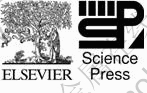
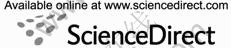
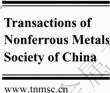
Trans. Nonferrous Met. Soc. China 22(2012) 1961-1966
Effect of joining temperature on microstructure and properties of diffusion bonded Mg/Al joints
SHANG Jing, WANG Ke-hong, ZHOU Qi, ZHANG De-ku, HUANG Jun, GE Jia-qi
School of Materials Science and Engineering, Nanjing University of Science and Technology, Nanjing 210094, China
Received 10 November 2011; accepted 27 April 2012
Abstract: The joining of AZ31B Mg alloy to 6061 Al alloy was investigated at different joining temperatures by vacuum diffusion bonding method. The microstructures of Mg/Al dissimilar joints were studied by means of optical microscopy (OM), scanning electron microscopy (SEM) and energy dispersive X-ray (EDX). The results show that the thickness of each layer in the diffusion zone increases with the increase of joining temperature, and the microstructure changes obviously. At joining temperature of 440 ℃, the diffusion zone is composed of Mg2Al3 layer and Mg17Al12 layer. At joining temperatures of 460 and 480 ℃, the diffusion zone is composed of Mg2Al3 layer, Mg17Al12 layer, eutectic layer of Mg17Al12 and Mg-based solid solution. The width of high-hardness zone in the joint increases with increasing joining temperature, and the micro-hardnesses at different locations in the diffusion zone are significantly different. The joining temperature of 440 ℃ offers the highest tensile strength of 37 MPa, and the corresponding joint exhibits brittle fracture at the intermetallic compound layer of Mg17Al12.
Key words: vacuum diffusion bonding; 6061 aluminum alloy; AZ31 magnesium alloy; dissimilar metal joint; microstructure; mechanical property
1 Introduction
As is well known that magnesium alloys and aluminum alloys are widely used in aviation, aerospace, automotive, machinery, electrical and chemical industry. Wide and cross application of these two metals makes the connection of Mg/Al dissimilar metals inevitable [1,2]. For example, the Mg-Al laminated armor is used in tanks and armored vehicles [3], Mg-Al complex structure is used in aerospace engines and components [4], and Mg-Al connected pipe is used in bicycle manufacture [5]. So achieving reliable connection of Mg/Al dissimilar metals can exert different performance, which will be widely used in military, aerospace, automotive and other fields [6,7].
At present, it is difficult to get joint of Mg/Al dissimilar metals with good performance. Intermetallic compounds which seriously impact the performance of the joint are inevitably generated in the joint during the welding process [8-11]. So an effective method that can control the generation of intermetallic compounds is urgently needed to achieve the reliable connection of Mg-Al dissimilar joint. Vacuum diffusion bonding can precisely regulate the heating temperature and holding time, and then control the generation of intermetallic compounds [12,13]. The dissimilar materials of Ti/Al and Fe3Al/Q235 have been bonded successfully by means of vacuum diffusion bonding [14,15]. In this work, vacuum diffusion bonding was used to study the internal relations between welding temperature and joints performance. The study provides a theoretical reference and practical experience for Mg/Al dissimilar metal connection.
2 Experimental
Samples of AZ31B Mg alloy and 6061 Al alloy were welded in this experiment. The chemical compositions and part of the physical and mechanical properties of these two metals are listed in Tables 1 and 2, respectively.
Before welding, the oxide films on substrates surface were removed by stainless steel wire brush, and acetone was wiped to remove the oil. Base metals were welded in a vacuum ZTY-40-20 type diffusion bonding machine. The main welding parameters are listed in Table 3.
Table 1 Chemical compositions of AZ31B and 6061alloys (mass fraction, %)

Table 2 Physical and mechanical properties of AZ31B and 6061 alloys

Table 3 Welding parameters of AZ31B and 6061 alloys
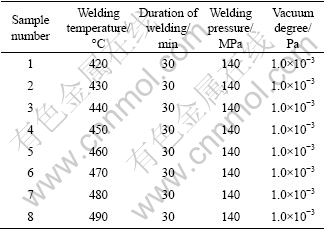
A series of specimens were cut along the longitudinal direction of the joints by a lining cutting machine and mounted in bakelite for microstructure examination. The Mg alloy side of the joint was etched in a solution of 1 mL oxalic acid+1 mL nitric acid + 1 mL acetic acid + 150 mL distilled water. The Al alloy side of the joint was etched in a solution of 2 mL hydrofluoric acid + 5 mL nitric acid + 95 mL distilled water. The microstructure, fracture morphology and phase composition of the joints were observed and analyzed respectively by an Olympus (GX41) optical microscope, S-3400N II scanning electron microscope and EX-250 energy dispersive spectroscope. The micro-hardness distribution was measured by the 402MVD digital display micro-hardness tester under a load of 0.98 N for 10 s. A SANS CMT5105 universal testing machine was used for tensile test at a loading rate of 1 mm/min. The dimensions of the tensile test samples are shown in Fig. 1.
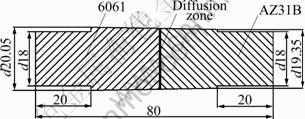
Fig. 1 Dimensions of tensile test samples (Unit: mm)
3 Results and discussion
3.1 Microstructures of joints
By Arrhenius formula, welding temperature is the most important parameter in diffusion bonding process, therefore, the microstructures of the joints welded at different temperature were studied. The microstructure of the joint welded at 440 ℃ is shown in Fig. 2(a). It can be seen that the joint is combined well without defects such as inclusion, porosity and incomplete fusion. Two layers can be clearly observed in the joint, the thickness of layer A near Al side is about 15 μm, the thickness of layer B near Mg side is about 5 μm. The microstructure of the joint welded at 460 ℃ is shown in Fig. 2(b). With the rise of welding temperature, the thicknesses of layers A and B increase significantly. Layer B is grown irregularly towards Mg side, and eutectic layer C is formed due to the continuous diffusion of the atoms. The microstructure of the joint welded at 480 ℃ is shown in Fig. 2(c). The thickness of layer A continuously increases, and layer B is changed into irregular columnar grain structure which is grown into layer C. Meanwhile, the thickness of layer C which is composed of relatively uniform eutectic structure increases sharply because atoms are diffused evenly and fully at higher welding temperature.
The SEM micrograph of the joint in sample 5 is shown in Fig. 3. Some micro-voids can be seen between layers B and C due to Kirkendall effect [16]. The reason is that the diffusion rates of Mg and Al atom are different in the welding process, the diffusion of Al atom to Mg substrate is faster than that of Mg atom to Al substrate, so the quantities of atoms transferred and diffused between layers B and C are unequal in both sides of the interface, then the micro-voids are formed with the loss of atoms at the interface. The EDX analysis results obtained from different locations shown in Fig. 3 are illustrated in Figs. 4(a)-(c). Combined with Al-Mg phase diagram and the analysis of Ref. [17], the results are obtained as follows. Figure 4(a) shows the EDX result from point 1 in Fig. 3, it consists of 59.60% Al and 40.40% Mg (molar fraction), which suggests that layer A is composed of Mg2Al3. Figure 4(b) shows the EDX result from point 2 in Fig. 3, where Mg element increases to 58.55%, while Al decreases to 41.45%, indicating that layer B is composed of Mg17Al12. Figure 4(c) shows the EDX result from point 3 in Fig. 3, it consists of 8.36% Al and 91.64% Mg, suggesting that this location mainly consists of Mg-based solid solution. So it can be confirmed that the eutectic structure in layer C is composed of Mg17Al12 and Mg-based solid solution.
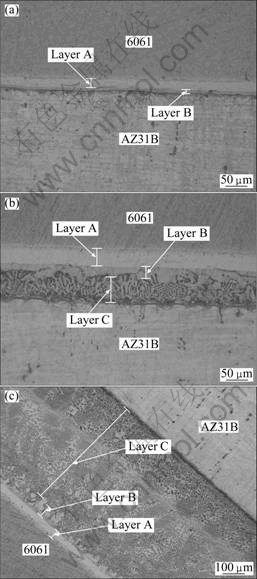
Fig. 2 Microphotographs of joints at different welding temperatures: (a) 440 ℃; (b) 460 ℃; (c) 480 ℃
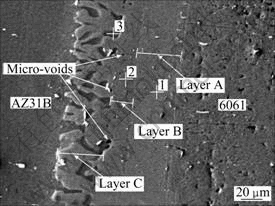
Fig. 3 SEM micrograph of joint in sample 5
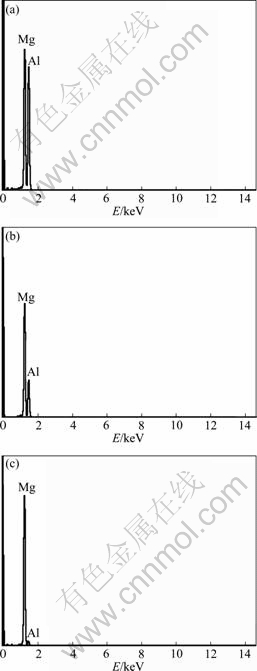
Fig. 4 EDX analysis results from different locations shown in Fig. 3: (a) Point 1; (b) Point 2; (c) Point 3
3.2 Mechanical properties of joints
3.2.1 Micro-hardness analysis
The micro-hardness test points were taken with the step of 0.15 mm on the joints. The distributions of micro-hardness of samples 3, 5 and 7 are respectively shown in Figs. 5 (a)-(c). Two dotted lines represent the fusion lines of Al side and Mg side, respectively. The general distribution trends of the micro-hardness of the joints welded at different welding temperature are the same. The micro-hardness suddenly increases in the diffusion zone, and the micro-hardnesses in both sides of the substrates are distributed uniformly, about HV 60 in Mg side and HV 42 in Al side. As the welding temperature increases, the width of the high- hardness zone in the joint increases. In addition, the micro- hardnesses in different locations of the diffusion zone are significantly different, as shown in Fig. 5(c). In diffusion zone, the micro-hardness close to the Al substrate is about HV 240.1 (layer A), which is higher than HV 184.9 (Layer C) close to the Mg substrate. Different types of intermetallic compounds in different areas of diffusion zone result in different micro-hardness.
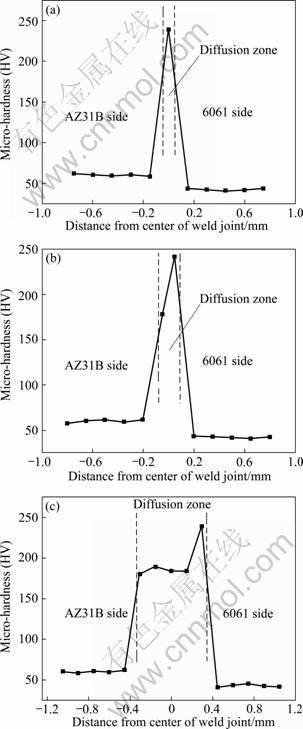
Fig. 5 Micro-hardness distribution of joints at different welding temperature: (a) 440 ℃; (b) 460 ℃; (c) 480 ℃
3.2.2 Tensile strength and fracture analysis
The tensile strengths of the joints at different welding temperature are shown in Fig. 6. As the welding temperature increases, the tensile strength shows an increasing trend at first and then decreases. When the welding temperature is 440 ℃, tensile strength reaches the highest value of 37 MPa. The reason is that when the welding temperature is low, the connection between atoms is not realized. With increasing welding temperature, high energy and sufficient diffusion can be obtained, appropriate thickness of the intermetallic compounds and good atom connection are formed. As the welding temperature continues to increase, the diffusion rate of atoms and the thickness of intermetallic compounds increase sharply, meanwhile, numerous welding defects such as diffusion holes and microcracks are generated in the diffusion zone. Thick intermetallic compound layers and numerous welding defects generated in the joint result in the decrease of joint strength.
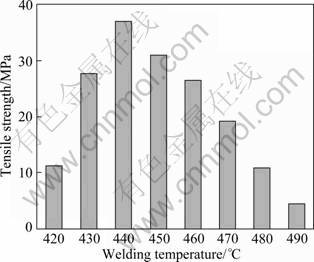
Fig. 6 Tensile strengths of joints at different welding temperature
Figure 7 shows the SEM photograph of the fracture in sample 3. Some cleavage steps and a large number of fan-shaped patterns are clearly seen. It can be confirmed that the fracture is in brittle fracture mode.
Figure 8 shows the EDX result from area A (Fig. 7), consisting of 58.19% Mg and 41.81% Al, which suggests that this location is mainly composed of Mg17Al12. It can be confirmed that the fracture occurs in layer B (Fig. 2(a)). The intermetallic compound of Mg17Al12 with brittle properties distributes continuously in the joint, leading to the fracture.
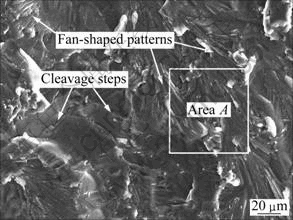
Fig. 7 SEM photograph of fracture in sample 3
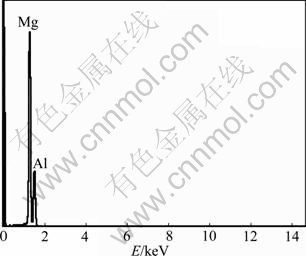
Fig. 8 EDX analysis result from area A shown in Fig. 7
4 Conclusions
1) AZ31B Mg alloy and 6061 Al alloy are successfully joined by vacuum diffusion bonding. With the increase of welding temperature, the thickness of each layer in diffusion zone increases. At the welding temperature of 440 ℃, the diffusion zone is composed of Mg2Al3 layer and Mg17Al12 layer. At welding temperature of 460 and 480 ℃, the diffusion zone is composed of Mg2Al3 layer, Mg17Al12 layer, eutectic layer of Mg17Al12 and Mg-based solid solution.
2) With the rise of welding temperature, the width of high-hardness zone in the joint increases and the micro-hardnesses in different locations of diffusion zone are significantly different, HV 240 close to the Al substrate and HV185 close to the Mg substrate.
3) As the welding temperature increases, the tensile strength shows an increasing trend at first and then decreases. The highest value of tensile strength is 37 MPa at welding temperature of 440 ℃. A large amount of Mg17Al12 intermetallic compound distributed continuously in the joint results in the brittle fracture.
References
[1] FENG Ji-cai, WANG Ya-rong, ZHANG Zong-dian. Status and expectation of research on welding of magnesium alloy [J]. The Chinese Journal of Nonferrous Metals, 2005, 15(2): 165-178. (in Chinese)
[2] CAO X, JAHAZI M, IMMARIGEON J P, WALLACE W. A review of laser welding techniques for magnesium alloys [J]. J Mater Process Technol, 2006, 171(2): 188-204.
[3] LI Hui, QIAN Ming, LI Da. The effect of intermetallic compounds on laser weldability of dissimilar metal joint between magnesium alloy AZ31B and aluminum alloy 6061 [J]. Laser Journal, 2007, 28(5): 61-63. (in Chinese)
[4] WANG J, FENG J C, WANG Y X. Microstructure of Al–Mg dissimilar weld made by cold metal transfer MIG welding [J]. Mater Sci Technol, 2008, 24(7): 827-831.
[5] KWON Y J, SHIGEMATSU I, SAITO N. Dissimilar friction stir welding between magnesium and aluminum alloys [J]. Mater Lett, 2008, 62: 3827-3829.
[6] ZENG Rong-chang, KE Wei, XU Yong-bo, HAN En-hou, ZHU Zi-yong. Recent development and application of magnesium alloys [J]. Acta Metallurgica Sinica, 2001, 37(7): 673-685. (in Chinese)
[7] LARSSON J K. Overview of joining technologies in the automotive industry [J]. Welding Res Abroad, 2003, 49(6): 29-45.
[8] LIU L M, WANG H Y, ZHANG Z D. The analysis of laser weld bonding of Al alloy to Mg alloy [J]. Scripta Mater, 2007, 56: 473-478.
[9] SHANG Jing, WANG Ke-hong, ZHOU Qi, ZHANG De-ku, HUANG Jun, LI Guang-le. Microstructure characteristics and mechanical properties of cold metal transfer welding Mg/Al dissimilar metals [J]. Mater Des, 2012, 34: 559-565.
[10] LIU Li-ming, REN Da-xin. A novel weld-bonding hybrid process for joining Mg alloy and Al alloy [J]. Mater Des, 2011, 32: 3730-3735.
[11] BORRISUTTHEKUL R, MIYASHITA Y, MUTOH Y. Dissimilar material laser welding between magnesium alloy AZ31B and aluminum alloy A5052-O [J]. Sci Technol Adv Mater, 2005, 6: 199-204.
[12] LIU Peng, LI Ya-jiang, WANG Juan, GUO Ji-shi. Vacuum brazing technology and microstructure near the interface of Al/18-8 stainless steel [J]. Mater Res Bull, 2003, 38(9-10): 1493-1499.
[13] MAHENDRAN G, BALASUBRAMANIAN V, SENTHILVELAN T. Developing diffusion bonding windows for joining AZ31B magnesium–AA2024 aluminium alloys [J]. Mater Des, 2009, 30: 1240-1244.
[14] REN Jiang-wei, LI Ya-jiang, FENG Tao. Microstructure characteristics in the interface zone of Ti/Al diffusion bonding [J]. Mater Lett, 2002, 56(5): 647-652.
[15] LI Ya-jiang, WANG Juan, YIN Yan-sheng, WU Hui-qiang, FENG Ji-cai. Analysis of microstructure in the interface of diffusion welding for Fe3Al/Q235 dissimilar material [J]. Transactions of the China Welding Institution, 2002, 23(2): 25-28. (in Chinese)
[16] OSMAN Y, MUSTAFA A. Investigation of micro-crack occurrence conditions in diffusion bonded Cu-304 stainless steel couple [J]. J Mater Process Technol, 2002, 121: 136-142.
[17] LI Xian-rong, LIANG Wei, ZHAO Xing-guo, ZHANG Yan, FU Xiao-peng, LIU Fen-cheng. Bonding of Mg and Al with Mg–Al eutectic alloy and its application in aluminum coating on magnesium [J]. J Alloys Compd, 2009, 471: 408-411.
焊接温度对Mg/Al扩散焊接头微观组织和性能的影响
尚 晶,王克鸿,周 琦,张德库,黄 俊,葛佳棋
南京理工大学 材料科学与工程学院,南京 210094
摘 要:采用真空扩散焊在不同焊接温度下对AZ31B镁合金和6061铝合金进行连接。利用光学显微镜(OM)、扫描电镜(SEM)和能谱(EDX)观察Mg/Al异种金属接头的显微组织。结果表明:随着焊接温度的升高,扩散区各层的厚度增加,且组织发生明显变化。440 ℃时扩散层由Mg2Al3层和Mg17Al12层组成;460和480 ℃时由Mg2Al3层、Mg17Al12层和Mg17Al12与镁基固溶体的共晶层组成。随着加热温度的升高,高硬度区域显著增多,区域内不同位置的硬度存在明显差别。当焊接温度为440 ℃时接头的最大抗拉强度为37 MPa,脆性断裂发生在Mg17Al12层。
关键词:真空扩散焊;6061铝合金;AZ31镁合金;异种金属焊接头;微观组织;力学性能
(Edited by FANG Jing-hua)
Foundation item: Project (51075214) supported by the National Natural Science Foundation of China
Corresponding author: WANG Ke-hong; Tel: +86-25-84315776; E-mail: khwang1602@163.com; wkh1602@mail.njust.edu.cn
DOI: 10.1016/S1003-6326(11)61414-3