
Mechanical properties of Mg-9Gd-4Y-0.6Zr alloy
XIAO Yang(肖 阳), ZHANG Xin-ming(张新明), CHEN Bu-xiang(陈部湘), DENG Zhen-zhen(邓桢桢)
School of Materials Science and Engineering, Central South University, Changsha 410083, China
Received 28 July 2006; accepted 15 September 2006
Abstract: The microstructures and mechanical properties of Mg-9Gd-4Y-0.6Zr alloy were investigated. The results show that the ultimate tensile strengths of the extrusion-T5 temper of this alloy at -196, 25, 250, 300 and 350 ℃ are as high as 521, 370, 348, 262 and 150 MPa, respectively. It is noteworthy that 8% plasticity occurs at -196 ℃ and 180% superplasticity occurs at 400 ℃. In the peak hardness of Cast-T5, Cast-T6, Ext-T5 and Ext-T6, the highest is that of Ext-T5. The prismatic precipitates providing an effective barrier to dislocation gliding on the basal plane are the cause of strengthening of this alloy. The plate-shaped precipitates formed on the prismatic planes provide the most effective barriers to the gliding dislocations, and they are the cause of strengthening of this alloy.
Key words: Mg-Gd-Y-Zr alloy; rare earth element; heat resistance; mechanical properties
1 Introduction
Magnesium alloys are quite attractive light structural materials, which are used in the aerospace, aircraft and automotive industries where low mass of the construction is of high importance. The demand of high enough strength is decisive for these alloys[1]. Rare earth elements have been used to raise the strength and heat resistance of magnesium alloys[2-3].
Magnesium alloys containing gadolinium and yttrium have notable effect on solution strengthening and precipitation strengthening, and the mechanical properties and creep resistance of Mg-9Gd-4Y-0.6Zr alloy are better than those of WE54 alloy[4], but these investigations were very limited[5]. In this study, the microstructures and mechanical properties at test temperatures ranging from -196 to 400 ℃ under different heat-treatment conditions of the alloy were investigated.
2 Experimental
Mg-9Gd-4Y-0.6Zr alloy was melted in an electrical resistance furnace by a mild steel crucible under a special flux. Alloying elements gadolinium, yttrium and zirconium were added in form of Mg-Gd, Mg-Y and Mg-Zr master alloys, respectively. The alloying temperature and pouring temperature are 750 and 730 ℃, respectively, and then the melt was poured into a steel mould and cooled in water. The actual composition (Mg-8.7Gd-4.1Y-0.4Zr, mass fraction, %) of this alloy was determined by atomic absorption spectroscopy.
The ingots were about 60 mm in diameter and 150 mm in length, which were solution treated at 520 ℃ for 8 h, then quenched in room temperature water, and extruded to rods of 15 mm in diameter at 440 ℃.
The ingots and rods were cut into pieces to test their Vickers hardness under selected various heat-treatment conditions. The mechanical properties were measured at -196-400 ℃ on Instron 8032 material test machine. The specimens were held in liquid nitrogen for 3 min (-196 ℃) or at elevated temperatures for 20 min at least before they started loading. The dimensions of all specimens were 6 mm in diameter and 36 mm in length.
The microstructures of the specimens were observed using poly var-met optical microscope, Sirion200 scanning electron microscope equipped with an energy dispersive X-ray spectrometer and Tecnai G2 20 transmission electron microscope.
3 Results and discussion
3.1 Microstructures of as-cast, solution-treated and as-extruded samples
The optical and SEM micrographs of this alloy are shown in Fig. 1, in which Fig.1(a) shows that a lot of equiaxed dendrites exist in the as-cast ingot, and the bright dendrites in Fig. 1(d) indicate that elements Gd and Y are susceptible to segregation during solidification.
After solution treatment, the grain size is about 50-80 μm, as shown in Fig.1(b), the dendrites have already vanished and the grain boundaries are clear. However, there are still a few black second-phases which can not be dissolved as shown in Fig.1(f), and the second-phases become white.
Fig.1(c) shows that the black second-phases are broken and the streamlines parallel to the extrusion direction form. Complete dynamic recrystallization occurs during the hot extrusion and the average grain size becomes fine and reduces to less than 20 μm.
Fig.1(g) clearly shows that the second-phases are in regular cubic structure in which high concentration Gd and Y elements were determined by EDAX analysis, and the mole ratio of Mg to Gd and Y is about 3?1. The second-phase may be Mg3X, because Gd and Y are mutual continuous solid solution[3]. The existence of second-phases reduces alloy elements solubility in magnesium matrix and the following precipitation, which maybe decreases the mechanical properties of this alloy.
3.2 Ageing characteristics and mechanical properties
The ageing curves of the different heat treatments at 225 ℃ are shown in Fig.2(a). Cast-T5 and Ext-T5 mean that the cast and the extruded specimens were directly aged, respectively, Cast-T6 and Ext-T6 indicate that the cast and extruded specimens were aged after solution treatment at 520 ℃ for 8 and 2 h, respectively. The hardness of the extruded specimen reaches the highest of all the examined alloys before ageing because the structures have the finest grains, which can cause fine-grained strengthening. It can be seen that the peak aged hardness of the Ext-T5 specimen is the highest and the time to peak hardness is the shortest, while that of the Cast-T5 specimen is inverse. The ageing curves of the Ext-T5 specimens aged at 200- 250 ℃ are shown in Fig.2(b). It can be found that the peak hardness and the corresponding time decrease with increasing temperature.
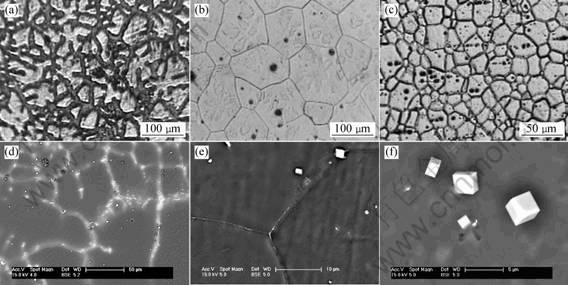
Fig.1 OM and SEM images of Mg-9Gd-4Y-0.6Zr alloy: (a), (d) As-cast; (b), (e) Solution-treated at 520 ℃ for 8 h; (c), (f) Longitudinal section of extruded rod
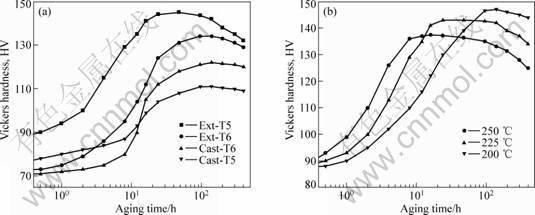
Fig.2 Ageing hardening curves of Mg-9Gd-4Y-0.6Zr alloy: (a) Ext-T5, Ext-T6, Cast-T6 and Cast-T5 samples aged at 225 ℃; (b) Ext-T5 samples aged at 250 ℃, 225 ℃ and 200 ℃
Table 1 lists the mechanical properties of Mg-9Gd-4Y-0.6Zr alloy, and it can be seen that the strength under Ext-T5 condition is the highest. Table 2 shows that the strength of Ext-T5 samples drops a little when tensile temperature increases from 25 to 250 ℃, which indicates good heat stability at these temperatures. The strength is still very high at 300 ℃, while it drops sharply at 350 ℃. The phenomenon of superplasticity occurred at 400 ℃ and plasticity became better at -196 ℃, the characteristic of heat resistance is very adapted to apply in space circumstance and the superplasticity to fabricate the complex parts in spacecraft. Compared with the mechanical properties of other magnesium-rare earth alloys such as WE54, WE43, HM31[6], the strength of the extruded Mg-9Gd-4Y-0.6Zr-T5 at various tempera- tures is markedly higher and so is the heat resistance.
Table 1 Mechanical properties of Mg-9Gd-4Y-0.6Zr alloy at room temperature after various heat treatments
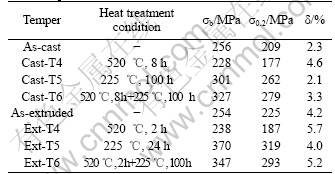
3.3 TEM observation of precipitates
According to the ageing curves in Fig.2(b), the precipitates in the Ext-T5 samples are observed. Fig.3(a) shows that white tiny precipitates are visible in the sample aged at 225 ℃ for 4 h (under aged). The patterns of the precipitates indicate that they have DO19 structure(β″ phase) which is coherent with magnesium matrix[7], and the hardness of the samples starts rapidly to increase with aging time.
Dense plate-like precipitates can be clearly seen in the sample peak-aged at 225 ℃ for 24 h. Figs.3(b) and (c) are the micrographs which are obtained with a beam parallel to [0001] and
zone axis, respectively. The diffraction patterns indicate the precipitates have base centred orthorhombic (bco) structure (β phase), which are semicoherent with the matrix[7]. The β′ precipitates have high volume fraction and internal fringe spacing are visible (Fig.3(b)). The precipitates formed on the prismatic planes of α-Mg matrix in interlocking triangular arrangement are the most effective obstacles to basal dislocation slip.
Table 2 Mechanical properties of Mg-9Gd-4Y-0.6Zr alloy under Ext-T5 condition at various tensile temperatures
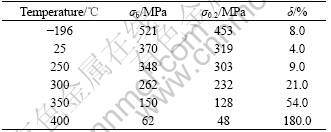
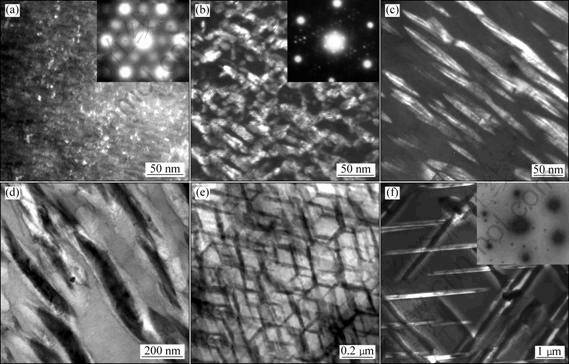
Fig.3 TEM images of Ext-T5 samples: (a) Aged at 225 ℃ for 4 h (B //[0001]); (b) Aged at 225 ℃ for 24 h (B //[0001]); (c) Aged at 225 ℃ for 24 h (B //
); (d) Aged at 225 ℃ for 240 h(B //
); (e) Held at 300 ℃ for 96 h after aged at 225 ℃ for 24 h (B //[0001]); (f) Held at 350 ℃ for 96 h after aged at 225 ℃ for 24 h(B//[0001])
The β′ phases grew up and were coarsened with aging time prolonging to 240 h at 225 ℃(over aged), as shown in Fig.3(d). The platelet size is about 50 nm in width and 500 nm in length, the amount of the precipitates decreases and inter-precipitate spacings become broad, which reduces the motion-impeding effect of dislocation and leads to the drop of mechanical properties. For checking the heat resistance, Figs.3(e) and (f) are corresponding microstructures of the Ext-T5 samples which were held at 300 and 350 ℃ for 96 h after they were aged at 225 ℃ for 24 h. The precipitates shown in Fig.3(e) has grown up to 200 nm in length, which shows that the precipitates still have high stability. But the precipitates in Fig.3(f) have grown up to 3-10 μm in length, and the diffraction pattern indicates that the precipitates have fcc structure (β equilibrium phase). The precipitation sequence ends with the transformation from metastable β″ and β′ phases to β equilibrium phase, and the mechanical properties and heat resistance sharply drop, the Vickers hardness of this sample at room temperature is 95 which is nearly equal to the value of the extruded sample, and this means that the effect of age-strengthening is disappear.
4 Conclusions
1) Mg-9Gd-4Y-0.6Zr alloy has excellent mechanical properties in the range of temperatures from -196 to 350 ℃. The 8% low-temperature plasticity at -196 ℃ and the 180% superplasticity at 400 ℃ are obtained.
2) As for the peak hardness of cast-T5, cast-T6, Ext-T5 and Ext-T6, the highest is for Ext-T5. The high volume fraction and fine scale plate-like metastable precipitates on the prismatic planes are the cause of strengthening and heat resistance.
References
[1] KOJIMA Y, AIZAWA T, KAMADO S. HRTEM observation of the precipitates in Mg-Gd-Y-Zr alloy[J]. Materials Science Forum, 2003, 419-422: 303-306.
[2] DRITS M E, SVIDERSKAYA Z A, ROKHLIN L L, NIKITINA N I.
Effect of alloying on the properties of Mg-Gd alloys[J]. Metallovedeniei Termicheskaya Obrabotka Metallov, 1979, 21(11): 62-64.
[3] DRITS M E, ROKHLIN L L, NIKITINA N I. State diagram of the Mg-Y-Gd system in the range rich in magnesium[J]. Izvestiya Akademii Nauk SSSR, Metally, 1983, 5: 215-219.
[4] ANYANWU I A, KAMADO S, KOJIMA Y. Aging characteristics and high temperature tensile properties of Mg-Gd-Y-Zr alloys[J]. Materials Transations, 2001, 42(7): 1206-1211.
[5] XIAO Yang, ZHANG Xin-ming, CHEN Jian-mei, JIANG Hao. Microstructures and mechanical properties of extruded Mg-9Gd-4Y-0.6Zr-T5 at elevated temperatures[J]. The Chinese Journal of Nonferrous Metals, 2006, 16(4): 709-714.(in Chinese)
[6] CAHN R W. Structure and Properties of Nonferrous Alloys[M]. DING Dao-yun transl. Beijing: Science Press, 1999: 109-114.(in Chinese)
[7] HONMA T, OHKUBO T, HONO K, KAMADO S. Chemistry of nanoscale precipitates in Mg-2.1Gd-0.6Y-0.2Zr (at.%) alloy investigated by the atom probe technique[J]. Mater Sci Eng A, 2005, 395: 301-306.
(Edited by LONG Huai-zhong)
Foundation item: Project(51330) supported by the National Fundamental Research Program of China
Corresponding author: ZHANG Xin-ming; Tel/Fax: +86-731-8830265; E-mail: xmzhang@mail.csu.edu.cn