
One-step pickling-activation before magnesium alloy plating
WANG Xin-juan(王新娟)1, YU Gang(余 刚)1, OUYANG Yue-jun(欧阳越军)1, 2,
HE Xiao-mei(何晓梅)3, ZHANG Jun(张 俊)1, YE Li-yuan(叶立元)1
1. State Key Laboratory of Chemo/Bisensing and Chemometrics, College of Chemistry and Chemical Engineering,
Hunan University, Changsha 410082, China;
2. Department of Chemistry and Chemical Engineering, Huaihua College, Huaihua 418000, China;
3. Department of Chemistry and Chemical Engineering, Hunan Institute of Technology, Hengyang 421002, China
Received 7 March 2008; accepted 21 May 2008
Abstract: A one-step pickling-activation process was proposed as an environmental friendly pretreatment method in phosphate-permanganate solution before electroplating on magnesium alloys. The effects of pickling-activation on qualities of coating were assessed by adhesion and porosity testing of copper plating. The interfacial reactions between specimen and solution were analyzed with SEM, EDX and XRD. The results show that the developed process of pickling-activation can equalize the potentials on substrate surface. The compacted zinc film can be obtained by zinc immersion after treating magnesium alloy in the pH 4-6 phosphate-permanganate solution for 3-5 min. The adhesion and corrosion resistance of copper plating are enhanced. The one-step pickling-activation can replace the existing two-step process of acid pickling and activation which contains a great deal of chromium and fluorine. The procedure of surface pretreatment is simplified and the production environment is improved.
Key words: magnesium alloy; pickling-activation; pretreatment; electroplating; corrosion resistance
1 Introduction
Magnesium alloy has the unique characteristics, such as low density, high specific strength and other good physical and mechanical properties, which make it valuable in a number of applications as automobile and computer parts, aerospace components, mobile phones, sporting goods, handheld tools and household equipment[1-4]. However, the relatively poor corrosion resistance limits its extensive utilization[5-9]. Thus, it is necessary to form anti-corrosion coatings on the surface of magnesium alloy in practical applications.
Among various surface treatment techniques, electroplating is of special interest due to its advantages such as low cost, good corrosion and wear resistance, good electrical and thermal conductivity, and good solderability[10-11]. However, the electroplating on magnesium alloy has many challenges in processing of plating[12]. The most difficult part of plating on magnesium alloy is the development of an appropriate pretreatment process. Once a suitable undercoating is in place, many desired metals can be plated. General process for treating magnesium alloy prior to plating is alkaline cleaning→acid pickling→activation→zinc immersion→copper cyanide electroplating[13-15]. Many reports are on improvement of copper cyanide electroplating[14-16]. The magnesium alloy was etched in a solution of chromium oxide and nitric acid. Then it was soaked in HF solution to form a conversion film before zinc immersion. Nevertheless, metal finishing industries have to look for alternative materials or special deposition methods to replace the chromium compounds, which are progressively restricted due to their high toxicity to environment. HF also exhibits strong corrosion that cannot be easily controlled. Thus, the environmental and health friendly technology has been extensively studied to inhibit effectively the magnesium alloy corrosion[17-19]. Moreover, copper deposition from a pyrophosphate solution is developed to replace the copper cyanide electroplating after the zinc immersion[20]. In our previous study, a copper deposition on AZ91D magnesium alloy was proposed from a pyrophosphate solution[20] after the alloy was pickled in the acid solution containing chromium trioxide and activated in HF solution, followed by zinc immersion.
In the present work, a phosphate-permanganate solution without chromium and fluorine was investigated for pickling-activation of die-cast AZ91D magnesium alloy. The effects of pickling-activation on qualities of copper coating were discussed and the interfacial reactions between specimen and solution were analyzed.
2 Experimental
2.1 Experimental materials
Die-cast AZ91D magnesium alloy was used as the testing material, the chemical composition of which was listed in Table 1. Prior to experiment, the testing specimens were cut into the pieces of 30 mm×25 mm ×1.5 mm. The surface of specimens was first ground with 600 grade SiC paper, then polished with metallographical papers.
2.2 Process flow
The process flow of alkaline cleaning→pickling- activation→zinc immersion was used to treat the surface of specimens prior to plating. The operating conditions of the process and the compositions of solution are given in Table 2.
2.3 Testing procedures
2.3.1 Porosity evaluation
The coverage of copper plating was evaluated by observing the corrosion spots through immersion in 5% NaCl solution for 2 h. The NaCl solution could enlarge pinhole on the surface of specimens in short time. If there is porous copper plating on magnesium alloy surface, strong galvanic corrosion on the porous surface will take place owing to a large standard potential difference between Zn (-0.76 V) and Cu (+0.34 V). Copper plating as cathode of the corrosion cell is protected, but the uncovered zone on magnesium alloy substrate as anode is fiercely dissolved so that the corroded holes appear clearly.
2.3.2 Adhesion detection
Evaluation of the adhesion was conducted according to Chinese Standard GB/T5270—85. A rigid steel blade with acute angle of 30? was used to score square grids of 1 mm×1 mm on the plating. Adequate pressure was brought to bear on the blade to lacerate the plating to fundus in order to observe whether the plating is sloughed off during scraping and peeled off by gluing- tearing or not.
2.3.3 Characterization of surface properties of plating
An ordinary three-electrode cell was used to measure the open circuit potential curves of magnesium alloy in phosphate-permanganate solution and the polarization curves of the plating in 5% NaCl solutions at room temperature. The mass change of specimen was measured by electron analytical balance (Shanghai Hengping Scientific Instrument Co., Ltd, Model FA2104). The microstructure and composition of the specimen were characterized with SEM-EDX (JEOL, Model JSM-6700F). The phases in the activation film were analyzed by XRD (Rigaku, Model D/max-rA, Cu Kα).
Table 1 Chemical compositions of die-cast AZ91D magnesium alloy (mass fraction, %)

Table 2 Compositions of pretreatment solution and operating conditions
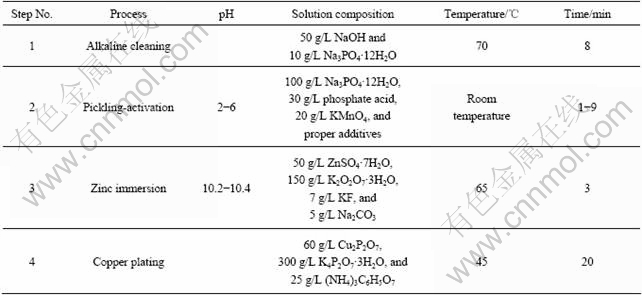
3 Results and discussion
3.1 Substrate surface by one-step pickling-activation treatment
Fig.1(a) shows the SEM image of the naked substrate without any pretreatments. The white dendrite structures display β phase composed of an intermetallic compound with the stoichiometric composition of Mg17Al12, and α phase is mainly composed of magnesium. Nucleation during solidification results in considerable variation in the distribution of aluminum and zinc in the microstructure of AZ91D alloy, leading to the asymmetrical distribution of the surface potential. The potentials of α and β phase are measured to be -1.58 and -0.99 V by MATHIEU et al[21], respectively, which makes the process of electroplating complicated. However, α phase and β phase of the alloy are not clearly demonstrated in Fig.1(b) after pretreatment by steps 1 and 2 shown in Table 2 owing to the chemical dissolution of the surface of matrix in pickling-activation process. The surface of specimen is covered with an activation film that resembles the phosphate conversion coating. Therefore, the distribution of surface potential on the substrate of magnesium alloy becomes more symmetric by pickling-activation treatment.
In order to explain the potential variations on specimen surface with the formation of activation film, the open circuit potential(φOC) curve of the specimen immersed in phosphate-permanganate solution at room temperature is shown in Fig.2. The open circuit potential starts at -0.96 V owing to the formation of alkaline film during the alkaline cleaning. Once the specimen is immersed in the phosphate-permanganate solution, the alkaline film is dissolved due to the reaction with acid in the solution. The electrode potential moves to negative direction quickly up to the lowest point B, -1.48 V, when the specimen is immersed for 50 s, as shown by segment AB in Fig.2. As demonstrated by segment BC in Fig.2, the activation film forms and makes the potential ascend rapidly. When arriving at point C, -1.41 V, the potential goes up slowly. After the specimen is immersed for 500 s, the potential reaches point D, hereafter, keeps constant, which indicates that a new steady activation film is formed. The new film demonstrates the discontinuous distribution of network-like cracks, as shown in Fig.1(b), which contribute to the subsequent zinc immersion treatment. The potential of -1.36 V is the steady potential for AZ91D magnesium alloy in solution. Therefore, the thermodynamic stability of the substrate surface of the specimen is improved by pickling-activation treatment.
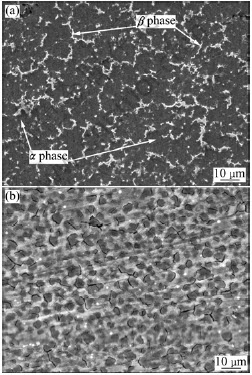
Fig.1 SEM images of die-cast AZ91D: (a) Naked substrate; (b) Substrate after pickling-activation
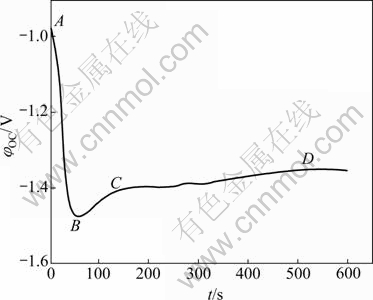
Fig.2 Dependence of φOC on immersion time in phosphate- permanganate solution
3.2 Interfacial reactions between specimen and solution
The phosphate-permanganate solution is composed of Na3PO4·12H2O, KMnO4, inorganic acid and additives. Hence, the hydrolysis reaction of phosphate happens in the solution as follows:
PO43-+H+→HPO42- (1)
HPO42-+H+→H2PO4- (2)
H2PO4-+H+→H3PO4 (3)
Consequently, a buffering system is formed to maintain the pH value of solution in a constant range. The alkaline film formed in the operating step 1 shown in Table 2 has the poor corrosion resistance due to loose and soluble porous structure. Therefore, it must be removed to form a new compacted protective layer on the substrate. When the specimen is immersed in the phosphate- permanganate solution, hydroxide and oxide films on the surface of the specimen are dissolved due to the reaction with H+ in the solution. In this case, the reactions between the interface of the specimen and solution can be expressed as follows:
Mg(OH)2+2H+→Mg2++2H2O (4)
MgO+2H+→Mg2++2H2O (5)
Then many micro-corrosion cells are formed due to asymmetrically distributed potentials. The electro- chemical corrosion occurs. The β phase with the higher potential as the cathode of the micro-cells is protected, while the α phase with the lower potential as the anode is ceaselessly dissolved. The possible reactions at the micro-anode zones are
Mg→Mg2++2e φ0= -2.36 V (6)
Al→Al3+ + 3e φ0 = -1.66 V (7)
Zn→Zn2++2e φ0= -0.74 V (8)
Based on the standard electrode potentials, the tendency of Eq.(6) is the largest among these reactions. Magnesium is the major element which dissolves and releases metal ions, aluminum is the second and there is not resolved zinc[22] in the micro-anode zones on the Mg-Al-Zn alloy surface. Therefore, the reaction (8) is difficult to occur. The reaction (7) becomes the major reaction form in the micro-anode zones.
Because many oxidizers such as hydrogen ions and permanganate ions exist in the solution, the possible reactions occurring at the micro-cathode zones include
2H++2e=H2 φ=0 V (9)
MnO4-+8H++5e=Mn2++2H2O, φ=1.51 V (10)
MnO4-+4H++3e=MnO2+2H2O, φ=1.725 4 V (11)
According to Pourbiax diagram (Fig.3)[23] for the Mn-H2O system, MnO4- cannot be directly reduced into Mn2+ when the pH value of the solution is in the range of 1-14. Therefore, reaction (10) is difficult to proceed in the system. A series of reactions of unstable MnO2 in the solution can happen when pH<7, including
MnO2+4H++2e=Mn2++2H2O, φ=1.239 V (12)
MnO2+2H++2e=Mn2O3+H2O, φ=0.967 5 V (13)
Mn2O3+2H++2e=2Mn3O4+H2O, φ=0.827 9 V (14)
Mn2O3+6H++2e=2Mn2++3H2O, φ=1.511 1 V (15)
Mn3O4+8H++2e=3Mn2++4H2O, φ=1.852 8 V (16)
Mn2++2e=Mn, φ= -1.191 V (17)
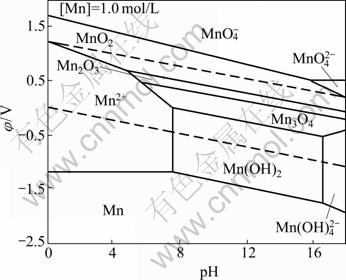
Fig.3 φ—pH diagram of Mn-H2O system
The trend for reaction (17), i.e. the divalent manganese ions deoxidized into metal Mn is small. Eqs.(9), (11), (12), (13), (14), (15) and (16) are the most possible reactions occurring in the micro-cathode zones. When the [Mg2+]3[PO43-]2≥Ksp=1.04×10-24, phosphate deposits are preferentially formed at the β phase zones. Some manganese oxides with low valence and low solubility such as MnO2, Mn2O3 and Mn3O4 are formed and deposited in the micro-cathode zones together with the phosphate deposits to form activation film. Then they act as the micro-cathode to promote the reactions progressing continuously until the basic film forms. The micro-anode zones dwindle.
The XRD patterns of the activation film with pickling-activation treatment for 10 s and 180 s are shown in Fig.4, which indicates that only Mg17Al12 phase and Mg phase in the specimen substrate are detected, and no other deposits are detected on the surface of substrate. However, the results of EDX in Table 3 show that activation film on the surface of specimens is composed of C, O, Na, Mg, Al, Zn, P, K and Mn, which indicates that other deposits may be formed on the surface of substrate. Therefore, it can be conjectured that the new activation film is the deposit with amorphous structures. According to the calculation of the percentage of elementary atoms and the analysis of interfacial reactions, the activation film may be composed of manganese oxides (MnO2, Mn2O3, Mn3O4) and other species such as Mg3PO4, MgO, Al2O3, C, Na3PO4, and K3PO4. The metal elements of Mg, Al, Zn should come from the substrate of magnesium alloy. The substrate oxides, such as MgO and Al2O3, can be replaced by Zn in the zinc immersion treatment. The manganese oxides (MnO2, Mn2O3, Mn3O4) and deposits of Mg3PO4, MgO, Al2O3, C, etc. protect the most zones of the substrate surface from being attacked by treating solution, enhancing the stability of substrate.
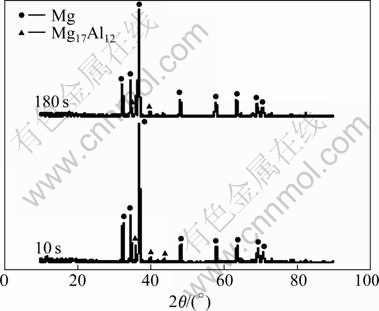
Fig.4 XRD patterns of activation film after pickling-activation treatment for 10 s (a) and 180 s (b)
Table 3 Elemental composition of specimen surface after pickling-activation (molar fraction, %)
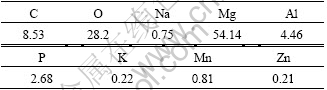
3.3 Effect of pH value and treating time on pickling- activation
As a new pretreatment process, operation parameters on the quality of pickling-activation ultimately influence the subsequent copper plating. The data of the parameters in the copper plating on AZ91D magnesium alloy are little reported. In the present work, the effect of pH value of the solution and time of pickling-activation have been investigated by detecting plating adhesion and plating corrosion resistance in order to obtain the optimum treating parameters.
3.3.1 Optimum pH value
The effect of the pH value of phosphate- permanganate solution on the quality of pickling- activation is shown in Table 4. The specimens were treated with the solution of different pH values based on steps 1-4 shown in Table 2. The results in Table 4 indicate that the optimum pH value of the solution is 4-6. The corrosion rate of the specimen in the solution of different pH values was measured to evaluate the effect of the pH value further, as shown in Fig.5. The specimens are eroded quickly when pH<3, at which there are sparsely free
and a large amount of hydrogen gas forms in the anode, hindering the deposit formation. So, it is difficult to form good activation film or the formed film is coarse and loose. The corrosion rate is very low when pH>6, at which the surface of the specimen cannot be attacked deficiently. A lot of dregs deposited on the substrate cannot be removed. Although the plating can be conducted after the zinc immersion, its adhesion and corrosion resistance are all poor. When pH>3, a certain protective film begins to produce, and the corrosion rate is reduced. In the pH values of 4-6, the corrosion reaction is diminished because equilibrium of the film formation and the film dissolution is built. When the stable activation film is formed, the subsequent copper plating can be satisfactorily carried out, as shown in Table 4.
Table 4 Performances of copper plating obtained for different pickling-activation pH
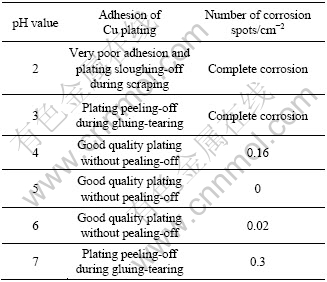
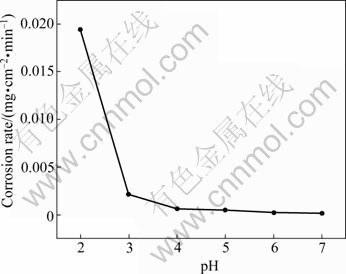
Fig.5 Effect of pH on corrosion rate of specimen
3.3.2 Optimum pickling-activation time
The effect of treating time on the quality of pickling-activation is shown in Table 5. The specimens were treated based on time shown by the steps 1-4 in Table 2. The optimum pickling-activation time ranges from 3 to 5 min. If time is less than 1 min, the activation film forms deficiently on the surface. The uncovered Mg due to the existence of many cracks reacts acutely with Zn2+ in the solution, which produces the loose zinc film. The adhesion is reduced between the subsequent plating and the substrate. Similarly, if the time exceeds 5 min, the amount of network-like cracks will decrease due to the thicker film, which reduces the active sites and hinders the formation of the zinc film. The activation film with optimum network-like cracks is formed in 3-5 min, which provides the reaction sites for replacement reaction to form uniform zinc film, as shown in Fig.6. Therefore, the copper plating with good adhesion and corrosion can be obtained.
Table 5 Performances of copper plating obtained for different pickling-activation time
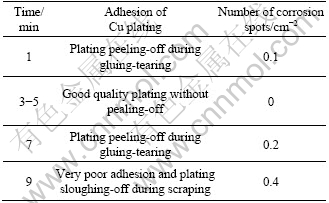
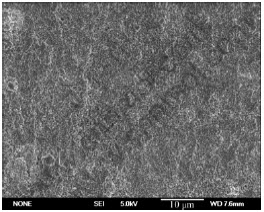
Fig.6 SEM image of film for zinc immersion after pickling- activation for 3 min
3.4 Corrosion resistance of copper plating obtained by two processes
Fig.7 shows the polarization curve (Curve 1) for the copper electroplating with the pretreatment in 5% NaCl solution. For comparison, the polarization curve (Curve 2) of the copper electroplating films on the substrate with Dow pretreatment[19] is also shown in Fig.7. It should be noted that both of the copper electroplatings have approximate thickness. The corrosion potential φcorr of the copper electroplating on the substrate with the developed pretreatment is more positive (0.065 V) than that (0.045 V) on the substrate with Dow pretreatment. In other words, the developed pretreatment process is more effective than Dow pretreatment for the substrate of magnesium alloy. Therefore, the corrosion resistance for the copper electroplating is improved by one-step pickling-activation pretreatment.
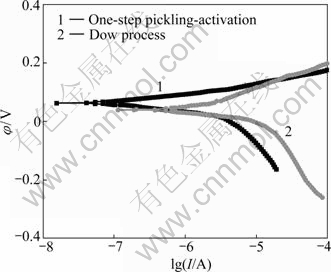
Fig.7 Potentiodynamic polarization curves of different copper platings
4 Conclusions
1) A phosphate-manganese solution was developed as the pickling-activation pretreatment solution for AZ91D magnesium alloy plating. The activation film formed in the solution enhances the thermodynamic stability of substrate and reduces the potential difference between the matrix phase and the second phase.
2) The optimum pH value of the phosphate- manganese solution is 4-6 and the optimum pickling- activation time is 3-5 min.
3) One-step pickling-activation pretreatment redounds to improve the corrosion resistance of the substrate and to obtain the copper plating with good adhesion and high coverage. The developed pretreatment is an environment friendly process, and can simplify the surface pretreatment as well as increase production efficiency.
References
[1] LIU Zheng, ZHANG Kui, ZENG Xiao-quan. Theory and application of magnesium alloys [M]. Beijing: China Machine Press, 2002: 1-10. (in Chinese)
[2] HOLLSTEIN F, WIIEDEMANN R, SCHOLZ J. Characteristics of PVD-coatings on AZ31hp magnesium alloys [J]. Surface & Coatings Technology, 2003, 162: 261-268.
[3] ZENG Rong-hang, KE Wei, XU Yong-bo, HAN En-hou, ZHU Zi-rong. Recent development and application of magnesium alloys [J]. Acta Metallurgica Sinica, 2001, 37(7): 673-685. (in Chinese)
[4] FUNATANIA K. Emerging technology in surface modification of light metals [J]. Surface & Coatings Technology, 2000, l33/l34: 264-272.
[5] J?NSSON M, PERSSON D, THIERRY D. Corrosion product formation during NaCl induced atmospheric corrosion of magnesium alloy AZ91D [J]. Corrosion Science, 2007, 49: 1540-1588.
[6] LI Ying, ZHANG Tao, WANG Fu-hui. Effect of microcrystallization on corrosion resistance of AZ91D alloy [J]. Electrochimica Acta, 2006, 51: 2845-2850.
[7] ZHANG Tao, LI Ying, WANG Fu-hui. Roles of β phase in the corrosion process of AZ91D magnesium alloy [J]. Corrosion Science, 2006, 48: 1249-1264.
[8] AMBAT R, AUNG N N, ZHOU W. Evaluation of microstructural effects on corrosion behaviour of AZ91D magnesium alloy [J]. Corrosion Science, 2000, 42: 1433-1455.
[9] ANDREI M, GABRIELE F, BONORA P L, SCANTIEBURY D. Corrosion behaviour of magnesium sacrificial anods in tap water [J]. Materials and Corrosion, 2003, 54: 5-11.
[10] JIANG Y F, LIU L F, ZHAI C Q, ZHU Y P, DING W J. Corrosion behavior of pulse-plated Zn-Ni alloy coatings on AZ91magnesium alloy in alkaline solutions [J]. Thin Solid Films, 2005, 484: 232-237.
[11] SONG Guang-ling, ATRENS A. Understanding magnesium corrosion—A framework for improved alloy performance [J]. Advanced Engineering Materials, 2003, 5: 837-857.
[12] GRAY J E, LUAN B. Protective coatings on magnesium and its alloys—A critical review [J]. Journal of Alloys and Compounds, 2002, 336: 88-113.
[13] JIANG Y F, ZHAI C Q, LIU L F, ZHU Y P. DING W J. Zn-Ni alloy coatings pulse-plated on magnesium alloy [J]. Surface & Coatings Technology, 2005, 191: 393-399.
[14] CHEN Jue-ling, YU Gang, HU Bo-nian, LIU Zheng, YE LI-yuan, WANG Zhen-feng. A zinc transition layer in electroless nickel plating [J]. Surface & Coatings Technology, 2006, 201: 686-690.
[15] GU Chang-dong, LIAN Jian-she, LI Guang-yu, NIU Li-yuan, JIANG Zhong-hao. Electroless Ni-P plating on AZ91D magnesium alloy from a sulfate solution [J]. Journal of Alloys and Compounds, 2005, 391: 104-109.
[16] YIN Jian-jun, LI Yuan-dong, LIANG Wei-dong, HAO Yuan. Investigation of pretreatment process with zinc-plating for magnesium alloy electroplating [J]. Journal of Gansu University of Technology, 2003, 29(1): 36-38. (in Chinese)
[17] WANG Xiao-min, ZHOU Wan-qiu, HAN En-hou. Electroplating zinc transition layer for electroless nickel plating on AM60 magnesium alloys [J]. Trans Nonferrous Met Soc China, 2006, 16(3): l798-1801.
[18] DABALà M, BRUNELLI K, NAPOLITANI E, MAGRINI M. Cerium-based chemical conversion coating on AZ63 magnesium alloy [J]. Surface & Coatings Technology, 2003, 172: 227-232.
[19] ZHAO Ming, WU Shu-sen, LUO Ji-rong, FUKUDA Y, NAKE H. A chromium-free conversion coating of magnesium alloy by a phosphate-permanganate solution [J]. Surface & Coatings Technology, 2006, 200: 5407-5412.
[20] YU Gang, LIU Yun-e, HU Bo-nian, LIU Zheng, YE Li-yuan. Investigation of electroplating copper in pyrophosphate on magnesium alloy [J]. Journal of Hunan University: Natural Science, 2005, 32(4): 77-81. (in Chinese)
[21] MATHIEU S, RAPIN C, HAZAN J, STEINMETZ P. Corrosion behaviour of high pressure die-cast and semi-solid cast AZ91D alloys [J]. Corrosion Science, 2002, 44: 2737-2756.
[22] SONG Guang-ling, ATRENS A, WU Xian-liang, ZHANG Bo. Corrosion behaviour of AZ21, AZ501 and AZ91 in sodium chloride [J]. Corrosion Science, 1998, 40: 1769-1791.
[23] ZHAO Zhong-wei, HUO Guang-sheng. Thermodynamic analysis of Li-Mn-H2O system [J]. China Nonferrous Metals, 2004, 24(11): 1296-1231 (in Chinese)
Corresponding author: YU Gang; Tel: +86-731-8822275; E-mail: yuganghnu@163.com
DOI: 10.1016/S1003-6326(08)60303-9
(Edited by YANG Bing)