J. Cent. South Univ. Technol. (2008) 15: 373-377
DOI: 10.1007/s11771-008-0070-3

FTIR analysis of adsorption of poly diallyl-dimethyl-ammonium chloride on kaolinite
SUN Wei(孙 伟), LIU Wen-li(刘文莉), HU Yue-hua(胡岳华)
(School of Resource Processing and Bioengineering, Central South University, Changsha 410083, China)
Abstract: The flocculation behavior of ultrafine kaolinite suspension was investigated through settlement tests and FTIR method was employed to probe the adsorption mechanism of flocculant on kaolinite. The results show that the maximum settling rate of kaolinite occurs at pH value of 3.33, which is close to the point of zero charge(PZC) of kaolinite (3.5). This result is in good agreement with the double electric layer theory. Kaolinite suspension reaches the largest settling rate at a low concentration of 39 g/t for poly diallyl-dimethyl-ammonium chloride(PDADMA) flocculant, whereas for polyacrylamides(PAM) the dosage is required to be 500 g/t. When macromolecule polymer is adsorbed on surface, kaolinite particles may be flocculant due to the bridging effect. There are cation flocculant characteristic bands on the spectrum of kaolinite but no obvious shifting. Thus, the adsorption of poly diallyl-dimethyl-ammonium chloride on kaolinite surface is physical adsorption.
Key words: adsorption; FTIR spectrum; kaolinite; poly diallyl-dimethyl-ammonium chloride; electrostatic; flocculant
1 Introduction
Diasporic-bauxite in China is characterized by high content of aluminum oxide and silica but low mass ratio of Al2O3 to SiO2 (average 5-6). Bayer’s process cannot economically produce aluminum oxide from diasporic-bauxite because the high content of SiO2 causes high NaOH and energy consumption[1-4]. Therefore, it is necessary to effectively remove the aluminosiliate minerals such as kaolinite, pyrophllite and illite from the bauxite to increase the mass ratio of Al2O3 to SiO2[5-7].
Flotation provides a promising way to remove silicates. Direct flotation has been proved to be an efficient method to de-silicate diasporic-bauxite using oleic acid as collector in the slurry with pH value of 9-10[8-12]. However, clay tailings arising from diaspore direct flotation incur great problems in dewatering process such as the poor settling rates, the long production flow, the collapse of thicker and the difficulty of utilizing recycling water. It is understood that coagulation and flocculation are in favor of tailing rapid settlement and water reusing[13]. Our goal in this research is to find a flocculant to promote the settling rate of clay tailing. Many researches on the settlement of clay suspensions have been carried out. Currently, a mass of work has been done to use polyacrylamides(PAM) as flocculants in kaolinite settling systems due to its effec-tiveness and ability to produce good settling performance. Several studies have been conducted to investigate the accelerating effect of polyacrylamide on kaolinite settlement. LEE et al[14] have concluded that the adsorption of polyacrylamide occurs primarily onto the edge surface of kaolinite (i.e. on the broken bonds of aluminol (Al—OH) and silanol (Si—OH) groups) via hydrogen bonding and 94% of adsorption takes place on the edge surface. NASSER and JAMES[15] has reported that employing high molecular mass anionic PAM as flocculation reagent is in favor of the settling of negatively charged kaolinite particle, in which the polymer bridging mechanism is of primary importance, whereas charge neutralisation will be of secondary or little importance. In this work, a new kind of flocculant for kaolinite settling, poly diallyl-dimethyl-ammonium chloride (PDADMA or Cat-Floc), which is harmless, more efficient and environmental friendly, was used in bauxite ore processing. Its adsorption mechanism was discussed based on the results of FTIR spectrum.
2 Experimental method
2.1 Material and reagent
Lump of kaolinite was from Shanxi Deposit, China. The mineral lumps were crushed and ground in a laboratory porcelain mill. Particles with an average diameter of 3.85 μm were collected and used in settle-ment tests. The chemical composition of the material was analyzed. The results are listed in Table 1.
Table 1 Analytical results of chemical composition for kaolinite (mass fraction, %)
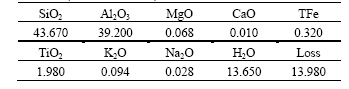
As pH regulator, analytical grade hydrochloric acid and NaCO3 were diluted to 1%. PAM and PDADMA were industrial grade, which were provided by Zhuzhou Flotation Reagent Corporation, their relative molecular masses were around 8×106. Distilled water was used in all tests.
2.2 Settlement tests
In settlement experiments, the kaolinite suspension was prepared by putting 3 g mineral samples into a beaker with 40 mL distilled water. The resultant suspension was dispersed by an electronic stirrer at a speed of 1 300 r/min for 8 min. After adding flocculant or pH regulator the suspension was agitated for 3 min. Then the slurry was gently poured into a 100 mL measuring cylinder and inverted 10 times. The height of ‘mud-line’ (solid-liquid interface) was recorded at certain intervals.
3 Results and discussion
3.1 Effect of pH value on settling of kaolinite suspension
Tests were undertaken as described above, initially at pH values of 1.93, 2.06, 2.88, 3.33, 3.62 and 5.09 using hydrochloric acid as pH regulator. The results are presented in Fig.1. It can be seen that with the increase of settling time, the height of solid-liquid interface decreases and kaolinite particles settle. The more obviously the height of solid-liquid interface decreases at certain intervals, the greater the settling rate of kaolinite is. The maximum settling rate of kaolinite is observed at pH=3.33, when pH<3.33 or pH>3.33, the settling rate of the kaolinite decreases.
These results can be explained by the charged mechanism of kaolinite. The electrical property of a mineral surface is greatly related to pH value, which is described as

MOH(s)+H+(aq) (1)
MOH(s)
MO-(s)+H+ (2)
where M is cation of kaolinite, Al or Si, MOH(s) is non-charged region,
is positive charged
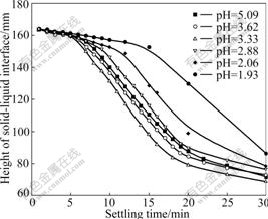
Fig.1 Settling rate of kaolinite suspension as function of pH value
region and MO-(s) is negative charged region. Our previous reports showed that the kaolinite was negatively charged and the PZC was around pH=3.5[16]. At pH<pHPZC the particle surface is positively charged, and at pH>pHPZC the particle surface is negatively charged. Only when pH is around pHPZC, the mineral particle surface repulsion force becomes weaker, the suspension gets unstable, and particles begin to agglomerate, resulting in high settling rate.
3.2 Effect of flocculant on flocculation settling of kaolinite
Fig.2 shows the flocculation rate of the kaolinite suspension as a function of dosage of flocculant, i.e. PDADMA. It can be seen that with the increase of the dosage of flocculants, the settling rate of the kaolinite suspension increases. The settling rate of kaolinite suspension is largest at 39 g/t, after which it descends. And also with the increase of settling time, the settling rate increases in the presence of flocculant. Compared with that without flocculant in Fig.1, the addition of PDADMA greatly promotes the settling of kaolinite
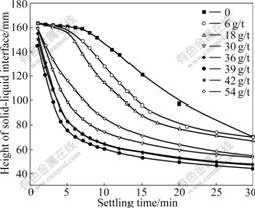
Fig.2 Settling rate of kaolinite suspension as function of dosage of PDADMA (pH=6.86, concentration of PDADMA is 0.006%)
particles. The height of “mud-line” is below 80 mm in a short time about 5 min in the presence of flocculant compared with that in 30 min in the absence of flocculant.
Fig.3 shows the settling rate of the kaolinite suspension as a function of dosage of flocculant, i.e. PAM. With the increase of the dosage of flocculants, the settling rate of the kaolinite suspension increases, but the dosage (100-500 g/t) is far more than that of PDADMA (<40 g/t).
Fig.4 compares flocculation efficiencies of the kaolinite suspension by using PDADMA (39 g/t) and PAM (500 g/t), respectively, which indicates that flocculation efficiency of kaolinite suspension using PDADMA as flocculant is greater than that using PAM as flocculant.
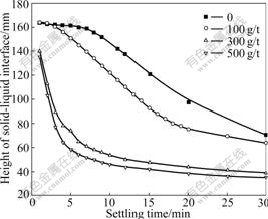
Fig.3 Settling rate of kaolinite suspension as function of dosage of PAM (pH=6.86, concentration of PAM is 0.05%)
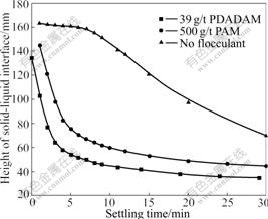
Fig.4 Comparison of flocculation ability of PDADMA and PAM (pH=6.86, concentration of PDADMA is 0.006%, concentration of PAM is 0.05%)
4 FTIR analysis on surface interaction of PDADMA with kaolinite
The FTIR spectra of kaolinite and flocculant were measured. The results are given in Figs.5-7.
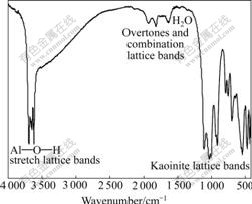
Fig.5 FTIR spectrum of kaolinite
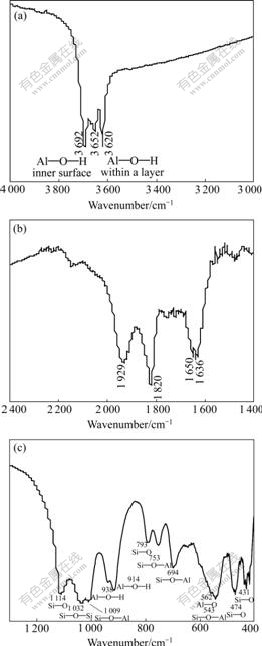
Fig.6 Enlarged FTIR spectra of kaolinite: (a) Al—O—H stretch lattice bands; (b) Overtones and combination lattice bands; (c) Kaolinite lattice bands
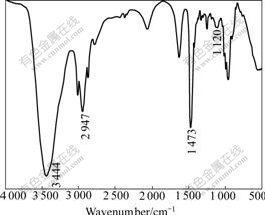
Fig.7 FTIR spectrum of PDADMA
Figs.5 and 6 show the FTIR spectra and enlarged spectra of kaolinite, respectively. The spectrum of kaolinite is composed of three parts: Al— O—H stretch bands are at 3 600-3 700 cm-1, overtones and combination lattice bands are at 1 200-400 cm-1, kaolinite lattice bands are at 400-1 300 cm-1.
In Al—O—H stretch lattice bands, there are O—H stretches of the inner surface Al—O—H groups between the kaolinite layers at 3 692, 3 669 and 3 652 cm-1, O—H stretch of the inner Al—O—H between the siloxane and alumina sheet within layers at 3 620 cm-1. Furthermore, Al—O—Al deformation stretches of O—H appear at 938 and 914 cm-1.
In kaolinite lattice bands, there are Si—O stretches at 1 114, 1 100, 1 032, 1 009, 793, 753, 694 cm-1 and Si—O deformation at 543, 474 and 431 cm-1.
Al—O stretch is mostly in low wave number regions, only a weak band is seen at 562 cm-1.
Table 2 shows the assignment of bands observed in the FTIR spectra of kaolinite.
Fig.7 shows the FTIR spectrum of PDADMA. N—C symmetrical stretch is at 1 120 cm-1. C—N stretch of amine is generally at 1 230-1 030 cm-1 because of the induction effect of C joined with N, and this band is in the region of low wavenumber. The assignment of bands observed in the FTIR spectrum of PDADMA is presented in Table 3.
Fig.8 shows the FTIR spectra of kaolinite adsorbed with flocculant. Some characteristic bands of PDADMA appear on the spectrum of kaolinite with flocculant: C—H unsymmetrical stretch of —CH3 is at 2 947 cm-1; O—H unsymmetrical deformation stretch is at 1 465 cm-1; C—H deformation stretch is at 1 339 cm-1. Because the concentration is too low, the three bands are very weak and have no marked shifting. This indicates that the adsorption of PDADMA on kaolinite is physical adsorption. Because PDADMA is a cationic polymer, it is adsorbed on negatively charged kaolinite surface and
makes the kaolinite particles flocculated through
Table 2 Assignment of bands observed in FTIR spectrum of kaolinite
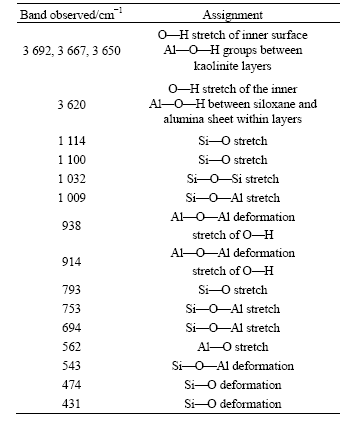
Table 3 Assignment of bands observed in FTIR spectrum of PDADMA
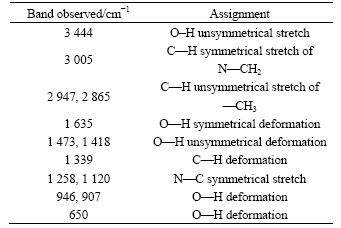
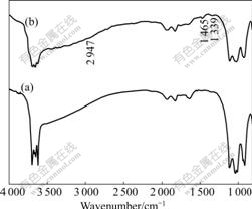
Fig.8 Spectra of kaolinite with flocculant adsorbed: (a) Kaolinite; (b) Kaolinite with flocculant adsorbed
bridging action. The physical adsorption of PDADMA on kaolinite is mainly due to the electrostatic interaction.
RYTWO et al[17] measured Si—OH stretch bands of non-charged point of clay (at around 3 700 cm-1) and O—H bending bands (at around 800 cm-1) to explain the adsorption of organic molecule on clay. Fig.9 shows the O—H deformation band at about 800 cm-1. When PDADMA is adsorbed on non-charged point of ore, the two O—H bands will become wider.
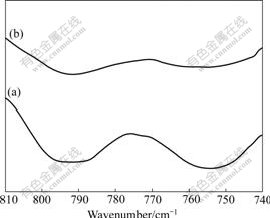
Fig.9 O—H deformation bands at 740-810 cm-1: (a) Kaolinite; (b) Kaolinite with flocculant adsorbed
5 Conclusions
1) The point of zero charge of kaolinite is 3.5. The settling rate of kaolinite suspension is the largest at pH=3.33.
2) With the increase of the dosage of flocculants, the settling rate of the kaolinite suspension increases, and after getting a peak it descends. The settling rate of kaolininite suspension is the largest at PDADMA dosage of 39 g/t.
3) The FTIR analysis indicates that the adsorption of PDADMA on kaolinite is physical adsorption mainly due to the flocculants interaction. The focculant molecules are adsorbed on negatively charged point of kaolinite.
References
[1] LUO Zhao-jun, WANG Yu-hua, HU Yue-hua, QIU Guan-zhou. Optimization of grinding in reverse flotation for bauxite [J]. Transactions of Nonferrous Metals Society of China, 2001, 11(3): 444-446.
[2] ZHANG Jian-feng, HU Yue-hua, WANG Dian-zuo. Preparation and determination of hydroximic polyacrylamide [J]. Journal of Central South University of Technology, 2002, 9(3): 177-180.
[3] OUYANG Jian, LU Shou-ci. Character and processing research of Chinese bauxite resource [J]. Multipurpose Utilization of Mineral Resource, 1995(2): 24-27. (in Chinese)
[4] LIU Zhong-fan. Outlook of Chinese bauxite resource [J]. Light Metals, 2001(2): 4-9. (in Chinese)
[5] HU Yue-hua, QIU Guan-zhou, MILLER J D. Aggregation/dispersion of ultrafine silica in flotagent solution [J]. Transactions of Nonferrous Metals Society of China, 2001, 11(5): 768-773.
[6] WANG Yu-hua, HU Yue-hua, LIU Xiao-wen. Flotation de-silicating from diasporic-bauxite with cetyl trimethylammonium bromide [J]. Journal of Central South University of Technology, 2003, 10(4): 324-328.
[7] ZHANG Guo-fan, FENG Qi-ming, LU Yi-ping, LIU Guang-yi, OU Le-ming. Effect of sodium hexametaphosphate on flotation of bauxite [J]. Journal of Central South University of Technology: Natural Science, 2001, 32(2): 127-130. (in Chinese)
[8] BOJANA D, LUDVIK T. The impact of structure on the undrained shear strength of cohesive soils [J]. Engineering Geology, 2007, 92(1/2): 88-96.
[9] DAI X N, HOU W G, DUAN H D, NI P. Thixotropy of Mg-Al-layered double hydroxides/kaolinite dispersion [J]. Colloids and Surfaces A: Physicochemical and Engineering Aspects, 2007, 295(1/3): 139-145.
[10] ZHANG Guo-fan. Theory and technology of flotation on bauxite desilicate [D]. Changsha: Central South University, 2001: 7-8. (in Chinese)
[11] LU Yi-ping, ZHANG Guo-fan, FENG Qi-ming, OU Le-ming. A noval collector RL for flotation of bauxite [J]. Journal of Central South University of Technology, 2002, 9(1): 21-24.
[12] CHEN Xiang-qing, HU Yue-hua, WANG Yu-hua. Effects of sodium hexmeta phosphate on flotation separation of diaspore and kaolinite [J]. Journal of Central South University of Technology, 2005, 12(4): 421-423.
[13] MCFARLANE A, BREMMELL K, ADDAI-MENSAH J. Improved dewatering behavior of clay minerals dispersions via interfacial chemistry and particle interactions optimization [J]. Journal of Colloid and Interface Science, 2006, 293(1): 116-127.
[14] LEE Y S, PARK I H, YOO J S, CHUNG S Y, LEE Y C, CHO Y S, AHN S C, KIM C M, CHOI Y L. Cloning, purification, and characterization of chitinase from bacillus sp. DAU101 [J]. Bioresource Technology, 2007, 98(14): 2734-2741.
[15] NASSER M S, JAMES A E. Effect of polyacrylamide polymers on floc size and rheological behaviour of kaolinite suspensions [J]. Colloids and Surfaces A: Physicochemical and Engineering Aspects, 2007, 301(1/3): 311-322.
[16] LIU Xiao-wen, HU Yue-hua, JIANG Hao, CAO Xue-feng, QIU Guang-zhou. Crystal structure property of kaolinite in hard kaolins and soft kaolins [J]. The Chinese Journal of Nonferrous Metals, 2001, 11(4): 684-687. (in Chinese)
[17] RYTWO G, TROPP D, SERBAN C. Adsorption of diquat, paraquat and methyl green on sepiolite: Experimental results and model calculations [J]. Applied Clay Science, 2002, 20(6): 273-282.
(Edited by CHEN Wei-ping)
Foundation item: Project(2005CB623701) supported by the Major State Basic Research Development Program of China
Received date: 2007-09-15; Accepted date: 2007-10-29
Corresponding author: HU Yue-hua, Professor; Tel: +86-731-8879259; E-mail: hyh@mail.csu.edu.cn