium is shown in Fig.1 from Ref.[1]. The calculation results of the separation angles between the typical crystal face and the basal face {0001}, and the atomic density of the crystal face are shown in Table 1. It can be seen from Table 1 that the crystal face {0001} is a closest-packed face, and the crystal face {DEFG} is a secondary closest-packed face. Because the difference of the atomic densities between them is very small, the two crystal faces are considered the closest-packed faces at the same time, and the energies of them are the lowest. In contrast, other crystal faces are not closest-packed faces.
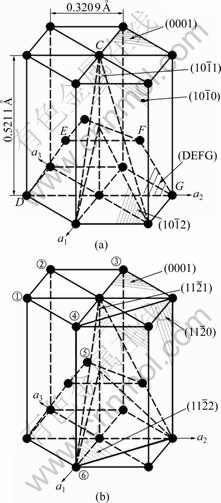
Fig.1 Unit cell of magnesium (①-⑥ represent atom position numbers): (a) Unit cell; (b) Orientation relations of various crystal faces
Table 1 Calculation results of relative parameters for magnesium crystal
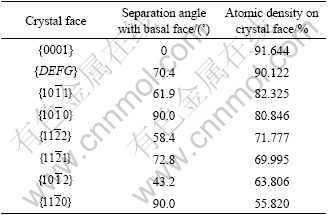
3.2 Valence electron structure of magnesium crystal
According to the calculation method of the valence electron structure (VES) of alloy proposed in Ref.[14], the VES of magnesium crystal cell is calculated on the basis of the structure and lattice constants of magnesium crystal shown in Fig.1, and the calculation results of the VES are shown in Table 2. In Table 2, σMg is the hybridization state of Mg atom;
and
represent the numbers of covalent electron and lattice electron in the valence electron layer of Mg atom on the third hybridization state, respectively;
is the single bond radius of Mg atom when it is in the third hybridization state; Iα is the equivalent bond number of α bond; Dna is the experimental length of α bond;
is the theoretical length of α bond;
is the bond length difference between experimental bond length and the theoretical one; na is the number of covalent electron pairs on the α bond;
is the lattice electron number of unit volume;
is the covalent electron number of unit volume; b is a factor in the bond length difference of empirical electron theory of solids and molecules, which is 0.071 0 nm here;
is the bond name of A bond formed by atoms ① and ② in Fig.1;
,
and
represent the bond names formed by atoms ② and ⑤, ④ and ⑤, ④ and ⑥, ① and ③, respectively. The space distributions of bonds A and B are shown in Fig.2.
Table 2 Valence electron structure of magnesium unit cell
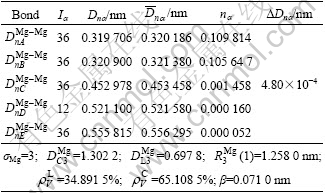
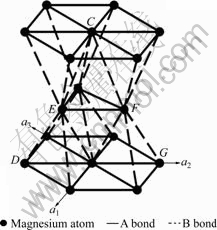
Fig.2 Space distributions of bonds A and B in magnesium crystal cell
In the view of Ref.[14], the larger the nα is, the stronger the bond strength is. From Table 2, it can be seen that the nα value of bond A is the largest, which indicates that bond A is the strongest bond, and bond B is the second strong bond. It can also be found that the strengths of bonds A and B are nearly identical; however, the nα values of bonds C, D and E are quite smaller than those of bonds A and B. The bond strengths of bonds C, D and E are very small, so it is easy to grow along the direction of bond A, and it is also possible to grow along the direction of bond B during the growth process of crystal. The final growth direction depends upon the energy of the solid/liquid interface.
3.3 Crystal face on solid/liquid interface of magnesium solidified in cellular crystal
According to Hartman theory, by combining Fig.1, Fig.2 and Table 2, and considering the synthetical factors the bonding direction and the energy of the solid/liquid interface, the relationship between crystal orientation on the solid/liquid interface and the bonding direction, of which liquid atoms are changed into solid state, is shown in Fig.3.
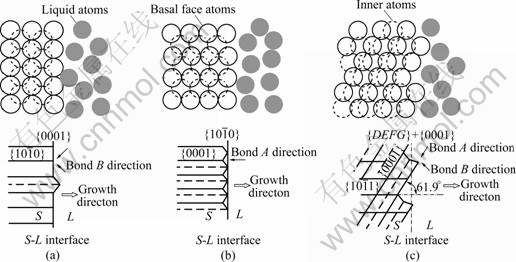
Fig.3 Sketch map of solid/liquid interface structure and crystal orientation: (a) S-L interface covered by {0001}; (b) S-L interface covered by
(c) S-L interface covered by both {0001} and {DEFG}
Fig.3(a) shows that the solid/liquid interface is covered by the basal face {0001} of magnesium, where bond B is formed in the direction of 19.6? deviating the growth direction, and bond A depending on bond B is formed in the direction normal to the crystal growth direction. The crystal face {DEFG} is formed as a result of the atom stacking on the crystal face {0001}. The solid/liquid interface is together covered by the crystal faces {0001} and {DEFG}. Under this condition, the energy of the solid/liquid interface is the lowest, in despite of the fact that the second strong bond B can be formed; however, the strongest bond A cannot be directly formed. So, this mode cannot exist in the process of solidification. The strongest bond A can be easily formed according to the atom occupying mode from Fig.3(b), and the low-energy crystal face {DEFG} can also appear on the solid/liquid interface in the solidification. The simultaneous appearance for the crystal faces
and {DEFG} will result in the fact that the solid/liquid interface is covered by the prism face and its energy is the largest. So, it is not easy to exist for this kind of mode. Fig.3(c) is the middle state between Fig.3(a) and Fig.3(b). The crystal face
is parallel to the crystal growth direction, and the solid/liquid interface is covered by the basal faces {0001} and crystal face {DEFG}. From atoms scale, the solid/liquid interface is in rugosity and it is easy to receive the liquid atoms. Bond A will be formed in the direction of 61.9? deviating from the growth direction, and bond B will be formed in the directions of 8.5? and 47.7?, respectively, deviating from the growth. Because the energy of crystal face {DEFG} is also the lowest, the energy of the solid/liquid interface is the lowest and the separation angle between the basal face {0001} and the growth direction is 61.9?. By analyzing, we can draw conclusion that the solid/liquid interface cannot be covered by other crystal faces, which is not in agreement with Hartman theory. It can be deduced that Fig.3(c) is the easiest way to exist when magnesium grows in fashion of cellular crystal.
The grain arrangement is fixed in the solidification structure after the solidification, namely, the separation angle between the basal face and the growth direction is 61.9? when magnesium grows in fashion of cellular crystal.
The slab of pure magnesium was prepared through Ohno continuous casting method by SUSUMU et al[15], and the separation angle between crystal face {0001} and crystal growth direction was measured through X-ray diffraction analysis; and the results are shown in Fig.4. The crystal face {0001} is parallel to the width direction of the slab, and the angle with the crystal growth direction is 65?. As mentioned above, the grain arrangement is fixed in the solidification structure after the solidification, so the grain arrangement in the solidification structure represents indirectly the crystal face arrangement on the solid/liquid interface in the solidification. Hence, the experimental results of SUSUMU et al can be used to verify the results of the theoretical analysis above. By comparison, the theoretical analysis, i.e., the segregation angle between the basal face and the crystal growth direction is 61.9? when magnesium grows in fashion of cellular crystal, is consistent with the experimental result of SUSUMU et al. The difference of 3? exists between the experimental result and the theoretical one, which is thought to be the result of the existence of the sub-boundary in pure magnesium structure.
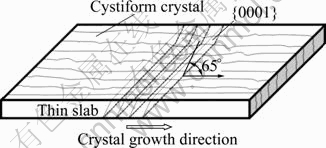
Fig.4 Sketch map of relation between {0001} and growth direction of cellular crystal for pure magnesium slab
4 Conclusions
1) The solid/liquid interface is covered by the basal face {0001} and the crystal face {DEFG}, which is made up of three atoms located at the orientation {0001}<0100> and two atoms located at the inner of crystal cell, so the energy of the solid/liquid is the lowest. Bond A is formed in the direction of 61.9? deviating the growth direction; bond B is formed in the direction of 8.5? and 47.7? deviating the growth direction; and the separation angle between the basal face {0001} and the growth direction is 61.9?.
2) Theoretical analysis is basically consistent with the experimental result of SUSUMU et al.
Acknowledgements
The authors are grateful to Prof. LIU Wei-dong in School of Materials and Chemical Engineering, Liaoning University of Technology, China, for extending the calculation results of the valence electron structure for magnesium unit cell.
References
[1] ASM International. Magnesium and magnesium alloy [M]. OH: Metal Park, 1999: 1-18.
[2] POLMEAR I J. Magnesium alloy and applications [J]. Mater Sci and Tech, 1994, 10(1): 1-16.
[3] KU K. Magnesium alloys and their applications [M]. Oberursel: DGM Information Sgesellschaft, 1992: 19-23.
[4] KUROTA K, MABUCHI M, HIGASHI K. Review processing and mechanical properties of fine-grained magnesium alloys [J]. J Mater Sci, 1999, 34(10): 2255-2259.
[5] EMLEY E F. Principles of magnesium technology [M]. Oxford: Pergamon, 1966: 122-125.
[6] DAS S K, CHANG C F. Magnesium alloys and their applications [M]. Oberursel: DGM Information Sgesellschaft, 1992: 56-60.
[7] WANG Bin, YANG Yuan-sheng, ZHOU Ji-xue, TONG Wen-hui. Microstructure refinement ofAZ91D alloy solidified with pulsed magnetic field [J]. Transactions of Nonferrous Metals Society of China, 2008, 18(3): 536-540.
[8] MIZUTANI Y, TAMURA T, MIWA K. Microstructural refinement process of pure magnesium by electromagnetic vibrations [J]. Mater Sci Eng A, 2005, 413/414A: 205-207.
[9] FENG Can-feng, ZHANG Xing-guo, JI Shou-hua, JIN Jun-ze, CHANG Yu-bao. Microstructure and corrosion property of AZ61 magnesium alloy by electromagnetic stirring [J]. Transactions of Nonferrous Metals Society of China, 2005, 15(3): 536-541.
[10] ESKIN G I, ESKIN D G. Some control mechanisms of spatial solidification in light alloys [J]. Z Metallk, 2004, 95(8): 682-686.
[11] ZHANG Hai-bo, ZHAI Qi-jie, QI Fei-ping, GONG Yong-yong. Effect of side transmission of power ultrasonic on structure of AZ81 magnesium alloy [J]. Transactions of Nonferrous Metals Society of China, 2004, 14(2): 302-305.
[12] KURZ W, FISHER D J. Fundamentals of solidification [M]. Aedermannsdorf: Trans Tech Publications, 1989: 27-31.
[13] HARTMAN P. Crystal growth [M]. Amsterdam: North-Holland Publishing Company, 1973: 35-52.
[14] LIU Zhi-lin, LI Zhi-lin, LIU Wei-long. Interface electron structure and interface property [M]. Beijing: Science Press, 2002: 38-156. (in Chinese)
[15] SUSUMU O, HIROKI K, SATOSHI H, SEI M. Crystal orientation and yield strength of pure magnesium continuously cast with a heated mold [J]. Japan Inst Metals, 1995, 59(6): 607-611. (in Japanese)
Foundation item: Project(2007T078) supported by the Outstanding Innovation Team in Colleges and Universities of Education Department of Liaoning Province, China
Corresponding author: CHANG Guo-wei; Tel: +86-416-4198809; E-mail: g.w.chang@hotmail.com
DOI: 10.1016/S1003-6326(09)60136-9
(Edited by YANG Hua)