
Optimization of processing parameters for rheo-casting AZ91D magnesium alloy
WANG Ya-bao(王亚宝), XU Jun(徐 骏), YANG Bi-cheng(杨必成), ZHANG Zhi-feng(张志峰)
General Research Institute for Nonferrous Metals, Beijing 100088, China
Received 12 June 2008; accepted 5 September 2008
Abstract: A design of experiment technique was used to optimize the microstructure of the AZ91D alloy produced by rheo-casting. The experimental design consists of four parameters (pouring temperature, shearing temperature, shearing time and shearing rate) with three levels. The grain size and shape factor measurements of primary α-Mg particles were conducted to determine the microstructure. The contribution of each parameter shows that pouring temperature is the most significant parameter affecting the grain size, and the shape factor highly depends on the shearing temperature. The optimized rheo-casting processing parameters are 650 ℃ for pouring temperature, 585 ℃ for shearing temperature, 40 s for shearing time, and 600 r/min for shearing rate. Under the optimized processing parameters, the average grain size is 28.53 μm, and the shape factor is 0.591.
Key words: AZ91D; rheo-casting; processing parameters; optimization
1 Introduction
With the manufacturing process of the magnesium alloys, the semi-solid forming technologies have been increasingly developed[1]. The rheo-forming process is an innovative one-step semisolid processing technique with many advantages, such as fine and uniform microstructure, enhanced mechanical properties[2]. However, one of the challenges for the application of rheo- forming is to ensure that components hold consistent mechanical and metallurgical properties, implying that semi-solid technology must be controlled within a certain range[3].
During rheo-forming, the preparation of semi-solid slurry with spherical primary grains plays a very important role, because it is the most important factor to effectively achieve the cost and make rheo-forming commercial in industry application. At present, there are many rheo-casting methods for preparing semi-solid slurry, such as the electromagnetic stirring, the new rheo casting (NRCTM) process, the twin-screw process, the semi-solid rheo-casting(SSRTM) process, continuous rheo-convection process(CRP), the sub-liquidus casting(SLC) and the near liquidus casting process. However, only the electromagnetic stirring and the NRCTM processes have successfully been used for exploiting the potential of rheo-forming, and the other processes are still in development now[4-10]. FAN[10] have accounted out that the microstructure of the magnesium alloy was primarily determined by several key processing parameters, such as slurry treatment temperature, treatment time and treatment intensity[11].
In this study, a design of experiment technique was used for better microstructure of the AZ91D. The effects of the parameters were used to determine the optimal levels. The contribution of each factor was determined by the analysis of variance. Proving trails were conducted to verify the analytical results.
2 Experimental
In this study, the AZ91D was melted in an electrical resistance furnace, and then the liquid metal was converted into semi-solid slurry by a twin screw slurry maker. The process was protected by the N2+0.6%SF6 gaseous mixtures[12]. Then the semi-solid slurry was cast in a copper mould to get a d90 mm cylindrical sample. The schematic diagram of the rheo-casting process is shown in Fig.1.
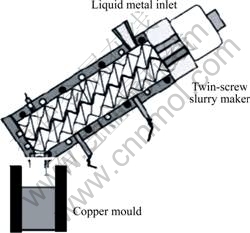
Fig.1 Schematic diagram of rheo-casting process of Mg alloy
Specimens for microstructure observation were cut from the middle section of the samples. The specimens for optical microscopy were prepared by the standard technique of grinding and polishing, followed by etching in an aqueous solution of 60% (volume fraction, the same below) ethylene glycol, 20% acetic acid, 1% concentrated HNO3. The Zeiss optical microscope was utilized for the optical microscopy observations and quantitative measurements. The size and shape factor of primary grains were measured by Micro-image Analysis & Process (MIAPS) software.
The four influential parameters were selected, i.e. pouring temperature, shearing temperature, shearing time and shearing rate, with three levels for each parameter, as listed in Table 1.
Table 1 Parameters and levels selected for experimental analysis
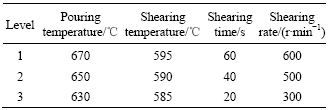
The parameters and levels were used to set up a standard L9(34) orthogonal matrix for the experimental analysis, as listed in Table 2, in which the grain size was measured with the diameter of the globular primary grains, and the shape factor was calculated using F=4πA/P2, where A and P are the total area and the peripheral length of one primary grain, respectively.
3 Results and discussion
The typical microstructure of the rheo-cast AZ91D is shown in Fig.2. It can be seen that the primary grains are tiny and equiaxed. The results of the grain size and the shape factor are listed in Table 2. Ki (i=1, 2, 3) means the average value of level i. To minimize the system error, each trail was repeated twice and two specimens were prepared for statistical evaluation.
3.1 Effect of parameters
Fig.3 shows the effects of the pouring temperature on the grain size and the shape factor. It can be seen that the grain size drops to the bottom of the curve as the
Table 2 Trail conditions and results for experimental analysis
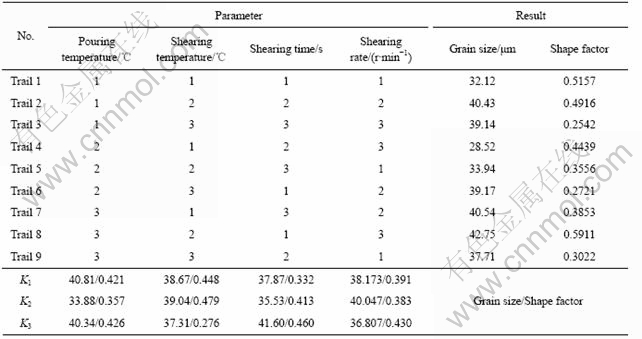
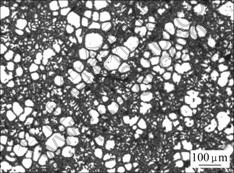
Fig.2 Typical microstructure of AZ91D produced by rheo- casting process for trail 4
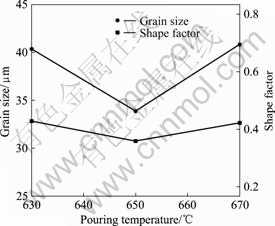
Fig.3 Effect of pouring temperature on grain size and shape factor
pouring temperature increases from 630 ℃ to 650 ℃. When the temperature reaches 670 ℃, the grain size bounces back upward. The shape factor of the grain changes slightly while the pouring temperature increases. This means that the pouring temperature influences the grain size more significantly than the shape factor. As the pouring temperature increases, there are fewer potential nuclei within the bulk liquid and the mechanism of copious nucleation is less pronounced, which eventually leads to the formation of a coarser structure[13-14].
Fig.4 indicates that the grain size and the shape factor reach the peaks with shearing temperature increasing to 590 ℃, and then the grain size decreases mildly with the shearing temperature increasing while the shape factor decreases obviously. The results show that the shearing temperature has a few effects on the grain size but has significant effect on the shape factor. In the intensive shearing process, the effective nucleation rate and the grain growth rate almost keep the same level with the shearing temperature changing, but the grains are not easy to keep round at the higher shearing temperature [15-16].
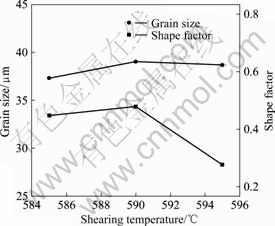
Fig.4 Effect of shearing temperature on grain size and shape factor
The effects of shearing time on grain size and shape factor are shown in Fig.5. It can be seen from Fig.5 that the effect of shearing time is similar to that of the pouring temperature. At first, the grain size decreases as the shearing time increases from 20 s to 40 s, and then increases to the maximum as the shearing time reaches 60 s. The shape factor increases with the shearing time expending. The results are mainly influenced by the continuous nuclear mechanism and the Ostwald ripening. The new grains nucleate and grow up continuously, which leads to the minimum average grain size. Moreover, all the grains will be more and more spherical, owing to the Ostwald ripening[17-18].
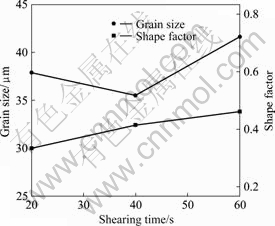
Fig.5 Effect of shearing time on grain size and shape factor
It can be seen from Fig.6 that both the grain size and the shape factor change a little, with the shearing rate increasing from 300 to 500 r/min. However, when the shearing rate increases from 500 to 600 r/min, the grain size drops down with the shape factor increasing, which means the higher shearing speed offers higher power. Higher shearing speeds render smaller and more spherical agglomerates of primary particles.
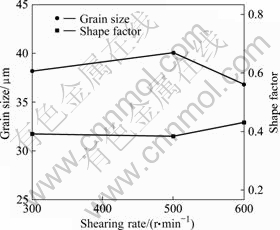
Fig.6 Effect of shearing rate on grain size and shape factor
The contribution of each parameter can be determined by performing analysis of variance based on Table 2. The analysis results of variance are summarized in Table 3. The data show that the contribution of the pouring temperature on the grain size (54.01%) is a half of the contributions of all parameters. Among the contributions of the four parameters, the shearing temperature (65.45%) is more than the contribution
(34.55%) of all the other three parameters. The pouring temperature has the least contribution (2.99%). With a long-range of variation (?t=50 ℃), the pouring temperature has the most important effect on the primary grain size. Although the shearing temperature has little change (?t=10 ℃), it makes the grains more spherical. Therefore, it can be concluded that fine and spherical grain can be obtained if the shearing temperature keeps lower. Shearing time takes the modest contribution to the grain size and the shape factor. The shearing rate has mild contribution to the microstructure of AZ91D. This means that sufficient energy has been supplied by the smallest shearing rate.
3.2 Proving trail
To obtain a fine and uniform microstructure, the pouring and shearing temperatures have to be controlled strictly. As a result, the optimized processing parameters are determined to 650 ℃ for pouring temperature, 585 ℃ for shearing temperature, 40 s for shearing time, and 600 r/min for shearing rate.
Fig.7(a) shows the microstructure of the rheo-cast AZ91D with the optimized parameters. The results are projected to 28.53 μm for the average grain size, and 0.591 for the shape factor.
Table 3 Results of variance for grain size and shape factor
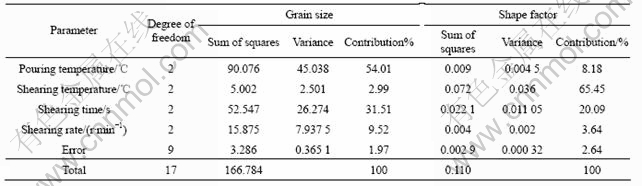
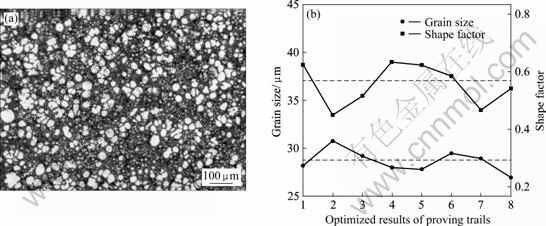
Fig.7 Proving trails results of rheo-cast AZ91D with optimized processing parameters: (a) Typical optimized microstructure; (b) Grain size and shape factor of proving experiments
It can be seen that the primary grains become smaller and more spherical, which is in good agreement with the projected parameters. It can also be found in Fig.7(b) that the optimized results are reproducible. With the optimized processing parameters, it is pointed out that the fine and spherical grains can be obtained and the process appears stable.
4 Conclusions
1) The pouring and shearing temperatures have the most significant contribution to the development of a fine and uniform microstructure. The shearing time and the shearing rate have few effects on the microstructure of the rheo-cast magnesium alloy AZ91D.
2) The optimized rheo-casting processing parameters are determined to 650 ℃ for pouring temperature, 585 ℃ for shearing temperature, 40 s for shearing time and 600 r/min for shearing rate.
3) The optimized outcome is 28.53 μm for the average grain size, and 0.591 for the shape factor. The proving trail results verify that the experimental analysis is reliable.
References
[1] BROWN S B, FLEMINGS M C. Net-shape forming via semi-solid processing [J]. Advanced Materials & Process, 1993, 1(93): 36-40.
[2] JOLY P A, MEHRABIAN R. The rheology of a partially solid alloy [J]. J Mater Sci, 1976, 11: 1393-1418.
[3] CHOU H N, GOVENDER G, IVANCHEV L. Opportunities and challenges for use of SSM forming in the aerospace industry [C]// Proceedings of the 9th International Conference on Semi-Solid Processing of Alloys and Composites. Busan, Korea, 2006: 92-95.
[4] KIRKWOOD D H. Semisolid metal processing [J]. International Materials Reviews, 1994, 39(5): 174-189.
[5] FLEMINGS M C. Behavior of metal alloys in the semisolid state [J]. Metallurgical Transactions, 1991, A 22(4): 957-981.
[6] DOHERTY R D, LEE H I, FEEST E A. Microstructure of stir-cast metals [J]. Material Science and Engineering A, 1984, 65: 181-189.
[7] KAUHANN H, WABUSSEG H, UGGOWITZER P J. Metallurgical and processing aspects of the NRC semi-solid casting technology [J]. Aluminum, 2000, 76(1/2): 70-75.
[8] MARTINEZ R A, FLEMINGS M C. Evolution of particle morphology in semisolid processing [J]. Metall Mater Trans, 2005, A36: 2205-2210.
[9] APELIAN D, PAN Q Y, FINDON M. Low cost and energy efficient methods for the manufacture of semi-solid(SSM) feedstock [J]. Die Casting Engineer, 2004, 48(1): 22-28.
[10] FAN Z. Semisolid metal processing [J]. International Materials Reviews, 2002, 47(2): 1-34.
[11] ZHANG Shao-ming, YANG Bi-cheng, FAN Zhong-yun, XU Jun, SHI Li-kai, CHEN Guo-liang. Rheo-extrusion of AZ61 Mg alloy and its microstructure [J]. The Chinese Journal of Nonferrous Metals, 2007, 17(9): 1423-1428. (in Chinese)
[12] JI S, FAN Z, BEVIS M J. Semi-solid processing of engineering alloys by a twin-screw rheomoulding process [J]. Materials Science and Engineering A, 2001, 299(1/2): 210-217.
[13] NING Zhi-liang, WANG H, SUN Jian-fei. The effect of initial microstructure of A356 alloys on the mechanical behavior in the semisolid state[C]// Proceedings of the 9th International Conference on Semi-Solid Processing of Alloys and Composites. Busan, Korea, 2006: 449-452.
[14] LASHKARI O, NAFISI S, GHOMASHCHI R. Microstructural characterization of rheo-cast billets prepared by variant pouring temperatures [J]. Materials Science and Engineering A, 2006, 441(1/2): 49-59.
[15] YANG Ming-bo, PAN Fu-sheng, CHENG Ren-ju, BAI Liang. Effect of semi-solid isothermal heat treatment on the microstructure of Mg-6A1-1Zn-0.7Si alloy [J]. Journal of Materials Processing Technology,2008, 206(1/3): 374-381.
[16] ZHAO Hong-jin, KANG Yong-lin, WANG Zhao-hui, DU Hai-liang. Effect of disturbing conditions in semi-solid state on microstructure evolution of magnesium alloy [C]// Proceedings of the 9th International Conference on Semi-Solid Processing of Alloys and Composites. Busan, Korea, 2006: 296-299.
[17] CHEN H I, CHEN J C, LIAO J J. The influence of shearing conditions on the rheology of semi-solid magnesium alloy [J]. Materials Science and Engineering A, 2008, 487(1/2): 114-119.
[18] FAN Z, LIU G, HITCHCOCK M. Solidification behaviour under intensive forced convection [J]. Materials Science and Engineering A, 2005, 413/414: 229-235.
(Edited by LI Xiang-qun)
Foundation item: Project (2006CB605203) supported by the National Basic Research Program of China; Project (2006AA03Z115) supported by the Hi-tech Research and Development Program of China
Corresponding author: WANG Ya-bao; Tel: +86-10-82241229; E-mail: wangyabao@hotmail.com