Trans. Nonferrous Met. Soc. China 26(2016) 1367-1372
Cr-poisoning under open-circuit condition in LaNi0.6Fe0.4O3-δ-based nano composite cathodes for solid oxide fuel cells prepared by infiltration process
Yeong-Ju CHOE, Jeong-Uk SEO, Kyoung-Jin LEE, Min-Jin LEE, Hae-Jin HWANG
Department of Materials Science and Engineering, Inha University, 22212, 100, Inha-ro, Nam-gu, Incheon, Korea
Received 2 July 2015; accepted 24 December 2015
Abstract: LaNi0.6Fe0.4O3-δ (LNF) powders were synthesized by the glycine-nitrate process and LNF-gadolinium-doped ceria (GDC) nanocomposite cathodes for solid oxide fuel cells (SOFCs) were fabricated by infiltration from LNF porous backbones. Electrochemical properties and Cr-poisoning behavior of LNF-GDC cathodes were studied. Single phase perovskite LNF could be obtained at the glycine to nitrate molar ratio of 1:1. The polarization resistance of the LNF-GDC nanocomposite cathode was significantly decreased in comparison with the LNF. This phenomenon was associated with enhanced catalytic activity and enlarged triple-phase boundary (TPB) length by GDC nano particles. In addition, the nanocomposite cathode showed good Cr tolerance under open circuit condition. The LNF-GDC nanocomposite cathodes were expected for use as a potential cathode in intermediate- temperature solid oxide fuel cells (IT-SOFC).
Key words: Cr-poisoning; perovskite; infiltration; solid oxide fuel cell; LaNi0.6Fe0.4O3-δ
1 Introduction
A solid oxide fuel cell (SOFC) is an all-solid-state electrochemical device that converts the chemical energy of fuels (hydrogen) and an oxidant (oxygen or air) directly into electricity. Since SOFCs operate in the high temperature range of 800-1000 °C, they have various advantages such as high efficiency, multi-fuel capability, and no requirement of noble metal catalysts compared with other fuel cells [1,2]. On the other hand, the high temperature requires the use of expensive alloys and temperature cycling can introduce mechanical stress. In addition, high temperatures promote the diffusion of constituents of the electrode into the electrolyte. In this regard, the operating temperature of SOFCs should be lowered to an intermediate temperature range (650-850 °C) and this has been one of the most important research issues in the past few decades [2,3].
Cathode materials for intermediate temperature solid oxide fuel cells (IT-SOFC) are required to have not only a high electrochemical activity but also high tolerance to chromium poisoning because of the exposure to gaseous chromium species resulting from stainless steel interconnects [4-6]. Many researchers have reported that the reaction between Mn or Sr in lanthanum strontium manganite (LSM) and lanthanum strontium cobalt ferrite (LSCF) cathodes and the gaseous chromium species is responsible for chromium poisoning and results from increased cathode polarization [6,7].
LaNi0.6Fe0.4O3 (LNF) with a perovskite structure is considered to be a promising cathode material for IT-SOFCs [8]. LNF exhibits a high electrical conductivity, compatible thermal expansion coefficient with yttrium-stabilized zirconia (YSZ) electrolyte, and good tolerance against gaseous chromium species. However, the electrochemical performance of LNF is not adequate compared with commonly used cathode materials such as LSCF and LSM [8]. In this study, a LNF-GDC nanocomposite cathode was fabricated by infiltrating GDC nanoparticles into the LNF cathode backbone. The infiltration technique is a great strategy to disperse nano-sized particles into a porous oxide backbone to improve the electrochemical activity of cathode materials [9,10]. The LaNi0.6Fe0.4O3 (LNF) powder used for the cathode backbone was synthesized by the glycine nitrate process (GNP). The electrochemical performance of symmetrical cells consisting of a GDC electrolyte and LNF-GDC nanocomposite cathodes was investigated. In addition, the Cr-poisoning behaviors of LNF and the LNF/GDC nanocomposite cathode were also examined.
2 Experimental
The LaNi0.6Fe0.4O3-δ powders were synthesized by the glycine-nitrate process shown in Fig. 1 [11]. The starting materials were La(NO3)3·6H2O (99.9%, Sigma- Aldrich, USA), Ni(NO3)2·6H2O (99.9%, Sigma-Aldrich, USA), Fe(NO3)3·9H2O (99.5%, Sigma-Aldrich, USA), and glycine (99.0%, Junsei, Japan). The metal nitrates were dissolved in distilled water and then, glycine was added into the mixed nitrate solution at fuel to nitrate molar ratios of 1:1, 1.5:1 and 0.5:1. The solution was heated to 200 °C and the combustion reaction subsequently occurred. The precursor powders were then calcined at 700 °C for 5 h.
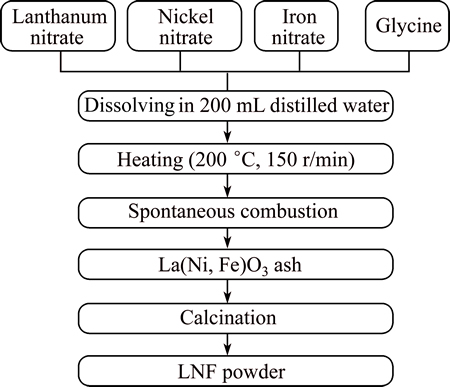
Fig. 1 Schematic diagram of synthesis of LaNi0.6Fe0.4O3-δ powders
The solution for infiltration was prepared from Gd(NO3)3·6H2O (99.9%, Sigma-Aldrich, USA), Ce(NO3)3·6H2O (99.0%, Sigma-Aldrich, USA), and ethanol. The gadolinium to cerium molar ratio was 2:8 and the concentration of the nitrate solution was 1 mol/L. The porous LNF backbone was coated on both sides of the GDC electrolyte disk (20 mm in diameter) by screen printing and was subsequently heat-treated at 1000 °C for 2 h. Infiltration was performed by dropping 30 μL of the infiltration solution into the porous LNF backbone using a micro pipet, as shown in Fig. 2. After infiltration, the sample was placed in a vacuum oven for uniform coating and homogeneous precipitation. The infiltrated samples were calcined at 350 °C for 2 h. The infiltration and calcination processes were repeated 7 times.
The Cr-poisoning behaviors of the LNF and LNF-GDC nanocomposite cathode were evaluated using the symmetrical cell exposed to the gaseous chromium species produced from the chromium oxide (Cr2O3) powder (99.0%, Junsei, Japan) compact, as shown in Fig. 3. The sample was heat-treated at 800 °C for 100 h in an atmosphere containing gaseous chromium species. The Cr2O3 powder was uniaxially pressed into a disk type and sintered at 1000 °C for 1 h. For comparison, the same sample was heat-treated at 800 °C for 100 h in a dry air atmosphere.
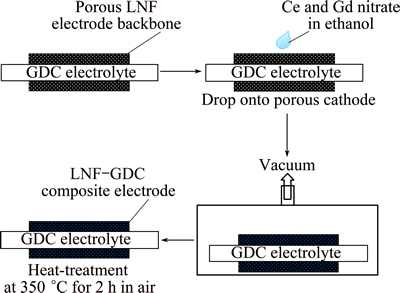
Fig. 2 Schematic illustration showing fabrication of electrode by infiltration of GDC components into porous LNF backbone and schematic of structure of LNF-GDC composite cathode
Phase identification of the synthesized powder was performed using a powder diffractometer (DMAX-2500, Rigaku, Japan) with Ni-filtered Cu Kα radiation. The microstructure of the cathode was observed by a field emission scanning electron microscope (FE-SEM, S-3200, Philips). The electrochemical performance of the symmetrical cells was measured by electrochemical impedance spectroscopy (EIS). AC impedance spectroscopy was performed with an excitation potential of 20 mV over a frequency range of 1 MHz to 0.1 Hz generated by a frequency analyzer (IM6e, Zhaner).
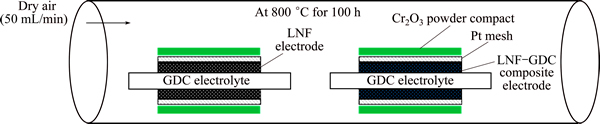
Fig. 3 Schematic diagram of Cr-poisoning experimental setup
3 Results and discussion
Figure 4 shows the XRD patterns of the LaNi0.6Fe0.4O3-δ powders calcined at 700 °C for 5 h in air. In the case of a glycine to nitrate molar ratio of 1:1, the reflections corresponding to the identified phases are labeled and closely match the JCPDS data (File No. 01-088-0637). It can be seen that the synthesized powder can be identified as a single-phase perovskite with a general formula of ABO3-δ [8,12]. On the other hand, secondary phases such as La2O, NiO and La4Ni3O10 were detected in powder samples at the glycine to nitrate molar ratios of 0.5:1 and 1.5:1. The molar ratio of glycine to nitrate is a very important parameter in the glycine nitrate process. When the molar ratio is too small (in the case of the glycine to nitrate molar ratio of 0.5:1), the perfect chelation of nitrates does not occur and this can result in inhomogeneity of the powder composition or in the appearance of unreacted starting materials. In the case of the glycine to nitrate molar ratio of 1.5:1, it seems that the excess glycine made the flame temperature too much higher, which can allow non-uniform reaction [11,13].
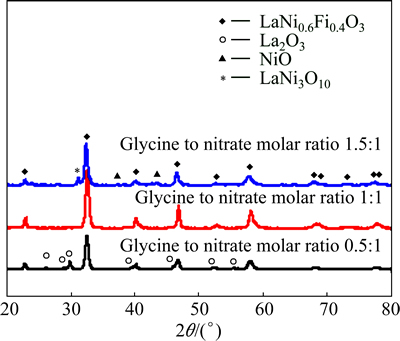
Fig. 4 XRD patterns of synthesized LaNi0.6Fe0.4O3-δ powders calcined at 700 °C as function of glycine to nitrate molar ratio
Figures 5 (a) and (b) show the impedance spectra of the LNF cathodes and GDC-infiltrated LNF (LNF-GDC) composite cathodes, respectively, as a function of temperature. The polarization resistances of the LNF and LNF-GDC nanocomposite cathodes decreased with increasing temperature. The LNF-GDC nanocomposite cathodes showed low polarization resistances at all temperatures. For example, the polarization resistance decreased from 84.8 to 0.35
·cm2 at 650 °C. This result indicates that the GDC nanoparticles are effective in enhancing the catalytic activity for the oxygen reduction reaction at the cathode. The infiltrated GDC nanoparticles cause the triple-phase boundary (TPB)
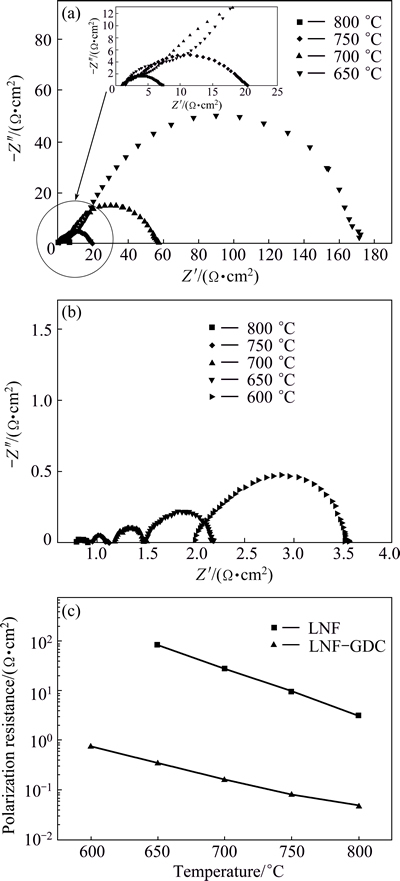
Fig. 5 Impedance spectra of LNF (a) and GDC-infiltrated LNF composite cathodes (b) as function of temperature, Arrhenius plots of polarization resistances of LNF and LNF-GDC composite cathodes (c)
length to enlarge and the conducting pathway to connect, which facilitates high electrochemical performance and effective charge transport [9]. There are two basic types of infiltration methods, structures of ionic conductor particles with an electronic conductor backbone and electronic conductor particles with an ionic conductor backbone [10,14,15]. In this study, the LNF-GDC cathodes correspond to the former case. The advantage of infiltrating the electrolyte nanoparticles into the electrode backbone is that there is no requirement to heat them at high temperatures over 850 °C in order to form the perovskite phases [16]. It also reduces coarsening of the infiltrated nanoparticles compared with the electrolyte backbone.
Cross-sectional and high magnification SEM images of the LNF and LNF-GDC nanocomposite cathodes are shown in Fig. 6. As evident in Figs. 6(a) and (c), the thickness of the cathodes was ~23 μm and the cathodes exhibited porous microstructures in good interfacial contact with the GDC electrolyte, with no signs of delamination. The infiltrated GDC nanoparticles were homogeneously distributed in the LNF backbone and the size of the GDC was estimated to be approximately a few tens of nanometer, as can be seen in Fig. 6(d). It appears that both LNF and GDC make a 3-dimension network structure which resulted in the enhanced catalytic activity of the LNF-GDC nanocomposite cathode.
To analyze the chromium poisoning behavior under open circuit condition, the polarization resistances of the LNF/GDC/LNF and LNF-GDC/GDC/LNF-GDC cells were measured before and after exposure to gaseous chromium species. The results are shown in Fig. 7 and Table 1. Almost the same polarization resistance was obtained in the two cases in spite of the exposure to chromium vapors, which demonstrates that the LNF and LNF-GDC composite cathodes have high tolerance to Cr-poisoning, as reported by other researchers [8,17,18]. In our previous study, there is a degradation of LSCF electrode under same condition [19]. Although there is literature about degradation of electronic conductivity of LNF under current load because of the nickel chromate formation by nickel segregation, it was reported to have no significant change on the cathode performance in comparison to the LSM [18]. Chromium deposition is generally observed at the electrode/electrolyte interface in the case of materials vulnerable to chromium such as LSM and LSCF [6,7,17]. ZHEN et al [8] reported that there was no visible Cr deposition at 900 °C at the LNF/YSZ interface in the presence of a Fe-Cr alloy.
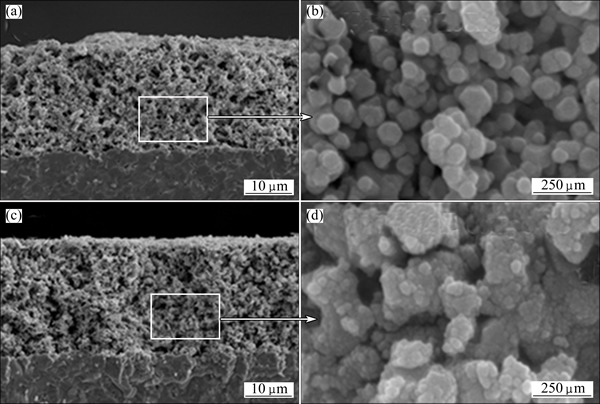
Fig. 6 SEM images of LNF (a, b) and GDC-infiltrated LNF electrode (c, d)
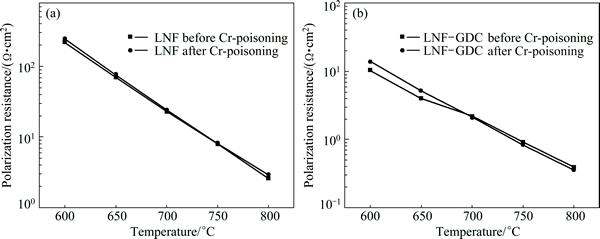
Fig. 7 Arrhenius plots of polarization resistances (Rp) of LNF (a) and LNF-GDC composite cathodes (b) before and after Cr-poisoning
Table 1 Polarization resistances of LNF and LNF-GDC composite cathodes before and after Cr-poisoning
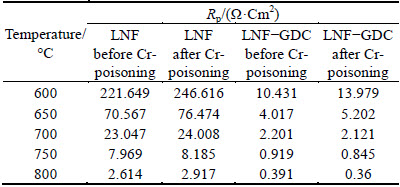
After the cells were heat-treated at 800 °C for 100 h in the atmosphere consisting of gaseous chromium species, the LNF backbone and GDC particles were well adhered. Also, degradation of the electrochemical performance was not observed in the LNF cathodes or the LNF-GDC composite cathodes shown in Fig. 7. Although the polarization resistance of the LNF-GDC nanocomposite cathode increased after heat treatment at 800 °C in air, this phenomenon resulted from coarsening of infiltrated GDC particles at high temperature. The LNF-GDC nanocomposite has potential for use in IT-SOFC cathodes with a high tolerance to Cr-poisoning. Although Ni chromate can form by nickel segregation, it was reported to have a negligible effect on the cathode performance [20].
To examine the reaction between LNF and gaseous chromium species, we confirmed the phase change before and after Cr-poisoning, and the resulting XRD patterns are shown in Fig. 8. There were no additional peaks due to the reaction between the LNF or GDC and the gaseous chromium species. Compared with reports in Refs. [21,22] in which the LSCF reacts with the gaseous chromium species to produce strontium chromite, it seems that LNF is a relatively stable material against Cr vapors, which is related to the almost same polarization resistance obtained before and after Cr-poisoning.
4 Conclusions
LaNi0.6Fe0.4O3-δ (LNF) powders were synthesized by glycine nitrate process (GNP) and LNF-GDC nanocomposite cathodes were fabricated by infiltration from the LNF porous backbone. The catalytic activity of the LNF-GDC nanocomposite cathode was significantly improved compared with that of the LNF cathode. The addition of GDC into the LNF cathode resulted in an enhanced ionic conductivity and triple-phase boundaries. Although the polarization resistances of the LNF-GDC nanocomposite cathodes were slightly increased after heat treatment at 800 °C for 100 h, they were much lower than that of the LNF cathode. It was found that the nanocomposite cathode showed good durability against Cr-poisoning under open-circuit condition and can be considered for use as a potential cathode in intermediate-temperature solid oxide fuel cells (IT-SOFC).
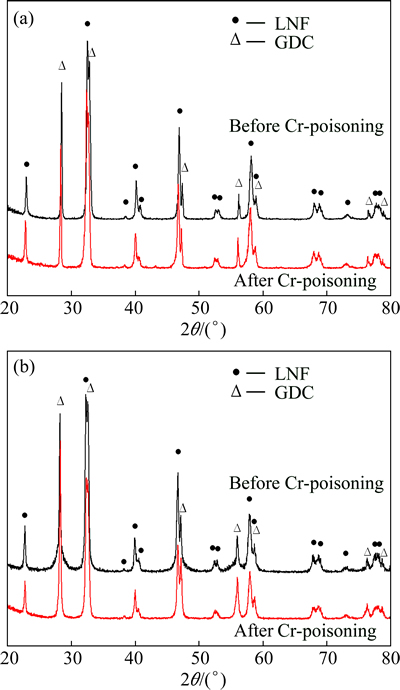
Fig. 8 XRD patterns of LNF (a) and LNF-GDC composite cathodes (b) before and after Cr-poisoning
Acknowledgments
This research was supported by a grant from the Fundamental R&D Program for Core Technology of Materials (No.10051006) funded by the Ministry of Knowledge Economy, Republic of Korea, and supported by the New & Renewable Energy Core Technology Program of the Korea Institute of Energy Technology Evaluation and Planning (KETEP), granted financial resource from the Ministry of Trade, Industry & Energy, Republic of Korea (No. 20113020030050).
References
[1] ATKINSON A, BARNETT S, GORTE R J, IRVINE J, MCEVOY A J, MOGENSEN M, SINGHAL S C, VOHS J. Advanced anodes for high-temperature fuel cells [J]. Nature Materials, 2004, 3(1): 17-27.
[2] FERGUS J W. Oxide anode materials for solid oxide fuel cells [J]. Solid State Ionics, 2006, 177(17): 1529-1541.
[3] LUO Zhi-an, XIAO Jian-zhong, XIA Feng, YANG Yi-fan. Steady polarization process modelling of noble metal-electrolyte cermet composite electrode [J]. Transactions of Nonferrous Metals Society of China, 2006, 16(1): 142-147.
[4] XIA Wei-sheng, YANG Yun-zhen, ZHANG Hai-ou, WANG Gui-lan. Fabrication and electrochemical performance of solid oxide fuel cell components by atmospheric and suspension plasma spray [J]. Transactions of Nonferrous Metals Society of China, 2009, 19(6): 1539-1544.
[5] HUIJSMANS J, van BERKEL F, CHRISTIE G. Intermediate temperature SOFC—A promise for the 21st century [J]. Journal of Power Sources, 1998, 71(1): 107-110.
[6] JIANG S P, CHEN X. Chromium deposition and poisoning of cathodes of solid oxide fuel cells—A review [J]. International Journal of Hydrogen Energy, 2014, 39(1): 505-531.
[7] TUCKER M C, KUROKAWA H, JACOBSON C P, de JONGHE L C, VISCO S J. A fundamental study of chromium deposition on solid oxide fuel cell cathode materials [J]. Journal of Power Sources, 2006, 160(1): 130-138.
[8] ZHEN Y, TOK A, JIANG S, BOEY F. La(Ni,Fe)O3 as a cathode material with high tolerance to chromium poisoning for solid oxide fuel cells [J]. Journal of Power Sources, 2007, 170(1): 61-66.
[9] JIANG Z, XIA C, CHEN F. Nano-structured composite cathodes for intermediate-temperature solid oxide fuel cells via an infiltration/impregnation technique [J]. Electrochimica Acta, 2010, 55(11): 3595-3605.
[10] JIANG S P. Nanoscale and nano-structured electrodes of solid oxide fuel cells by infiltration: Advances and challenges [J]. International Journal of Hydrogen Energy, 2012, 37(1): 449-470.
[11] CHICK L A, PEDERSON L, MAUPIN G, BATES J, THOMAS L, EXARHOS G. Glycine-nitrate combustion synthesis of oxide ceramic powders [J]. Materials Letters, 1990, 10(1): 6-12.
[12] LEE S, KIM J, SON J, LEE J, KIM B, JE H, LEE H, SONG H, YOON K J. High performance air electrode for solid oxide regenerative fuel cells fabricated by infiltration of nano-catalysts [J]. Journal of Power Sources, 2014, 250: 15-20.
[13] JIANG S P, WANG W. Fabrication and performance of GDC-impregnated (La,Sr)MnO3 cathodes for intermediate temperature solid oxide fuel cells [J]. Journal of Electrochemical Society, 2005, 152(7): A1398-1408.
[14] LEE S, BEVILACQUA M, FORNASIERO P, VOHS J M, GORTE R J. Solid oxide fuel cell cathodes prepared by infiltration of LaNi0.6Fe0.4O3 and La0.91Sr0.09Ni0.6Fe0.4O3 in porous yttria-stabilized zirconia [J]. Journal of Power Sources, 2009, 193(2): 747-753.
[15] PENG R, FAN X, JIANG Z, XIA C. Characteristics of La0.85Sr0.15MnO3–d powders synthesized by a glycine-nitrate process [J]. Fuel Cells, 2006, 6(6): 455-459.
[16] PARK E, TANIGUCHI S, DAIO T, CHOU J, SASAKI K. Influence of cathode polarization on the chromium deposition near the cathode/electrolyte interface of SOFC [J]. International Journal of Hydrogen Energy, 2014, 39(3): 1463-1475.
[17] KOMATSU T, ARAI H, CHIBA R, NOZAWA K, ARAKAWA M, SATO K. Cr-poisoning suppression in solid oxide fuel cells using LaNi(Fe)O3 electrodes [J]. Electrochemical and Solid-state Letters, 2006, 9(1): A9-A12.
[18] CHOE Y, HWANG H. Electrochemical performance and Cr tolerance in a La1-xBaxCo0.9Fe0.1O3-δ (x=0.3, 0.4 and 0.5) cathode for solid oxide fuel cells [J]. Journal of the Korean Ceramic Society, 2015, 52(5): 1-7.
[19] ANDREAS SCHULER J, LUBBE H, HESSLER-WYSER A, van HERLE J. Nd-nickelate solid oxide fuel cell cathode sensitivity to Cr and Si contamination [J]. Journal of Power Sources, 2012, 213: 223-228.
[20] KONYSHEVA E, PENKALLA H, WESSEL E, MERTENS J, SEELING U, SINGHEISER L, HILPERT K. Chromium poisoning of perovskite cathodes by the ODS alloy Cr5Fe1Y2O3 and the high chromium ferritic steel Crofer22APU [J]. Journal of Electrochemical Society, 2006, 153(4): A765-773.
[21] LAU G Y, TUCKER M C, JACOBSON C P, VISCO S J, GLEIXNER S H, DEJONGHE L C. Chromium transport by solid state diffusion on solid oxide fuel cell cathode [J]. Journal of Power Sources, 2010, 195(22): 7540-7547.
[22] TAKESHI K, YOSHITERU Y, KIMITAKA W, REIICHI C, HIROAKI T, HIMEKO O, HAJIME A. Degradation behavior of anode-supported solid oxide fuel cell using LNF cathode as function of current load [J]. Journal of Power Sources, 2010, 195(17): 5601-5605.
熔渗法制备固体氧化燃料电池LaNi0.6Fe0.4O3-δ基纳米复合阴极在开路条件下的铬中毒
Yeong-Ju CHOE, Jeong-Uk SEO, Kyoung-Jin LEE, Min-Jin LEE, Hae-Jin HWANG
Department of Materials Science and Engineering, Inha University, 22212, 100, Inha-ro, Nam-gu, Incheon, Korea
摘 要:利用甘氨酸-硝酸盐方法合成LaNi0.6Fe0.4O3-δ(LNF)粉末,采用LNF多孔主干熔渗法制备掺杂二氧化铈LNF-钆(GDC)固体氧化燃料电池纳米复合阴极。研究了LNF-GDC纳米复合阴极的电化学性能和铬中毒行为。当甘氨酸和硝酸盐的摩尔比为1:1时可得到单相钙钛矿LNF。与LNF相比,LNF-GDC纳米复合阴极的极化阻抗显著降低,这与增强的催化活性和增大的三相边界长度有关。另外,在开路条件下,纳米复合阴极有较好的铬容限。在中温固体氧化燃料电池中,LNF-GDC纳米复合阴极有望成为一种颇具潜力的阴极材料。
关键词:铬中毒;钙钛矿;熔渗;固体氧化燃料电池;LaNi0.6Fe0.4O3-δ
(Edited by Xiang-qun LI)
Corresponding author: Hae-Jin HWANG; Tel: +82-32-860-7521; Fax: +82-32-862-4482; E-mail: hjhwang@inha.ac.kr
DOI: 10.1016/S1003-6326(16)64240-1