J. Cent. South Univ. (2016) 23: 2402-2409
DOI: 10.1007/s11771-016-3299-2

Study on “triangle” water-inrush mode of strong water-guide collapse column
LI Zhen-hua(李振华)1, FENG Guo-rui(冯国瑞)2, ZHAI Chang-zhi(翟常治)1
1. School of Energy Science & Engineering, Henan Polytechnic University, Jiaozuo 454000, China;
2. College of Mining Technology, Taiyuan University of Technology, Taiyuan 030024, China
Central South University Press and Springer-Verlag Berlin Heidelberg 2016
Abstract: The water-inrush mechanism of strong water-guide collapse column in coal seam is studied based on the establishment of geological and mathematical models of “triangle” water-inrush mode. The geological background of Shuangliu mine is considered a prototype, similar simulation tests are adopted to analyze the water-inrush rules under this model, and the formation of water-guide channel and water-inrush process is investigated by examining the changes in rock resistivity. This work also uses the coupled cloud image derived from numerical simulation software to verify the results of simulation test. Results show that the numerical simulation of “triangle” water-inrush mode is consistent with the similar simulation. The “triangle” seepage area, which is located at the bottom of collapse columns and is connected to aquifer, is caused by the altered seepage direction and strengthened seepage actions after the overlapping of hydraulic transverse seepage in collapse column and hydraulic vertical seepage flow in aquifer. Under “triangle” water-inrush model, water-guide channel is formed by the communication between plastic failure zone of working face baseplate and “triangular” seepage area. Accordingly, the threatening water-inrush distance between working face and collapse column increases by 20 m compared with that of theoretical calculation.
Key words: strong water-guide collapse column; “triangle” water-inrush mode; similar simulation; numerical simulation; seepage
1 Introduction
Karst collapse column is a geological phenomenon with distinctive regional features and is widely developed in the coal field area of North China. This structure is generated by continuously rising collapse of large-span caverns in coal underlying karst cave system [1-2]. Collapse columns can be divided into fully-filled strong water-guide collapse column, edge-filled water- guide collapse column, and non-water-guide collapse column [3]. Among them, the fully-filled strong water-guide collapse column has the largest water-inrush threat for coal mining. Many scholars conducted considerable research on the formation mechanism, development characteristics, water-inrush mechanism, and water-inrush prediction of fully-filled strong water- guide collapse column [4-7]. Considering the water- inrush mode of collapse column, YIN [8] proposed two water-inrush modes based on the positional relationship between working face or tunnel and collapse column. These modes are sidewall water-inrush mode and top- bottom water-inrush mode. Furthermore, other modes were introduced, including thick-walled cylinder water-inrush mode, thin plate theory water-inrush mode, shear failure theory water-inrush mode, and fracture water-inrush mode; and established a theoretical criterion [9-10].
Given its complex geological background and irregular fillings, achieving the physical simulation of collapse column is quite difficult. Hence, many scholars conducted considerable numerical simulation research. WANG and YANG [11] adopted FLAC3D to simulate the process of destroying collapse column in coal seam floor, and considered that mining will first affect floor and will then affect collapse column. SONG et al [12] adopted numerical simulation to establish the mechanical criteria for thick-walled cylinder water-inrush mode. YIN and WU [13] used simulated working face to promote the whole process, and considered that optimal state of shear failure can be reached when the collapse column wall, working face plate compression zone, and the dividing line of expansion area are on the same line; and that heaving floor water inrush is more prone to occur. Based on these studies, the author extends another water-inrush mode according to sidewall water-inrush mode, because the traditional sidewall water-inrush criterion cannot ensure mining safety under this mode. Based on the theoretical analysis, this work adopts similar simulation, numerical simulation, and mutual corroboration to explore the water-inrush mechanism of this water-inrush mode.
2 “Triangle” water-inrush mode based on sidewall fracturing water-inrush mode
2.1 Geological model
The geological model of “triangle” water-inrush mode is shown in Fig. 1. It is shown that the collapse column is a proven strong water-filled water collapse column, and the aquifer can provide full supplement. The surrounding fractures of collapse column are well- developed, and thus, seepage belt with a certain thickness is formed. Thick impermeable layers exist between the mining failure zone of coal seam floor and the rising belt of confined water, and the front side of working face and the seepage belt of collapse column retain coal pillars that are long enough. Under such circumstance, the largest water-inrush threat for mining space comes from the joint effect of mining damage area and “triangle” failure zone in front of working face. “Triangle” failure zone is located at the intersection of rising belt of confined water and seepage belt of collapse column. In this intersection, the seepage action is strengthened through the superposition of horizontal and vertical seepage fields. The seepage direction is also changed, and thus, a “triangle” failure zone is formed. This zone reduces the relative thickness of impermeable layer of floor and protective pillar of collapse column, and increases the risk of water inrush.
2.2 Mathematical model
Sidewall fracturing water-inrush mode is a sub-mode of collapse column sidewall water-inrush mode. The horizontal section of fracturing water-inrush mode is shown in Fig. 2. It is shown that the water within the collapse column connects other structures through hydrofracture and causes water inrush. When using this mode, main consideration should be given to the water pressure within collapse column. Assuming that the rock mass is homogeneous, linear elastic, and has the same nature, the liquid in collapse column and aquifer follow Darcy’s law when flowing in the gap between rocks. A principal stress direction is present in the rock, which is parallel to the axis of collapse column. Based on the principle of hydraulic fracturing, the mechanical model of collapse column can be simplified as a plane problem. Based on elastic mechanics analysis, this problem is equivalent to the action of the maximum principal stress σ1 and the minimum principal stress σ2 on round hole with radius of α on an infinite plate. The stress of any point M around the hole is
(1)
(2)
(3)
where σr is the radial stress of point M; σθ is the tangential stress of point M; θ is the internal friction angle; τrθ, τθr are shear stresses of point M; r is the distance between point M and the center of hole; when r=α, the round hole wall is in the stress state.
According to Eqs. (1)-(3), the concentrated stresses of points A and B in Fig. 2 are expressed as
(4)
(5)
The external water pressure pc that can cause extension fracture of column wall is called critical rupture pressure. Critical rupture pressure is the total sum of concentrated stress at column wall rupture and tensile strength of rock T minus pore pressure in rock p0, that is,
(6)
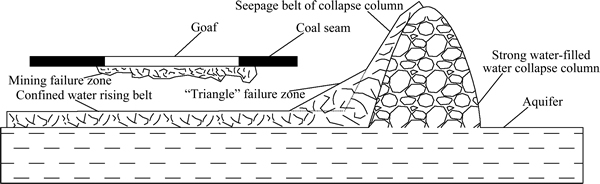
Fig. 1 Geological model of “triangle” water-inrush mode
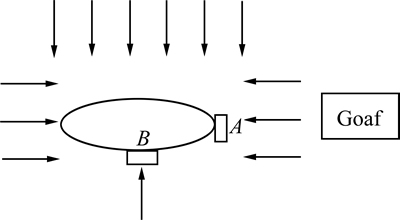
Fig. 2 Horizontal section of fracturing water-inrush mode
3 Similar simulation test
3.1 Establishment of model
The test selects the geological conditions of Shuangliu mine as test prototype, and adopts plane stress to test model frame. The length, width, and height of model frame are 2.5 m, 0.2 m, and 1.6 m, respectively; the bottom adopts the hydraulic loading system developed by China University of Mining and Technology [14-16] to simulate the pressure of confined water. The simulated water pressure can increase pressure on plate and penetration erosion. A hydraulic jack is adopted to compensate stress for the stratums that are not simulated. To ensure the accuracy and stability of the test, the dimension scale of selected model is 1:100.
3.2 Similarity theory
The following similarity ratios are determined according to similar simulation test principle and the actual situation.
1) Geometric similarity coefficient (geometric ratio) of model:
(7)
where Ym is the dimension of model; Yp is the dimension of prototype.
2) Time similarity coefficient:
(8)
where tm is the process time of model; tp is the process time of prototype.
3) Bulk density similarity coefficient:
(9)
where γm is the bulk density of model, and is set as 1.5×104 N/m3; γp is the bulk density of prototype, and is set as 2.5×104 N/m3.
4) Elastic modulus ratio:

where ar is bulk density similarity coefficient; aL is geometrical ratio.
3.3 Model layout and arrangement of measuring points
According to the prototype geologic column and mechanical parameters of rock layers, this work draws reference from the proportioning of similar materials, and adopts similar materials for layout to meet the requirements. Similar compressive strengths of simulation materials and original rock are shown in Table 1. Collapse column is a non-continuous layer, and its internal filling materials have strong water-filling and water-guiding performance. In this test, collapse column is filled with differently sized crushed stone to ensure that the properties of simulated collapse column are consistent with those of the prototype.
Model measuring points are shown in Fig. 3. Five resistivity survey lines are arranged on the coal seam floor, which are a, b, c, d, and e. The spacing between survey lines is 10 m, and the spacing between a survey line and coal seam floor is 5 m. Each survey line has five measuring points. The spacing between every two measuring points is 10 m, and the rightmost measuring point of each survey line is 10 m away from the left edge of collapse column. Built-in electrode is set in the measuring point to measure the changes in rock stratum resistivity.
Table 1 Compressive strength of similar simulation materials and original rock
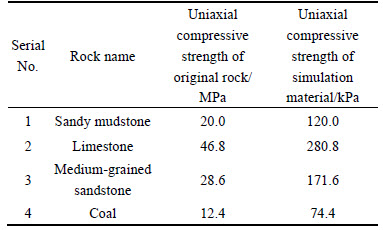
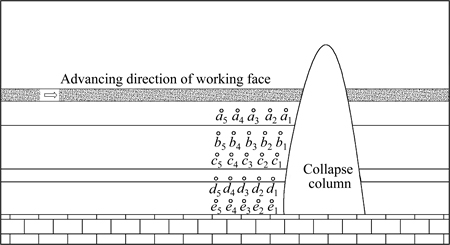
Fig. 3 Diagram of model measuring points
Coal pillars of 25 m are set on both sides as boundary coal pillars. After calculating using empirical formula, the length of coal pillar protected by collapse column is 30 m. The advancing direction of working face is shown in Fig. 3. The resistivity of each measuring point is measured before mining working face and increasing pressure to model. After applying pressure and stabilizing water seepage, the resistivity of measuring points should be measured again. When the working face is advanced for every 10 m, the interval of advancing time should be 4 h. When the rock stratum and water seepage become stable after mining, the resistivity and displacement of each measuring point are measured.
3.4 Analysis of similar simulation results
The resistivity curve of measuring point is shown in Fig. 4. The zero in the horizontal axis of Fig. 4 represents the resistivity of each measuring point before increasing water pressure. Survey lines are arranged in different rock stratums: d survey line is arranged in limestone and has the largest initial resistivity, followed by b and c survey lines which are arranged in sandstone, and finally, a and e survey lines which are arranged in sandy mudstone. As shown in Fig. 4, the resistivity decreases when rock moisture increases. The comparison of resistivity of measuring points is used to predict the seepage scope of confined water and collapse column, and the generation point of working face water-guide channel. After applying water pressure, the resistivity of five measuring points shows synchronous decline. The resistivity of points d4, d5 in d survey line has no significant change, which indicates that rising belt of confined water appears after applying water pressure. The height of the rising belt is 10-20 m. As observed from curves a1, b1, c1, d1, and e1, resistivity is reduced by varying degrees when water pressure becomes stable. This finding indicates that collapse column forms a seepage field with certain width under the action of confined water. In addition, a2 and b2 do not have similar changes with a1 and b1, which indicates that collapse column width is 12-20 m in the initial seepage area.
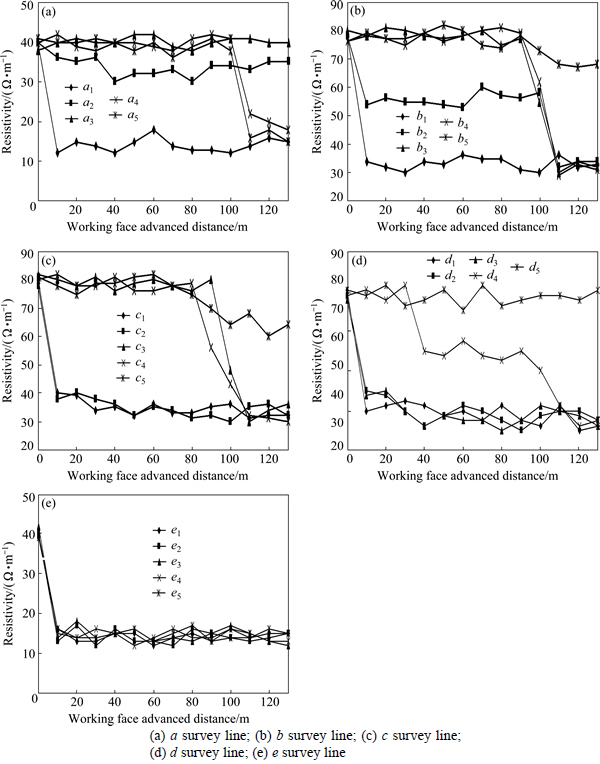
Fig. 4 Resistivity change curve of measuring point:
After the applied water pressure becomes stable, the resistivity of a1 point suddenly decreases, and the resistivity of a2 point remain unchanged; the resistivity of b1 point suddenly decreases, and the resistivity of b2 point slightly decreases; the resistivity of points c1 and c2 suddenly decreases, and the resistivity of c3 point is basically unchanged; the resistivity of points d1, d2, and d3 slightly decreases; the resistivity of point d4 is basically unchanged. As observed, a triangular initial seepage area is formed in the area of measuring points. This area is the superimposition of rising belt of confined water and seepage zone of collapse column. This area also broadens the two zones to a certain extent. The vertical seepage of confined aquifer overlaps with the lateral seepage of collapse column, and forms an overlapped seepage pointing at working face. Under this action, a triangle initial seepage area is formed.
Based on the calculation of water-inrush coefficient method, the rising belt of confined water cannot be connected with the plastic failure zone of floor mining, that is, they cannot form a water-guide channel, and thus, no threat of floor water inrush is formed. The preserved coal pillar of collapse column is calculated as 30 m. The internal pressure cannot break coal pillar and cannot form sidewall water-inrush threat within this distance.
In the process of advancing working face, the resistivity of each survey line shows that the resistivity of each measuring point changes slightly when working face is (70 m above) far away from collapse column. Mining basically exerts no impact on the seepage area of collapse column. When working face is advanced to 80 m (70 m away from collapse column), the resistivity of measuring points a4, a5, b3, b4, c3, and c4 gradually begins to decrease; when working face is advanced to 100 m (50 m away from collapse column), the infiltration rate of these measuring points reaches the resistivity under infiltration status. This finding indicates that the area that contains these points forms a water-guide channel. The on-site model shows that water begins to seep into the mined-out area from underneath the working face. Analysis shows that this water-guide channel is a “triangle” seepage area that connects with the plastic failure zone of working face. Compared with the results of theoretical Eqs. (1) and (3), the water-inrush occurs 20 m in advance.
4 Analysis of numerical simulation
FLAC3D numerical simulation software is adopted to simulate the “triangle” water-inrush mode of collapse column. The test also selects the geological conditions of Shuangliu mine as test prototype, simulates the physical parameters of rock stratums, as listed in Table 2, and uses software to simulate the initial seepage status of collapse column and artesian aquifer, and the coupled cloud images of plastic failure zone and seepage field of mining.
Table 2 Physical parameters of rock stratums
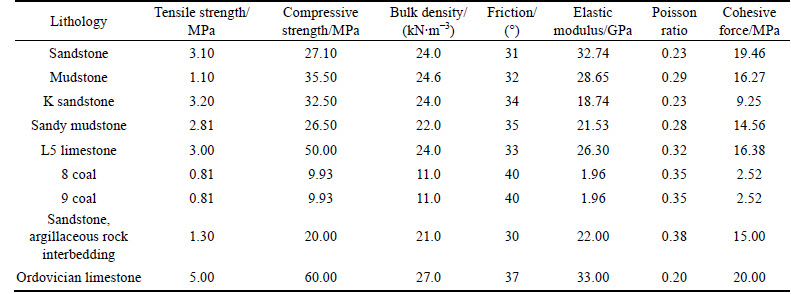
The initial seepage field of collapse column is shown in Fig. 5. The strong water-filling water-guide collapse column and surrounding fractures form a seepage field, and seepage intensity gets smaller when it gets far away from the center axis. The seepage intensity at the top of collapse column is significantly smaller than the seepage intensity at the bottom of collapse column. The simulation results indicate that the seepage scope of collapse column is 30 m away from aquifer, whereas the overlapped seepage area of bottom seepage area of collapse column and aquifer rising belt reaches 45-55 m. Accordingly, a distinct “triangular” seepage area is formed.
The coupling diagram when the distances between working face and collapse column are 70, 50, and 30 m is shown in Fig. 6. With the increase of working face,floor stress increases and decreases, and floor compresses and expands. The floor damage turns from stretching to shear failure, and damaged fractures of coal seam floor are generated during this process. If the damaged fractures are connected with the seepage zone of collapse column or rising belt of confined water, then water inrush will occur in the working face. As shown in Fig. 6(a), when the distance between working face and collapse column is 70 m, floor will form a 13 m-depth plastic damage area. The coal wall in front of working face will also form a plastic damage area. Within this distance, the damaged fractures of floor have no contact with aquifer rising belt and have no access to the “triangular” seepage zone. The plastic damage area in front of working face also does not come into contact with the “triangular” seepage zone. This distance is the safe mining distance, and thus, water inrush will not possibly occur.
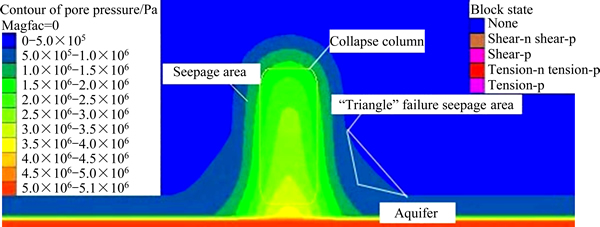
Fig. 5 Initial seepage field of collapse column
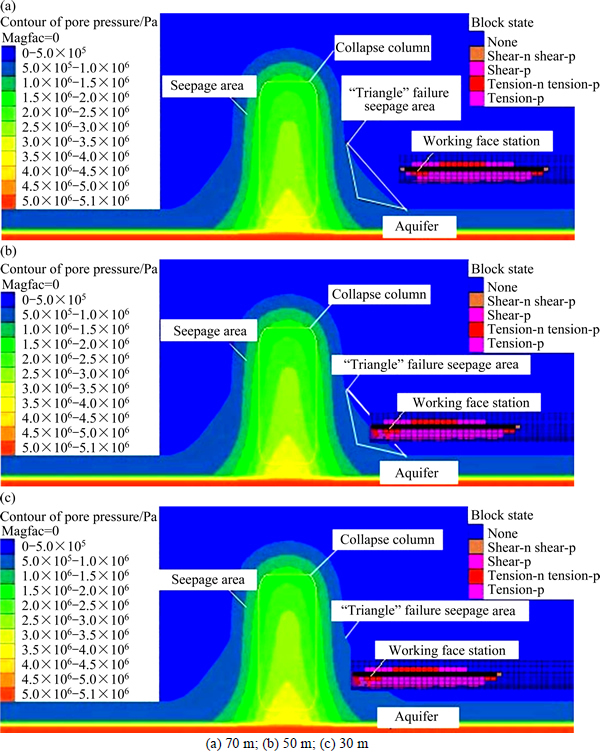
Fig. 6 Coupling diagram at different distances between working face and collapse column:
As shown in Fig. 6(b), when the distance between working face and collapse column is 50 m or further increases, the depth of plastic failure zone in front of working face increases. Moreover, the plastic failure zone at this position begins to come into contact with the “triangular” seepage zone, and thus, water inrush threat is formed. Assuming that no “triangular” seepage zone is present, the seepage zones on both sides of collapse column are its original seepage zone, and the upper part of aquifer is also the original water rising belt. As shown in Fig. 6(b), the damaged fracture zone of working face floor and its front plastic damage area do not connect with the original seepage zone of collapse column or the original water rising belt of confined aquifer. Accordingly, this distance is the safe mining distance according to the traditional computing method. In the process of preserving barrier pillar, designer should consider the existence of “triangular” seepage zone, that is, the width of barrier pillar should be increased.
As shown in Fig. 6(c), when the distance between working face and collapse column is 30 m, working face has completely entered the “triangular” seepage zone, and the damaged fracture zone of working face floor and its front plastic damage area enter the original seepage zone of collapse column. Water-guide channel is completely formed under this circumstance, and severe water inrush disaster will occur under water pressure.
5 Conclusions
1) By analyzing the geological model of water- inrush mode in the “triangular” seepage area of collapse column, this work establishes a “triangle” water-inrush mathematical model according to sidewall fracturing mode. This study also verifies that “triangular” seepage area is caused by the altered seepage direction and strengthened seepage actions after the overlapping of hydraulic transverse seepage in collapse column and hydraulic vertical seepage flow in aquifer.
2) Through simulation experiment of similar materials, the changes in rock resistivity are observed and are used to explore the position and formation process of water-inrush water-guide channel of collapse column. When “triangular” seepage area exists, the water-inrush location is 20 m smaller than the value derived from theoretical calculation, that is, the plastic failure zone of mining floor connects with the “triangular” seepage area, and forms a water-guide channel.
3) The results of numerical simulation are basically consistent with the results of similar material simulation. The initial seepage range of collapse column is approximately 30 m. The overlapped seepage area of bottom seepage area of collapse column and aquifer rising belt reaches 45-55 m. Water-inrush threat is formed when the working face is 50 m away from collapse column.
References
[1] YIN Shang-xian, WU Qiang, WANG Shang-xu. Water bearing characteristics and hydro-geological models of karstic collapse columns in North China [J]. Chinese Journal of Rock Mechanics and Engineering, 2005, 24(1): 77-82. (in Chinese)
[2] LI Zhen-hua, XU Gao-ming, LI Jian-bo. Research status and outlook of water-inrush from collapse column in China [J]. China Mining Magazine, 2009, 18(4): 107-109. (in Chinese)
[3] ZHONG Ya-ping. Study on comprehensive technology of water prevention in Kailuan Coal Mine [M]. Beijing: China Coal Industry Publishing Home, 2001. (in Chinese)
[4] ZHANG Mao-lin, YIN Shang-xian. Forming process of subsided column in coal fields of North China [J]. Coal Geology and Exploration, 2007, 35(6): 26-29. (in Chinese)
[5] WANG Jing-ming, LIU Wen-sheng, GUAN Yong-qiang. Mechanism of groundwater inner circulation in sinking column formation in North China coal field—A case study at Fengfeng mine [J]. Carsologica Sinica, 2007, 26(1): 11-17.
[6] LIU Deng-xian, LI Yong-jun. Morphological characters and hydrological geology characters of cambrian karstic collapse column in Kongji mining area, Huainan [J]. Coal Geology of China, 2006, 18(4): 38-40. (in Chinese)
[7] LI Yong-jun, PENG Su-ping. Classifications and characteristics of karst collapse columns in North China coalfields [J]. Coal Geology and Exploration, 2006, 34(4): 53-56. (in Chinese)
[8] YIN Shang-xian. Analysis, simulation and application of water inrush system in mine area [D]. Beijing: China University of Mining and Technology, 2002: 59-82. (in Chinese)
[9] YIN Shang-xian, WU Qiang, WANG Shang-xu. Hydrogeological and mechanical basics of water inrush from karstic collapse columns in Northern China [J]. Journal of China Coal Society, 2004, 29(2): 182-185. (in Chinese)
[10] YIN Shang-xian, WU Qiang. Simulation and mechanism analysis of water inrush from karstic collapse columns in cola floor [J]. Chinese Journal of Rock Mechanics and Engineering, 2004, 23(15): 2551- 2556. (in Chinese)
[11] WANG Jia-chen, YANG Sheng-li. Numerical simulation of mining effect on collapse column activated water conducting mechanism [J]. Journal of Mining & Safety Engineering, 2009, 26(2): 140-144. (in Chinese)
[12] SONG Yan-qi, WANG Xing-yu, CHENG Peng. The mechanical criterion and numerical simulation of thick-walled elliptical cylinder collapse column model under water inrush [J]. Journal of China Coal Society, 2011, 36(3): 452-455. (in Chinese)
[13] YIN Shang-xian, WU Qiang. A numerical simulation analysis of influence of karstic collapse columns on rock mass yield and water inrush from coal floor [J]. Journal of China Coal Society, 2003, 28(3): 264-269. (in Chinese)
[14] WANG Jia-chen, LI Jian-bo, XU Gao-ming. Development and application of simulation test system for water inrush from the water-conducting collapse column [J]. Journal of Mining & Safety Engineering, 2010, 27(3): 305-309. (in Chinese)
[15] LI Zhen-hua, LI Jian-bo, HE Zhi-hong. Analysis on development characteristics of Karst collapse column and water inrush risk in Shuangliu coal mine [J]. Journal of Mining & Safety Engineering, 2014, 31(1): 84-89. (in Chinese)
[16] LI Zhen-hua, XIE Hui, LI Jian-bo. Experimental study of mining effect on collapse column activated water conducting mechanism [J]. Journal of Central South University: Science and Technology, 2014, 45(12): 4377-4383. (in Chinese)
(Edited by DENG Lü-xiang)
Foundation item: Projects(51374093,51104058) supported by the National Natural Science Foundation of China; Project(2013CB227903) supported by the National Basic Research Program of China
Received date: 2015-11-25; Accepted date: 2016-03-17
Corresponding author: LI Zhen-hua, PhD, Associate Professor; Tel: +86-391-3987132; E-mail: jzlizhenh@163.com