
Effect of surface modification of metal hydride electrode on performance of MH/Ni batteries
YANG Kai(杨 凯), WU Feng(吴 锋), CHEN Shi(陈 实), ZHANG Cun-zhong(张存中)
School of Chemical Engineering and Environmental Science, Beijing Institute of Technology, Beijing 100081, China;
National Development Center of Hi-Tech Green Materials, Beijing 100081, China
Received 2 November 2005; accepted 6 March 2006
Abstract: A novel method was applied to the surface modification of the metal hydride(MH) electrode of MH/Ni batteries. Both sides of the electrode were plated with a thin silver film about 0.1μm thick using vacuum evaporation plating technology, and the effect of the electrode on the performance of MH/Ni batteries was examined. It is found that the surface modification can enhance the electrode conductivity and decrease the battery ohimic resistance. After surface modification, the discharge capacity at 5C (7.5A) is increased by 212 mA?h and the discharge voltage is increased by 0.11 V, the resistance of the batteries is also decreased by 32%. The batteries with modified electrode exhibit satisfactory durability. The remaining capacity of the modified batteries is 89% of the initial capacity even after 500 cycles. The inner pressure of the batteries during overcharging is lowered and the charging efficiency of the batteries is improved.
Key words: surface modification; vacuum evaporation plating technology; electrode; MH/Ni batteries
1 Introduction
The MH/Ni batteries have been commercialized and used widely in portable electric applications and electric vehicles because of their advantages such as cleanness, high energy density and no pollution[1,2]. As the active material of the negative electrode, the hydrogen storage alloys have also attracted much attention. So far, many multi-component, mischmetal-based hydrogen storage alloys have been developed[3,4]. In order to improve the performance of hydrogen storage alloys, several kinds of surface modifications have been employed. The micro- encapsulation of the alloys powder with an electroless plating of copper or nickel has been confirmed to be effective in improving the electrochemical characteristics of the negative electrode and decreasing the inner pressure of the MH/Ni batteries[5-7]. The surface treatment with alkaline and acid leads to the appearance of a Ni-rich surface, which is effective in enhancing the activation performance of alloys[8-11]. The modification by plating the MH electrode with platinum group metals, Co and Ni has been proved to be effective in improving the high-rate dischargeability as well as accelerating the activation process[12-14].
In this work, a novel method is employed to modify the surface of the MH electrode. The MH electrode is plated with a thin silver film using vacuum evaporation plating technology. The electrochemical properties such as the charge-discharge characteristics, the cycle life and the inner pressure of the batteries are investigated. In addition, the surface structure of the electrode is analyzed by X-ray photoelectron spectroscopy(XPS) and scanning electron microscope(SEM). The crystal structure of the alloy is identified by X-ray diffraction (XRD) and the particle size distribution of the alloy powders is also examined.
2 Experimental
The Ingots of MlNi3.6Co0.7Mn0.3Al0.4 (Ml=La-rich mischmetal, La 55.4%; Ce 26.2%; Pr 5.4%; Nd 12.6%; other rare earths 0.4%, in mass fraction), were mechanically crushed into powders with particle size 30-70 μm. The MH electrode was constructed by packing the paste of alloy powder, nickel powder and 3%(mass fraction) polytetrafluoroethylene(PTFE) as a binder into a foamed nickel substrate and then cold-compressed under a pressure of 20 MPa to 0.3 mm thickness. Both sides of the electrode were subsequently plated with a thin silver film of about 0.1 μm thickness in vacuum using vacuum evaporation plating technology.
The surface structure of the electrode was determined by X-ray photoelectron spectroscopy(XPS) equipped with an KRATOS XSAM800 spectrometer with Al Kα radiation. The surface morphology of the electrode was examined by scanning electron microscopy (SEM, JSM-35C). The crystal structure of the electrode was identified by X-ray diffraction(XRD, Rigaku Dmax-2500) with Cu Kα radiation.
The AA-size MH/Ni batteries were assembled using the surface modified MH electrode as the negative electrode. The electrolyte was 6 mol/L KOH+1 mol/L LiOH solution. The nominal capacity of the batteries was 1 500 mA?h. The sealed MH/Ni batteries were firstly activated by charge-discharge at a rate of 0.2C(300 mA) for 5 cycles. Then the discharge capacity of the MH/Ni batteries was determined at a rate of 0.2C. The performance of the batteries at high-rate discharging was evaluated from the discharge capacity and the plateau voltage during discharge at 1C, 3C, and 5C rate, respectively. In the cycle life test, the batteries were charged at 1C by controlling to cut-off at ?V=-10 mV, discharged at 1C to 1.0 V after a rest of 10 min. All the tests above were carried out on an Arbin instrument. The ohmic resistance of the batteries was tested by HIOKI 3551 Batteries Hitester. After the cycle life test, the particle size distribution of the alloy powder was examined by a HORIBA LA-300 laser scattering particle size distribution analyzer.
The inner pressure of the batteries during charging and discharging was measured by a battery inner pressure test system at 20-25℃ as reported previously [15]. The batteries were placed into a chamber and the gas generated during charging was led out to a pressure sensor through a hole on the top of batteries.
3 Results and discussion
3.1 Surface and crystal structure analysis of electrode
The SEM photographs of the electrode surface are shown in Fig.1. It is shown that the surface of the unmodified electrode is relatively smooth, and there exist some cleavage and small particles of 200 nm diameter on the surface. As for the modified electrode, the surface appears coarse and the cleavage which appears on the unmodified electrode is covered.
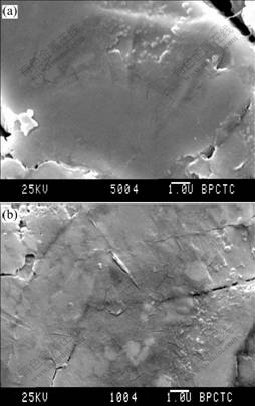
Fig.1 SEM photographs of metal-hydride electrode: (a) Surface modified electrode; (b) Surface unmodified electrode
The XPS analysis of the surfaces of the unmodified and modified electrodes was performed (Fig.2). Com- pared with the unmodified electrode, it is observed on the modified electrode that Ag exists in the metallic state, and is signed by Ag 3d5/2 and Ag 3d3/2 peaks with binding energy 368.1 eV and 374.0 eV, respectively. From the results of XPS analysis, the electrode can be surely plated with silver using the method described in this paper.
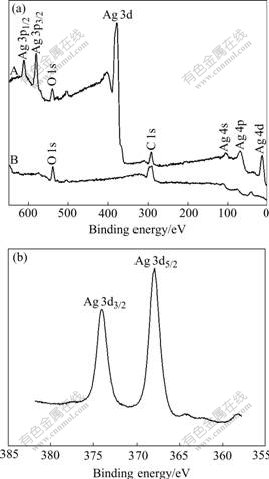
Fig.2 XPS spectra of electrode surface: (A) Surface modified electrode; (B) Surface unmodified electrode
The XRD patterns of the modified and unmodified electrodes are shown in Fig.3. The same structure can be observed in the two cases except that the peaks belonging to the Ag can be found in the pattern of the modified electrode. The result indicates that the surface modification method used in this work has no influence on the crystal structure of the alloy.
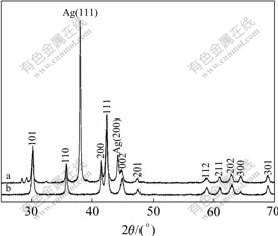
Fig.3 XRD patterns of metal-hydride electrode: (a) Surface modified electrode; (b) Surface unmodified electrode
3.2 Charge-discharge characteristics of MH/Ni batteries
Fig.4 shows the typical discharge curves at different rates for the MH/Ni batteries with modified and unmodified electrodes, respectively. The discharge capacity of the batteries with unmodified electrode is 1 414 mA?h at 1C, 1 148 mA?h at 3C and 1 069 mA?h at 5C. The plateau voltage during discharge is 1.20 V at 1C, 1.11 V at 3C and 0.92 V at 5C, respectively. For the batteries with modified electrode the capacity is 1 450 mA?h at 1C, 1 228 mA?h at 3C and 1 281 mA?h at 5C, and the plateau voltage is 1.22 V at 1C, 1.15 V at 3C and 1.03 V at 5C, respectively. It is obvious that the discharge capacity and the plateau voltage of the batteries with modified electrode are much higher than those of the unmodified one. Moreover, with the increasing discharge current, the differences in the discharge capacity and the plateau voltage between the two batteries become obviously large. Clearly, the electrode modification improves the high-rate dischargeability remarkably. The ohmic resistances of the two batteries are measured to be 25 mΩ and 17 mΩ for the unmodified and modified electrodes, respectively. Obviously, the modification of negative electrode can effectively decrease the inner resistance of the batteries. This result can be ascribed to the role of the silver film plated on the electrode. The presence of silver enhances the effectiveness of the current collection processes, and further improves the charge transfer process on the interface between the electrode and electrolyte interface.
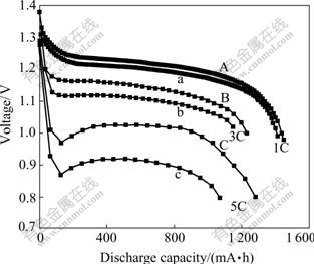
Fig.4 Discharge curves of AA-size batteries at 1C(1.5 A), 3C (4.5 A) and 5C(7.5 A): (A), (B), (C) With modified electrode; (a), (b), (c) With unmodified electrode
3.3 Charge-discharge cycle test
The discharge capacity of the MH/Ni batteries as a function of cycle number is shown in Fig.5. The initial discharge capacity of both batteries is all about 1 520 mA?h. The discharge capacity of both batteries decays progressively after 100 cycles. This can be ascribed to the continuous oxidation and disintegration of the alloy during cycling. Conspicuously, the capacity loss of the batteries with unmodified electrode is larger than that of the batteries with the modified electrode. After 500 cycles, the batteries with modified and unmodified electrode have the capacities of 1 352 mA?h and 1 235 mA?h, respectively. The discharge performance of the batteries is significantly stabilized after electrode modification and exhibits satisfactory durability.
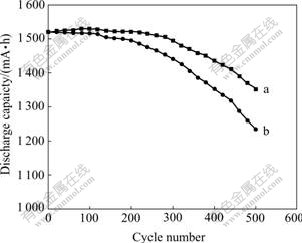
Fig.5 Cycle durability for AA-size batteries: (a) With modified electrode; (b) With unmodified electrode
The surface XPS analysis of the modified electrode after 500 cycles was performed and the atomic relative ratio of Ag, O, Ni on the modified electrode by XPS analysis is shown in Table 1.
Table 1 Surface components analyzed by XPS for modified electrode
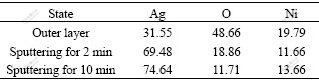
Though a high level of oxygen is observed on the outer layer, which can be attributed to the rare earth oxides that form readily on the surfaces of intermetallic hydrides, as reported by SCHLAPBACH, it drops greatly after sputtering for 2 min. This suggests that the silver film on the electrode restrains the oxidation of the alloy. Since the overcharge of the batteries accelerates its capacity decay, the oxidation should be caused mainly by the oxygen evolved on the nickel cathode during the charging process and then transferred to the anode across the thin separator. The longer cycle life of the batteries with the modified electrode can be partly ascribed to the role of silver film as an oxygen barrier, which protects the alloy surface from the oxidation.
Fig.6 displays the granularity distribution curves of the alloy powders which are stripped out from the MH electrode after 500 cycles using ultrasonic vibrations. The granularity distribution curve of the alloy powders from the unmodified electrode shifts to lower granularity region compared with the alloy powders from the modified electrode. The mean particle size of the modified and unmodified electrode are 17.28 μm and 5.65 μm, respectively. Obviously, the disintegration of the alloy is reduced due to the silver film plated on the electrode.
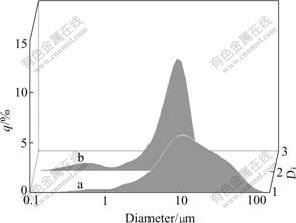
Fig.6 Particle size distribution curves of alloy powders of MH electrode: (a) Surface modified electrode; (b) Surface unmodified electrode
Combining the results of cycle life, XPS analysis and particle distribution, it is clearly proved that the silver plated on the electrode acts as a protective layer, which effectively restrains the alloy from oxidation and pulverization, which are known as the main causes that lead to the degradation of the batteries. Therefore, the decay of the capacity is decreased and the cycle life is prolonged.
3.4 Inner pressure of batteries
Fig.7 shows the inner pressure change of AA-size MH/Ni batteries when charging at 0.5C rate.
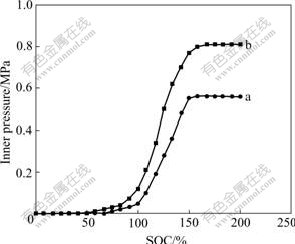
Fig.7 Inner pressure of MH/Ni batteries at 0.5C charge: (a) With modified electrode; (b) With unmodified electrode
It can be seen that the inner pressure of the batteries remains unchanged before being charged up to 50% of nominal capacity. After being charged up to 60%, the inner pressure begins to increase, but the rate of the increase is low. After being charged up to 100%, the inner pressure increases sharply. When charged up to about 150%, the inner pressure reaches equilibrium and up to 200% of nominal capacity, the inner pressure remains constant. It indicates that, in this period, the rate of gas evolution equals the rate of gas consumption. It can be seen that the batteries with the modified electrode displays the slower increase of inner pressure, which reaches equilibrium at 0.55 MPa. For the batteries with unmodified electrode, the inner pressure at equilibrium is 0.81 MPa, which is higher than that of the batteries with modified electrode.
The inner pressure change at 1C rate is illustrated in Fig.8.
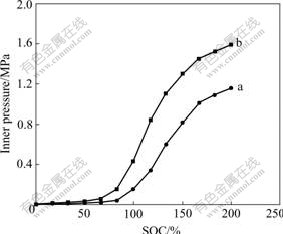
Fig.8 Inner pressures of MH/Ni batteries at 1C charge: (a) With modified electrode; (b) With unmodified electrode
The inner pressure of the unmodified batteries increases rapidly when approaching 80% of nominal capacity and reaches 1.59 MPa when charged up to 200% of nominal capacity. For the modified batteries, the inner pressure increases sharply when charged up to 100% and reaches 1.16 MPa at 200% of the nominal capacity.
The above results indicate that the inner pressure of the batteries decreases remarkably after surface modification. The decrease is due to the silver film on the electrode which has a role in preventing the release of hydrogen gas from the alloy surface, suppressing the recombination of hydrogen atoms.
4 Conclusions
The MH electrode of the MH/Ni batteries can be plated with a thin silver film by a surface modification method using vacuum evaporation plating technology. The crystal bulk structure of the alloy after modification remains unchanged. The silver film plated on the electrode can play following three important roles: 1) The microcurrent collector for facilitating the charge transfer on the electrode. The high-rate dischargeability of the batteries after the surface modification is improved, resulting from the enhancement of the electrode conductivity and the decrease of the batteries ohimic resistance. The plateau voltage increases by 0.11 V at 5C discharge rate. 2) The silver plated on the electrode surface also acts as a protective layer, which restrains the alloy from oxidation and pulverization. Therefore, the cycle life of the batteries is prolonged. 3) The inner pressure of the batteries can be decreased and the charging efficiency is improved
Acknowledgements
The authors are grateful for the assistance from Prof. HONG Cun-mao of Beijing University.
References
[1] ZHONG S, HOWES A, WANG G X, BRADHURST D H, WANG C, DOU S X, LIU H K. A new process for fabrication of metal-hydride electrodes for nickel-metal hydride batteries [J]. J Alloys Comp, 2002, 330/332: 760-765.
[2] ZHANG J X, YU J X, CHA C S, YANG H X. The effects of pulse charging on inner pressure and cycling characteristics of sealed Ni/MH batteries [J]. J Power Sources, 2004, 136: 180-185.
[3] DAN L, ALEXANDRU R B, ALEXANDRU S B, EMIL I, IOAN M, ANDREAS Z, LOUIS S. Cobalt-free over-stoichiometric Laves phase alloys for Ni-MH batteries [J]. J Alloys Comp, 2003, 350: 319-323.
[4] CHEN W X, XU Z D, TU J P. Electrochemical investigations of activation and degradation of hydrogen storage alloy electrodes in sealed Ni/MH battery [J]. International Journal of Hydrogen Energy, 2002, 27: 439-444.
[5] FENG F, NORTHWOOD D O. Improved performance of a metal hydride electrode for nickel/metal hydride batteries through copper-coating [J]. Surface and Coatings Technology, 2003, 167: 263-268.
[6] FENG F, NORTHWOOD D O. Effect of surface modification on the performance of negative electrodes in Ni/MH batteries [J]. International Journal of Hydrogen Energy, 2004, 29: 955-960.
[7] SAKAI T, YUASA A, ISHIKAWA H, MIYAMURA H, KURIYAMA N. Nickel-metal hydride battery using microencapsulated alloys [J]. J Less-Common Met, 1991, 172-174: 1194-1204.
[8] LIN H C, LIN K M, CHOU H T, YEH M T. Effects of annealing and NaOH pretreatment on an LmNi3.65Al0.34Mn0.27Co0.74 hydrogen storage alloy [J]. J Alloys Comp, 2003, 358: 281-287.
[9] YANAGIMOTO K, MAJIMA K, SUNADA S, SAWADA T. Effects of surface modification on surface structure and electrochemical properties of Mm(Ni, Co, Mn, Al)5.0 alloy powder [J]. J Alloys Comp, 2004, 377: 174-178.
[10] YUKA O, TOSHIRO K, HARU H U. Pulverization behavior of LaNi5 with alkaline pretreatment [J]. J Alloys Comp, 2002, 330/332: 632-635.
[11] UCHIDA H, YAMASHITA K, GOTO M. Comparison of pretreatments by alkaline (Li, Na, K) hydroxides with NH3-H2O in the work function and hydriding kinetics of LaNi2.5Co2.5 [J]. J Alloys Comp, 2002, 330/332: 622-626.
[12] MATSUOKA M, KOHNO T, IWAKURA C. Electrochemical properties of hydrogen storage alloys modified with foreign metals [J]. Electrochim Acta, 1993, 38: 787-791.
[13] WILLEY D B, HARRIS I R, PRATT A S. The improvement of the hydrogenation properties of nickel-metal hydride battery alloy by surface modification with platinum group metals (PGMs) [J]. J Alloys Comp, 1999, 293/295: 613-620.
[14] YANG K, WU F, CHEN S, ZHANG C Z. Influence of the surface modification of the MH electrode on the performance of the MH/Ni batteries [J]. Trans Nonferrous Met Soc China, 2005, 15(1): 183-188.
[15] HONG C M, XU J X, YANG J W. Inner pressure and activation of sealed Ni-MH battery [J]. Metallic Functional Materials, 1999, 6: 83-86.
Foundation item: Project(2002CB211800) supported by the National Basic Research Program of China; Project(05120404) supported by the Fundamental Research of Beijing Institute of Technology
Corresponding author: YANG Kai; Tel: +86-10-68912528; Fax: +86-10-68451429; E-mail: ykbit@bit.edu.cn
(Edited by YUAN Sai-qian)