
Mechanical properties and structure of zirconia-mullite ceramics prepared by in-situ controlled crystallization of Si-Al-Zr-O amorphous bulk
LIANG Shu-quan(梁叔全), ZHONG Jie(钟 杰), TAN Xiao-ping(谭小平), TANG Yan(唐 艳)
School of Materials Science and Engineering, Central South University, Changsha 410083, China
Received 10 November 2007; accepted 23 April 2008
Abstract: Zirconia-mullite nano-composite ceramics were fabricated by in-situ controlled crystallization of Si-Al-Zr-O amorphous bulk, which were first treated at 900-1 000 ℃ for nucleation, then treated at higher temperature for crystallization to obtain ultra-fine zirconia-mullite composite ceramics. The effects of treating temperature and ZrO2 addition on mechanical properties and microstructure were analyzed. A unique structure in which there are a lot of near equiaxed t-ZrO2 grains and fine yield-cracks has been developed in the samples with 15% zirconia addition treated at 1 150 ℃. This specific microstructure is much more effective in toughening ceramics matrix and results in the best mechanical properties. The flexural strength and fracture toughness are 520 MPa and 5.13 MPa·m1/2, respectively. Either higher zirconia addition or higher crystallization temperature will produce large size rod-like ZrO2 and mullite grains, which are of negative effect on mechanical properties of this new composite ceramics.
Key words: Si-Al-Zr-O amorphous bulk; crystallization; zirconia-mullite composite; structure
1 Introduction
Mullite has been widely investigated because of its outstanding importance as potential candidate of single-phase ceramics, composite ceramics for high temperature structured applications due to its favorable thermal and mechanical properties[1-4]. However, wider applications would be obtained only if its low flexural strength (150 MPa) and low fracture toughness (1.8 MPa·m1/2) could be improved. Many strategies have been developed to improve the mechanical properties of mullite ceramics such as adding ZrO2 component[5-7] and dispersing SiC particles[8], carbon nanotubes[9] and other micro-or nanoparticles in the mullite ceramic matrix as reinforcing phases.
Dispersing metastable tetragonal zirconia (t-ZrO2) particles in a mullite matrix is a well-known and relatively cheap route to reinforce mullite[6-7]. The principles of the reinforcement are based on the existence of tetragonal zirconia in which the phase transformation toughening effect could be resulted. Particularly, by adding stabilizing agents, transformation of tetragonal zirconia (t-ZrO2) to monocline zirconia (m-ZrO2) in cooling process could be prohibited and much better toughening effect could be obtained[6-8, 10-11].
Many other unconventional ways have been used to prepare zirconia-mullite ceramics[12-14]. In this work, ultra-fine zirconia-mullite composite ceramics were prepared by in-situ controlled crystallization of Si-Al-Zr-O amorphous bulk[15-16]. This method can avoid aggregation of nano-size starting powder, and receive higher densification which is hard to be achieved by nano-sized powder sintering. MONICA et al[17] has ever reported the crystallization of quenched Al2O3- ZrO2-SiO2 glasses but failed to receive high quality composite ceramics with high performance.
The purpose of this work is to report the processing and characterization of zirconia-mullite ceramics prepared by the new method. Mechanical properties, microstructure and the crystallization behavior were also evaluated.
2 Experimental
2.1 Samples preparation
The batch powder contented 30%-45% SiO2, 30%-40% Al2O3, 10%-25% ZrO2 (mass fraction) and a small amount of MgO, CaO, etc. They were mixed and homogenized by conventional ball milling with zirconia balls for 10 h. Then 20 g of mixed powders were put into Al2O3 crucible which was heated in air at temperatures in the range of 1 620-1 700 ℃ for 2-4 h in an electric furnace. The homogeneous flux was thereafter poured into a stainless mold and cooled with liquid nitrogen to produce amorphous bulks. Afterward, the as-received amorphous bulks were first treated at 900-1 000 ℃ for nucleation, then treated at higher temperature for crystallization to obtain nano-composite zirconia- toughened mullite ceramics. Samples numbered as Z15, Z18 and Z20 indicated zirconia mass fraction of 15%, 18% and 20%, respectively.
2.2 Characterization
Fracture toughness was determined for all the samples using a Vicker’s hardness tester with a Vicker’s indentor and a load of 9.8 N (MICRODUROMAT4000). Flexural strength was tested in three points bending on 3 mm×4 mm×35 mm, using a 30 mm span and a crosshead speed of 0.5 mm/min(CSS-44100). The reported values were the average data obtained from four tests of samples with the same raw powder composition and heat-treating procedure.
Phase compositions were measured by X-ray diffraction using Japanese D/MAX 2500VB instrument in a step-scanning mode with Ni-filtered Cu Kα as the radiation source and the radiation was over a range of 10?-80?. The volume fractions of tetragonal zirconia (φt) were calculated by the following equations[18]:
φt=1-φm (1)
where φm is the volume fraction of m-ZrO2, which can be calculated by
φm=
(2)
where Xm is the integrated intensity ratio, and P =1.340.
Xm=
(3)
where Im and It are the peak heights of m-ZrO2 and t-ZrO2, respectively.
After crystallization, the bulk density of samples was measured using the Archimedes’ technique. The microstructure was examined by a Siri-on200s canning electronic microscope(SEM). The bulk samples for SEM testing were etched by 1% (volume fraction) hydrofluoric acid-water solution after being polished and washed 3 times with deionized water. The crystallization status of t-ZrO2 was examined by selected-area electron diffraction to powder samples using a Tecnai G220 S-TWIN transmission electron microscope(TEM).
3 Results and discussion
3.1 Effects of ZrO2 content and heat-treatment temperature on mechanical properties
Fig.1 shows the flexural strength and fracture toughness data of samples Z15, Z18 and Z20 treated at 1 150 ℃ and 1 200 ℃ for 1 h crystallization, respectively. From the results, it can be found that the mechanical properties decrease with the increasing of heating temperature. Samples heat-treated at 1 150 ℃ for 1 h have better mechanical properties than those treated at 1 200 ℃, but with an exception of the fracture toughness of Z18. Sample Z15 heat-treated at 1 150 ℃ has the conditional optimized properties with 520 MPa of flexural strength and 5.13 MPa?m1/2 of fracture toughness, respectively. The flexural strength is 40% higher than that of the zirconia-toughened mullite ceramics which are fabricated by conventional means [10]. This suggests that in order to obtain better mechanical properties, it is better to control the heat-treatment temperature and let it not exceed 1 150 ℃. From Fig.1, it also can be found that the mechanical properties decrease with the increasing content of ZrO2 in starting materials after the content of ZrO2 exceeds 15%. This means that the further increase of zirconia after 15% ZrO2 addition has little help for the mechanical property improvement. That is, the optimum content of ZrO2 in starting materials should not be over 15%.
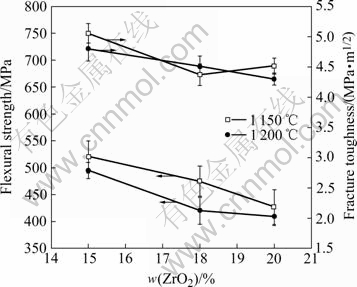
Fig.1 Mechanical properties of samples Z15, Z18 and Z20 treated at different temperatures
3.2 Phases analysis
Fig.2 shows the X-ray diffraction patterns of samples Z15, Z18 and Z20 treated at different temperatures. The nucleation test results at 900 ℃, 1 000 ℃ for Z20 sample are also given. The precipitated phases at different crystallization temperatures are listed in Table 1.
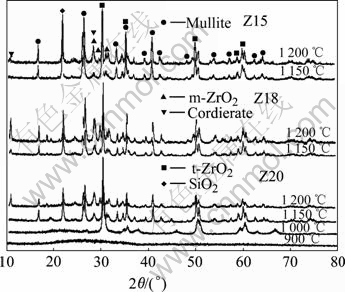
Fig.2 XRD patterns of samples Z15, Z18 and Z20 treated at different temperatures
Table 1 Phase components of sample Z20 treated at different temperatures
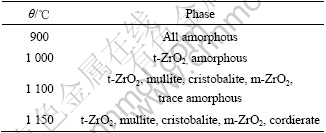
Sample Z20 was still amorphous pre-heated at 900 ℃. When increasing the temperature up to 1 000 ℃, the small amount of t-ZrO2 was precipitated firstly. When the temperature increased up to 1 100 ℃, the mullite, cristobalite and m-ZrO2 were precipitated, but there was still trace glass phase existing. When the heating temperature was higher than 1 150 ℃, for all samples there were no more new phases generated. The details for the crystallization have been discussed in our previous works[15-16].
Fig.3 shows the SEM micrograph of sample Z20 treated at 900 ℃. The sample had many voids after being etched by hydrofluoric acid-water solution. This means the phase separation occurred in the amorphous bulk, for the preparation of new phase crystallization. Fig.4 shows the TEM dark field micrograph of sample Z20 treated at 1 000 ℃. The bright particles are ZrO2 with the grain size less than 50 nm. The selected area diffraction spots for bright particles are inserted at the right corner, and the analysis to the diffraction spots shows that ZrO2 is of the tetragonal crystal structure (t-ZrO2). This is in agreement with XRD test results, which are shown in Fig.2. In order to promote the crystallization process for mullite and other new phases, it is better to increase the heat-treating temperature, such as up to 1 150 ℃ or 1 200 ℃. The experimental results indicate that no new phases are formed beyond 1 150 ℃ heat treatment. Although a higher temperature, such as 1 200 ℃, is helpful for speeding up the new phase crystallization from amorphous matrix, it is much harmful to the mechanical properties of this new materials due to the specific structure change, which will be discussed later on. This negative effect is indicated in Fig.1.
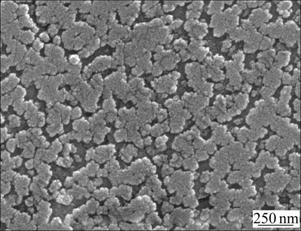
Fig.3 SEM micrograph of Z20 pre-treated at 900 ℃
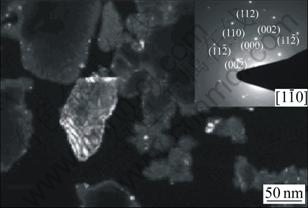
Fig.4 TEM dark field micrograph of Z20 powder heat-treated at 1 000 ℃
The calculated volume fractions of tetragonal zirconia (t-ZrO2) in samples treated at 1 150 ℃ and 1 200 ℃ according to Eqns.(1)-(3) are shown in Table 2. The results show that the heat-treatment temperatures and ZrO2 content in starting materials have important effects on the ZrO2 phase structure. With the increasing of heat treating temperature, the volume fractions of t-ZrO2 have dramatic reduction by about 13.9%, 16.7% and 22.7% for samples Z15, Z18 and Z20, respectively. This means that the same volume fraction of t-ZrO2 is transformed into m-ZrO2 correspondently, since the higher temperature accelerates the t-ZrO2 grain growth process, and results in the size of some t-ZrO2 grains to be over the t→m transformation critical size. From Table 2, it also can be found that the t-ZrO2 fraction increases with the increasing zirconia content at 1 150 ℃. This attributes to the fact that, with the increase of ZrO2 addition, there is more small size t-ZrO2 particles precipitated from the amorphous matrix. However, for samples treated at 1 200 ℃, the t-ZrO2 volume fraction decreases with the increasing of zirconia content. This drop of the t-ZrO2 volume fraction is resulted from the faster growth of the small size t-ZrO2 particles. Some of t-ZrO2 particles with over critical size are transformed into m-ZrO2. For sample Z20 treated at 1 200 ℃, due to both effects of the higher heat-treatment temperature and higher ZrO2 addition in the starting materials, the t-ZrO2 volume fraction drops to the lowest point 57.2%. This is the main reason why the mechanical properties for the sample treated at 1 150 ℃, on the whole, are better than those of the Z20 sample treated at 1 200 ℃, just as shown in Fig.1.
Table 2 Volume fractions of t-ZrO2 of samples Z15, Z18 and Z20 heat-treated at different temperatures (%)
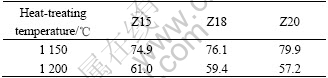
3.3 Microstructure
The average densities of samples Z15, Z18 and Z20 are given in Table 3. The densities increase with both the increasing of ZrO2 content and heat-treating temperature. Since the best mechanical properties are obtained by sample Z15 treated at 1 150 ℃, with a relatively low density, there should be other structural factors to play important roles in the determination of the mechanical properties, except for the density and the volume fractions of t-ZrO2.
Table 3 Average densities of samples heat-treated at 1 150 ℃and 1 200 ℃, respectively (g/cm3)
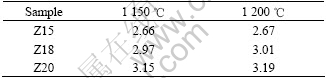
Fig.5 shows the back-scattered scanning electron micrographs of samples Z15 and Z18, treated at 1 150 ℃ and 1 200 ℃ for 1 h, respectively. As marked in images, the bright colour particles are ZrO2 grains, and the dark or gray areas are other phases. It can be found that the sample Z15 heat-treated at 1 150 ℃ contained a lot of nano-size ZrO2 grains, only about 60-80 nm. This is very important for obtaining the better mechanical property. The second obvious characteristic in Fig.5(a) is that there are a lot of micro-cracks in this new ceramic composite. These micro- cracks will give their contribution to the improvement of the mechanical properties by micro-cracking toughening mechanism. There are also some voids in the matrix, which are filled with amorphous phase before the sample is etched by 1% (volume fraction) hydrofluoric acid-water solution. On the other hand, with the increasing of the heat treatment temperature, say 1 200 ℃, the grains grow significantly. Some of ZrO2 grains approach 230 nm, just as shown in Fig.5(b). According to Table 2, there are 13.9% t-ZrO2 particles to be transformed into m-ZrO2. The third obviously structural change caused by the increasing of the heat treatment temperature is the decrease of micro-cracks in the matrix, compared with the sample Z15 treated at 1 150 ℃. These two important structural changes result in the mechanical property difference for sample Z15 treated at 1 150 ℃ and 1 200 ℃.
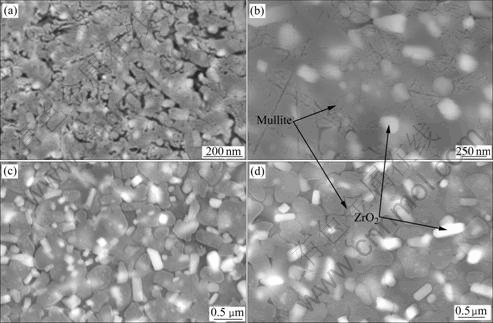
Fig.5 SEM micrographs of samples Z15, Z18 and Z20 treated at different temperatures: (a) Z15, 1 150 ℃, 1 h; (b) Z15, 1 200 ℃, 1 h; (c) Z18, 1 150 ℃, 1 h; (d) Z18, 1 200 ℃, 1 h
Figs.5(c) and 5(d) show the SEM micrographs of sample Z18 heated at 1 150 ℃ and 1 200 ℃, respectively. With the increasing of the heat treatment temperature, the average grain size of ZrO2 particles increases from 200 nm in Fig.5(c) to 500 nm in Fig.5(d). This results in 16.7% t-ZrO2 particles to be transformed into m-ZrO2 according to Table 2. On the other hand, it can be found that there are much less micro-cracks in the matrix, compared with sample Z15 treated at 1 150 ℃ and 1 200 ℃, as shown in Figs.5(a) and 5(b). The flexural strength drops by about 10%, and fracture toughness drops by about 20%.
Comparing Fig.5(a) with Fig.5(c), it can be found that the zirconia addition in starting materials has significant influence on microstructure for this new ceramic composite. In sample Z18, both the ZrO2 and mullite grains are of much larger grain size than in sample Z15. The largest ZrO2 and mullite grains approach 500 nm approximately. In addition, the increase of zirconia addition obviously decreases the micro- cracks in the matrix. These structural changes result in the mechanical property difference for samples Z15 and Z18 treated at 1 150 ℃, as indicated in Fig.1. The third important aspect is the effect of the zirconia addition in starting materials on the ZrO2 and mullite grain morphology. The ZrO2 grains in the samples contained less than 15% zirconia are near equiaxed, as shown in Fig 5(a), but in higher zirconia content samples, like Z18, the ZrO2 and mullite grains are of rod-like shape. The t-ZrO2 volume fractions decrease markedly when the samples contain more rod-like grains, as seen in Table 2. This is because the rod-like t-ZrO2 grains are easily grown beyond the critical size of tetragonal-monocline transformation. This process will be accelerated by both the higher heat treating temperature and higher zirconia addition in starting materials. The mechanical properties decrease linearly with the decrease of t-ZrO2 volume fraction at 1 200 ℃. Therefore, equiaxed ZrO2 grains are more effective in toughening mullite matrix. The conditional optimum content of zirconia should not excess 15%.
4 Conclusions
1) The main phases are zirconia and mullite. Zirconia starts to precipitate at around 1 000 ℃ in t-ZrO2 phase state from the Si-Al-Zr-O amorphous bulks, and mullite at about 1 100 ℃.
2) The crystallization heat treatment temperature and the zirconia addition in starting materials have great effect on the structure and mechanical properties. The Z15 sample treated at 1 150℃ for crystallization has developed a unique structure, in which there are a lot of near equiaxed t-ZrO2 grains and micro-cracks, and has the best mechanical properties. The flexural strength and fracture toughness are 520 MPa and 5.13 MPa·m1/2, respectively.
3) Either higher zirconia addition or higher crystallization temperature would lead a larger size rod-like ZrO2 and mullite grains to be developed, which has negative effect on mechanical properties of this new composite ceramics.
References
[1] MEDVEDOVSKI E. Alumina-mullite ceramics for structural applications[J]. Ceramics International, 2006, 32: 369-375.
[2] LAN Feng-tao, LI Ke-zhi, LI He-jun, FU Qian-gang. A cordierite- mullite anti-oxidation coating forcarbon/carbon composite [J]. Carbon, 2007, 45: 2692-2716.
[3] ZHANG H Y, MALJKOVIC N, MITCHELL B S. Structure and interfacial properties of nanocrystalline alumina/mullite composites [J]. Mater Sci Eng A, 2002, 326: 317-323.
[4] ANANTHAKUMAR S, JAYASANKAR M, WARRIER K G K. Microstructural, mechanical and thermal characterisation of sol-gel derived aluminium titanate-mullite ceramic composites [J]. Acta Materialia, 2006, 54: 2965-2973.
[5] KHOR K A, YU L G, LI Y. Spark plasma reaction sintering of ZrO2-mullite composites from plasma spheroidized zircon/alumina powers [J]. Mater Sci Eng A, 2003, 339(12): 286-296.
[6] JIN Xi-hai, GAO Lian, KAN Yan-mei, CHEN Yu-ru, YUAN Qi-min. Influence of Nb2O5 on the mechanical performances and toughening mechanism of ZrO2 in ZTM-Al2O3 [J]. Journal of Inorganic Materials, 2000, 15(6): 1009-1014.
[7] EBADZADEH T, GHASEMI E. Effect of TiO2 addition on the stability of t-ZrO2 in mullite-ZrO2 composites prepared from various starting materials [J]. Ceramics International, 2002, 28(4): 447-450.
[8] HONG J S, HUANG X X, GUO J K, LI B S, GUI L H. Strengthening and toughening of mullite ceramics by SiC particles and Y-TZP [J]. J Inorg Mater, 1990, 5(4): 340-345.
[9] WANG Jing, KOU Hua-min, LIU Xue-jian, PAN Yu-bai, GUO Jing-kun. Reinforcement of mullite matrix with multi-walled carbon nanotubes [J]. Ceramics International, 2007, 33: 719-722.
[10] HUANG Yang-feng, XIE Gen-sheng, XIAO Han-ning. The influence of CeO2 in ZTM ceramics prepared by in-situ sintering [J]. Ceramics, 2006, 6: 9-11. (in Chinese)
[11] GARRIDO L B, AGLIETTI E F. Reaction-sintered mullite-zirconia composites by colloidal processing of alumina-zircon-CeO2 mixtures [J]. Mater Sci Eng A, 2004, 369(12): 250-257.
[12] GARRIDO L B, AGLIETTI E F, MARTORELLO L, CAMERUCCI M A, CAVALIERI A L. Hardness and fracture toughness of mullite–zirconia composites obtained by slip casting [J]. Mater Sci Eng A, 2006, 419: 290-296.
[13] ZHAO S K, HUANG Y, WANG C A, HUANG X X, GUO J K. Sinterability of ZrSiO4/α-Al2O3 mixed powders [J]. Ceram Int, 2003, 29: 49-53.
[14] MAITRA S, RAHAMAA N, SARKA A R, TARAFDAR A. Zirconia-mullite materials prepared from semi-colloidal route derived precursors [J]. Ceramics International, 2006, 32: 201-206.
[15] LIANG Shu-quan, LI Shao-qiang, TAN Xiao-ping, TANG Yan, ZHANG Yong. Crystallization behavior of Si-Al-Zr-O amorphous bulk [J]. The Chinese Journal of Nonferrous Metals, 2005, 15(8): 1189-1193. (in Chinese)
[16] TAN Xiao-ping, LIANG Shu-quan, LI Shao-qiang, TANG Yan. Preparation of ZrO2-mullite nano-composite ceramics [J]. J Cent South Univ, 2005, 36(5): 790-794. (in Chinese)
[17] MONICA P, JOSE M, CALDERON M. Crystallization of gel-derived and quenched glasses in the ternary oxide Al2O3-ZrO2-SiO2 system [J]. J Non-cryst Solids, 2002, 297: 290-300.
[18] TORAYA H, YOSHIMURA M, SOMIYA S. Calibration curve for quantitative analysis of the monoclinic-tert ragonal ZrO2 system by X-ray diffraction [J]. J Am Ceram Soc, 1984, 6(2): 112-119.
Foundation item: Project supported by Foundation for Innovative Research Groups of China; Project(50634060) supported by the National Natural Science Founation of China
Corresponding author: LIANG Shu-quan; E-mail: lsq@mail.csu.edu.cn
(Edited by LI Xiang-qun)