
Nanoscale Ni-B amorphous alloy catalyst supported on carbon nanotubes
HU Chang-yuan(胡长员)1, 2, DUAN Wu-mao(段武茂)3, NING Yun-long(宁云龙)1,
WU Zhen-fei(伍振飞)1, LI Feng-yi(李凤仪)2
1. Jiangxi Key Laboratory of Surface Engineering, Jiangxi Science and Technology Normal University, Nanchang 330013, China;
2. School of Science, Nanchang University, Nanchang 330047, China;
3. Institute of Technology, Jiangxi Agriculture University, Nanchang 330045, China
Received 15 July 2007; accepted 10 September 2007
Abstract: Heat treatment of carbon nanotubes (CNTs) was carried out under ammonia atmosphere and then CNTs were modified by Triton x-100 (CNTs-T). Ni-B amorphous alloy catalysts supported on CNTs and CNTs-T were prepared by impregnation-chemical reduction method. The catalysts were characterized by TEM, ICP, XRD, BET and CO chemisorption, and studied in the acetylene selective hydrogenation. The results show that homogeneous Ni-B amorphous particles with mean size about 10 nm are successfully prepared on CNTs-T. Compared with Ni-B/CNTs, nickel loading of Ni-B/CNTs-T is increased by about 14.6%. Furthermore, the activity and selectivity of Ni-B/CNTs-T are much higher than those of Ni-B/CNTs in the acetylene selective hydrogenation under comparative condition.
Key words: carbon nanotubes; nanoscale Ni-B amorphous alloy; catalytic materials; Triton x-100
1 Introduction
Carbon nanotubes are severely hydrophobic and can be essentially bundled in solvent. These properties are generally considered as a significant barrier in both fundamental research and technological development toward the practical uses of CNTs. Thus, the functionalization of CNTs has received much attention for overcoming above-mentioned problems in recent years[1-2]. Many functionalization reactions reported in literatures were based on the covalent amidation or esterification of nanotube-bound carboxylic acid groups[3-4], which are associated with defects on as prepared and acid treated nanotubes. Additionally, the functionalization has also been utilized at the ends and/or walls of CNTs noncovalently[5-6] to attach certain functional groups onto nanotubes, improving hydrophilicity and dispersion properties of nanotubes in solvent.
SHIM et al[7] have reported that the adsorption of surfactant Triton x-100 (C14H22O(C2H4O)n) on SWNTs is effective in preventing nonspecific adsorption of streptavidin on nanotubes via hydrophobic interactions between the nanotubes and streptavidin (a kind of protein). Since the adsorption of Triton can prevent effectively the hydrophobic interactions between the nanotubes and protein, it is believable that Triton can be adsorbed onto CNTs as a wetting layer to enhance the hydrophilic property of CNTs[8]. So, it is desirable that nanotubes modified by Triton are the favorable parent materials for fabricating nano-composites. For these reasons, Triton x-100 was used to modify the surface of ammonia-treated CNTs[9] for preparing nanoscale Ni-B amorphous alloy catalyst supported on nanotubes. As a new class of advanced materials for catalytic applications, carbon nanotubes used as catalyst supports induce peculiar activity and selectivity in catalytic reactions, such as selective oxidation of H2S into elemental sulfur and selective hydrogenation of nitrobenzene into aniline[10]. In comparison with other Ni-based catalysts, Ni-B amorphous alloy catalysts exhibit higher activity and better selectivity, which have been widely studied by researchers in the past two decades[11]. Therefore, it is expected that the nanoscale Ni-B amorphous alloy particles supported on nanotubes can be a potentialcandidate for highly active hydrogenation catalyst by combination the merits of carbon nanotubes and amorphous alloy. The present work aimed at preparing well-dispersed Ni-B amorphous alloy nanoparticles supported on nanotubes and studying the catalytic performance in acetylene selective hydrogenation.
2 Experimental
Carbon nanotubes were prepared by catalytic decomposition of methane on Ni-Cu-Al catalyst[12]. As-grown nanotubes were carried out ammonia heat treatment (The final product is labeled as CNTs)[9]. Adsorption of Triton x-100 on CNTs was conducted according to previous work (The final product is labeled as CNTs-T)[13]. Ni-B/CNTs, Ni-B/CNTs-T amorphous alloy catalysts were prepared by impregnation-chemical reduction method elsewhere[13-14].
TEM observation was performed on a Hitachi H600 electron microscope. The Ni loading and bulk compositions of amorphous alloy were analyzed by inductively coupled plasma (ICP). The total surface area (SBET) was measured by N2 adsorption at 77 K. The surface area of active nickel (SNi) was determined by CO chemisorption. XRD analysis was executed on a Rigaku D/MAX-RA powder diffractometer to determine the amorphous structure of nanotubes supported Ni-B catalysts.
100 mg catalysts were loaded into the micro-reactor and then reduced by H2 in situ at 493 K for 30 min. After that, the gaseous reactant (V(C2H2)?V(H2)?V(N2)=2?4?94) flowed into the micro-reactor at a rate of 50 mL/min. The reactant and product were analyzed with an online gas chromatograph equipped with TCD, using a porapak N column.
3 Results and discussion
3.1 Characterization of Ni-B/CNTs catalysts
As shown in Fig.1(a), carbon nanotubes treated by ammonia entangle each other and most Ni-B alloys are in the form of highly aggregation except for a few high dispersion particles on nanotubes. Adsorption of Triton x-100 on carbon nanotubes treated by ammonia, however, results in relatively individual nanotubes and homogeneous Ni-B particles with mean size about 10 nm on nanotubes, as shown in Fig.1(b).
In order to confirm the amorphous structure of prepared catalysts, XRD measurement was performed. As shown in Fig.2, the XRD patterns of carbon nanotubes indicate the graphite structure of prepared sample. Cubic nickel was still presented in carbon nanotubes after they were treated with ammonia. The nickel could be assigned to the Ni residue from the Ni-
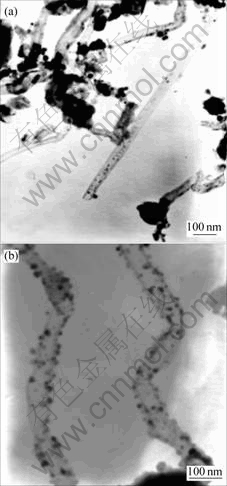
Fig.1 TEM images of catalysts: (a) Ni-B/CNTs; (b) Ni-B/ CNTs-T
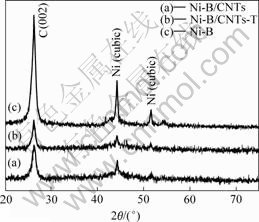
Fig.2 XRD patterns of samples
Cu-Al catalyst, used to synthesize CNTs. From this, it is likely that nickel residue in CNTs is difficult to remove by heat treatment with ammonia. Compared with the patterns of CNTs, the patterns of Ni-B/CNTs and Ni-B/CNTs-T do not exhibit other crystalline peaks, which means the amorphous structure of prepared catalysts[14].
The bulk composition, Ni-loading, total surface area and active surface area of the fresh catalysts are listed in Table 1. Ni-loading is increased approximately by 14.6% through adsorption of Triton x-100 on carbon nanotubes treated by ammonia. The reason may be that modification of CNTs with Triton has effects on the stabilization of nanotubes suspension and the wetting behavior of nanotubes surface. These changes facilitate the adsorption of nickel ions onto nanotubes surface and then increase Ni-loading. The surface area of CNTs decreases from 99.8 m2/g to 88.7 m2/g after the CNTs are decorated by Triton, which means that the Triton is partially incorporated into the channel of nanotubes. However, the surface area of supported Ni-B amorphous catalysts increases from 81.7 m2/g (Ni-B/CNTs) to 93.3 m2/g (Ni-B/CNTs-T). In addition, the surface area of active nickel increases from 19.8 m2/g (Ni-B/CNTs) to 29.9 m2/g (Ni-B/CNTs-T). The enhancement of SBET and SNi for Ni-B/CNTs-T can be attributed to the high dispersion Ni-B amorphous particles on CNTs-T, which is consistent with the TEM results.
Table 1 Some characteristics of the samples determined by ICP, BET and CO chemisorption
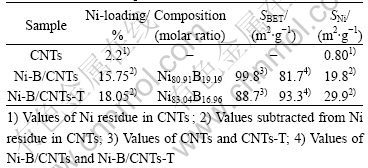
3.2 Catalytic performance
As shown in Fig.3, Ni-B/CNTs-T is more active than Ni-B/CNTs in the acetylene selective hydrogenation. It is understandable that higher dispersion of active components and nickel loading of Ni-B/CNTs-T promote the acetylene hydrogenation. Additionally, on the whole, the ethylene selectivity of Ni-B/CNTs-T is higher than that of Ni-B/CNTs over a wide range of equivalent acetylene conversion (Fig.4). In acetylene hydrogenation, the reaction proceeds via three major routes[15-16]. Route one is the hydrogenation of acetylene to ethylene followed by desorption from the surface or further hydrogenation. Route two is the hydrogenation of acetylene to intermediates, such as ethylidyne, which do not yield ethylene but are directly hydrogenated to ethane. Route three is the polymerization of ethylene to produce polymer that eventually covers the surface leading to the catalyst deactivation. Considering the reaction routes of acetylene hydrogenation, we may explain the selectivity improvement of Ni-B/CNTs-T in the reaction as follows. As discussed in the TEM section, uniform Ni-B amorphous alloy particles form on CNTs modified by Triton. This indicates that Ni-B highly spreads on the surface of nanotubes and the existence of multiple coordinated sites of nickel is greatly reduced. Undoubtedly, the well-dispersed Ni-B on nanotubes suppresses Routes two and three so that the production of ethane and polymer is decreased and the ethylene selectivity is improved[15-17]. Therefore, the combination of homogeneous Ni-B particles and higher Ni-loading resulted in the better catalytic performance of Ni-B/CNTs-T catalyst.
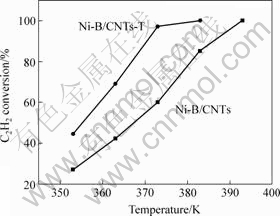
Fig.3 Activity of acetylene selection hydrogenation
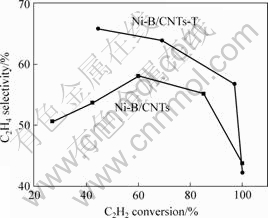
Fig.4 Changes in ethylene selectivity with conversion in acetylene hydrogenation at 353-393 K
4 Conclusions
1) Uniform Ni-B amorphous particles with mean size about 10 nm were successfully prepared on CNTs-T.
2) Nickel loading is increased by 14.6% through modification nanotubes treated by ammonia with Triton.
3) The activity and selectivity of Ni-B/CNTs-T are much higher than those of Ni-B/CNTs in the acetylene selective hydrogenation under comparative condition.
References
[1] HUANG W J, LIN Y, TAYLOR S, GAILLARD J, RAO A M, SUN Y P. Sonication-assisted functionalization and solubilization of carbon nanotubes[J]. Nano Lett, 2002, 2: 231-234.
[2] DIMITRIOS T, KOSTAS P, MAURIZIO P, IOANNIS K, CONSTANTINOS G. Water-soluble carbon nanotubes by redox radical polymenrization[J]. Macromol Ripid Commun, 2007, 28: 1553-1558.
[3] HUANG W J, FERNANDO S, LIN Y, ZHOU B, ALLARD L F, SUN Y.P. Preferential solubilization of smaller single-walled carbon nanotubes in sequential functionalization reactions[J]. Langmuir, 2003, 19: 7084-7088.
[4] QIN Y J, SHI J H, WU W, LI X G, GUO Z X, ZHU D B. Concise route to functionalized carbon nanotubes[J]. J Phys Chem B. 2003, 107: 12899-12901.
[5] KANG Y J, TATON T A. Micelle-encapsulated carbon nanotubes: a route to nanotubes composites[J]. J Am Chem Soc, 2003, 125: 5650-5651.
[6] CARRILLO A, SWARTZ J A, GAMBA J M, KANE R S. Noncovalent functionalization of graphite and carbon nanotubes with polymer multilayers and gold nanoparticles[J]. Nano Lett, 2003, 3: 1437-1440.
[7] SHIM M, KAM N W S, CHEN R J, LI Y M, DAI H J. Funtionalization of carbon nanotubes for biocompatibility recognition[J]. Nano Lett, 2002, 2: 285-288.
[8] GONG X Y, LIU J, BASKARAN S, VOISE R D, YOUNG J S. Surfactant-assisted processing of carbon nanotubes/polymer composites[J]. Chem Mater, 2000, 12: 1049-1052.
[9] JIANG L Q, GAO L. Modified carbon nanotubes: an effective way to selective attachment of gold nanoparticles[J]. Carbon, 2003, 41: 2923-2929.
[10] NHUT J M, PEASANT L, TESSONNIER J P. Mesoprous carbon nanotubes for use as support in catalysis and nanosized reactors for one-dimensional inorganic material synthesis[J]. Appl Catal A Gen, 2003, 254: 345-363.
[11] ZHANG R B, LI F Y, ZHANG N, SHI Q J. Benzene hydrogenation over amorphous NiB/bentonite catalyst and promoting effect of Nd[J]. Appl Catal A Gen, 2003, 239: 17-23.
[12] JU Y, LI F Y, WEI R Z. Catalytic growth of carbon nanotubes with large inner diameters[J]. J Serb Chem Soc, 2005, 70(2): 277-282.
[13] HU C Y, LI F Y, ZHANG R B, LIAO X N, HUA L. Effects of neodymium on the properties of Ni-B/CNTs amorphous alloy catalyst[J]. J Chin Chem Soc, 2007, 54: 559-562.
[14] XU S X, LI F Y, WEI R Z. Preparation of novel RuB amorphous alloy catalyst supported on carbon nanotubes[J]. Carbon, 2005, 43: 861-864.
[15] KANG J H, Shin EW, KIM W J, Park J D, MOON S H. Selective hydrogenation of acetylene on Pd/SiO2 catalysts promoted with Ti, Nb and Ce oxides[J]. Catal Today, 2000, 63: 183-188.
[16] KANG J H, Shin EW, KIM W J, Park J D, MOON S H. Selective hydrogenation of acetylene on TiO2-added Pd catalysts[J]. J Catal, 2002, 208: 310-320.
[17] KIM W J, KANG J H, AHN I Y. Effect of potassium addition on the properties of a TiO2-modified Pd catalyst for the selective hydrogenation of acetylene[J]. Appl Catal A Gen, 2004, 268: 77-82.
(Edited by HE Xue-feng)
Foundation item: Project (20263003) supported by the National Natural Science Foundation of China
Corresponding author: HU Chang-yuan; Tel: +86-791-3831266; E-mail:hcychem@hotmail.com