J. Cent. South Univ. Technol. (2010) 17: 28-33
DOI: 10.1007/s11771-010-0006-6 
Ignition-proof mechanism of ZM5 magnesium alloy added with rare earth
RAO Jin-song(饶劲松), LI Hua-ji(李华基), XUE Han-song(薛寒松)
School of Materials Science and Engineering, Chongqing University, Chongqing 400044, China
? Central South University Press and Springer-Verlag Berlin Heidelberg 2010
Abstract: The ignition-proof mechanism of ZM5 magnesium alloy added with 0.1% (mass fraction) rare earth (RE) was investigated. The oxide scales and substrates were characterized by scanning electronic microscope (SEM), X-ray diffraction (XRD), energy dispersive spectrometer (EDS) and tensile test. And an oxidation model of ZM5 alloy with RE was established. The results show that the ignition temperature of ZM5 alloy is particularly elevated from 654 to 823 ℃, the microstructure is refined, and the tensile strength is slightly improved from 168.2 to 174.6 MPa by adding 0.1% RE. A double-layer oxidation film formed on the alloy surface under high temperature mainly consists of MgO, RE2O3 and Al2O3, which is 2.5-3.5 ?m in thickness. It is found that the forming of protective oxidation film on the thermodynamics is attributed to RE elements congregating on the surface of molten Mg alloy.
Key words: rare earth (RE); magnesium alloy; oxidation film; ignition-proof
1 Introduction
Recently, with the development of the automobile and electronics industries, magnesium (Mg) alloys have been increasingly studied due to their excellent properties such as low density, high specific strength, specific rigidity and good damping property. However, their further applications are limited because of the problems of oxidation and burning during melting and casting [1-3]. Fluxes and cover gases are currently used in the melting and production processes, which need complex equipment and are prone to make casting impure, and pollute the environment. The alloying, a promising way to solve the problem of Mg alloys’ oxidation and burning, can reduce the complex of equipment and process, and will not cause any pollution. According to relevant reports, magnesium alloy can be prevented from burning by the addition of calcium (Ca), however, the alloy is prone to be hot-tear by the addition of calcium, and the excessive calcium can deteriorate the mechanical properties of the alloy. Beryllium (Be) is also one of important alloying elements that can enhance the ignition-proof performance of the magnesium alloys. However, Be is poisonous, and excessive Be can also damage mechanical properties. Therefore, the applications of alloying with Be or Ca have been greatly limited [4-8].
Rare earth elements have special arrange of electrons and play an important role in material area. Rare earths are often used as alloying elements added to alloys to get good performance [9-13]. It was shown that the ignition temperature could be greatly elevated with adding a certain amount of RE (rare earth optimized in composition) into ZM5 magnesium alloy in our previous research. When 0.1% RE (mass fraction) is added, the ignition-proof effect is the greatest and the ignition temperature is increased from 654 to 823 ℃, When raising the addition of RE beyond 0.1%, the ignition temperature would decrease gradually [14]. Based on the aforementioned research, the properties of oxidation film on the surface of ZM5 alloy added with 0.1% RE (Hereinafter, it is called ZM5-0.1%RE) were investigated, and the oxidation model of the ignition-proof mechanism was established in this work.
2 Experimental
Materials used in this experiment were as follows (mass fraction): 99.5% pure Mg, 99.7% industry pure Al, Al-10%Mn master alloy, and Al-10%RE master alloy. The temperature of the molten metal was tested by NiCr-NiSi thermocouples. The change of the temperature was recorded by a WXT-604 desk recording device. ZM5-0.1%RE alloy was melted in a graphite crucible by an electric resistance furnace. Chemical compositions (mass fraction) of the self-made fluxes used in the course of the experiments were 60%MgCl2, 30%KCl, 5%BaCl2 and 5%CaF2. 0.1%RE was added into the molten alloy at about 750 ℃. The temperature was maintained and the molten alloy was stirred evenly to ensure the complete melting of the alloy. The fluxes on the surface were removed lightly at 730 ℃. When the red molten liquid was all covered by a sheet of silvery film that could prevent the molten alloy from ignition, the molten alloy was cooled quickly to the room temperature.
The oxidation surface was sliced from the cooling specimen, and the microscopic morphology of oxidation film was observed by scanning electronic microscope (SEM, JSM-6460LV). The phases were checked by X-ray diffractometer (XRD, D/MAX1400) and the butt of diffractometer is made of Cu Kα.
The specimen was cut vertically from the oxidation layer, polished and etched, and then photographed under SEM. During the process the surface of oxidation film was protected by the resin in order to prevent the film from flaking off. The elements distribution of oxidation film in the depth direction was analyzed by the energy dispersive spectrometer (EDS) that is the accessory of SEM.
The gravity casting standard specimens by ingot mold were tested to get mechanical properties. The tensile strength and the elongation were the average data got by more than three times of tensile test. The microstructures of the specimens were observed by metallographic microscope.
3 Results and discussion
3.1 Microscopic properties of oxidation film
Fig.1(a) and Fig.1(b) show the microscopic morphologies of the oxidation films on the surfaces of ZM5 alloy without the addition of RE and with the addition of 0.1%RE, respectively. There are a lot of small holes on the oxidation film of ZM5 alloy without the addition of RE, which makes the oxidation film loose. However, it can be distinctly observed that the film of ZM5-0.1%RE is denser and no hole appears on the surface. The XRD pattern of the surface oxidation film of ZM5-0.1%RE alloy is shown in Fig.2, which reveals that the oxidation film consists of MgO, Al2O3 and (RE)2O3. The main intermetallic phase is recognized as Mg17Al12 resulting from the matrix of the alloy by XRD.
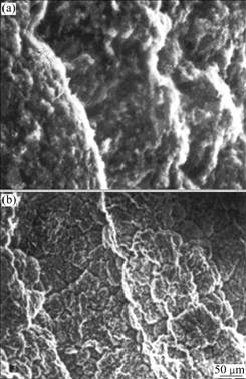
Fig.1 SEM images of ZM5 alloy without RE (a) and with 0.1%RE (b)
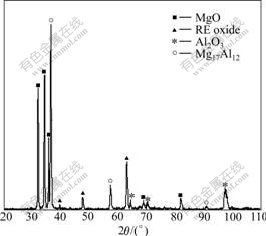
Fig.2 XRD pattern of surface oxidation film of ZM5-0.1%RE alloy
In the molten alloy of ZM5-0.1%RE, the content of Mg is about 90% (mass fraction), and the vapor tension is very high. So firstly Mg reacts with oxygen to form MgO deposited on the surface of the molten metal. According to Pilling-Bedworth principle of oxidation, the essential condition of forming tightly coherent oxidation film on the surface of oxidizing metal is that the volume ratio η (η is the ratio of the oxide volume to the volume of the original metal that is consumed to form the oxide) is greater than 1. That is to say, when η>1, the oxidation film can expand to bring compressive stress and the oxidation film becomes dense under the action of the compressive stress. The η value of Mg is 0.81, so the film of MgO is loose (Fig.1(a)). Oxygen can easily infiltrate into the molten alloy through this loose film during the melting process, which can result in further oxidation even burning. However, because the atomic affinity energy of RE—O is lower than that of Mg—O (the atomic affinity energies of Mg, La and Ce with O are -14.933 8, -19.060 8 and -19.505 0 eV, respectively) [15], the RE elements preferentially combine with O and form RE oxides when oxygen infiltrates in. In the meantime a little Al2O3 is generated with the reaction of aluminum and oxygen. Thus, the oxidation film mainly consisting of MgO, RE2O3 and Al2O3 is formed. Because η values of the RE oxides are greater than 1, the RE oxides can expand to fill in the holes of MgO to form tightly coherent film (Fig.1(b)) that can restrain oxygen from infiltrating into the molten alloy effectively. Thus, the molten alloy is prevented from further oxidizing, and the ignition temperature is elevated.
Fig.3 shows the cross-section picture of the oxidation film on the ZM5-0.1%RE alloy observed by SEM. The brighter part is the oxidation layer, the gray one is the matrix of the alloy, and the dark one is the resin. It is found that the thickness of the oxidation layer is 2.5-3.5 ?m. Four points across the oxidation film are selected to analyze the elements’ distribution in the depth direction of the oxidation film, as shown in Fig.3. Point 1 is close to the surface of film, point 2 is in the oxide layer, point 3 is close to the matrix, and point 4 is the matrix. Fig.4 reveals the corresponding energy spectrum analysis pictures of points 1-4 obtained by EDS.
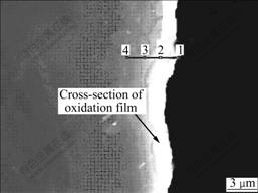
Fig.3 Cross-section picture of ZM5-0.1%RE alloy
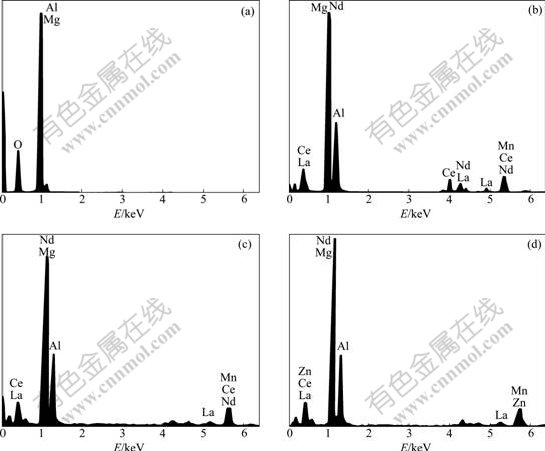
Fig.4 Energy spectrum analysis pictures of points 1-4: (a) Point 1; (b) Point 2; (c) Point 3; (d) Point 4
According to Hume Rothery Law, if the atom radius ratio of A to B is more than 15%, they can form only a small amount of solid solution. Because the atom radius ratio of RE to that of Mg is more than 15%, the solubility of RE in Mg is small (K<<1, K is the equation distribution coefficient), and it is called “surface active element” [16-17]. The elements’ distribution in the depth direction of the oxidation film is shown in Table 1 according to the EDS results, which apparently proves the obvious congregating of RE elements on the surface of the molten alloy. For example, at point 2, the mass fraction of RE is 14.32%, which is much higher than the average value of the alloy (0.1%).
Table 1 Elements distribution in depth direction of oxidation film
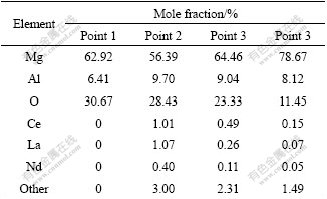
3.2 Thermodynamic analysis
The possible chemical reactions occurring on the surface of the molten ZM5-0.1%RE alloy when the fluxes were removed at 730 ℃ are as follows:
2Mg(l)+O2(g)=2MgO(s) (1)
4RE(l)+3O2(g)=2RE2O3(s) (2)
3MgO+2RE(l)=RE2O3(s)+3Mg(l) (3)
The standard Gibbs free energy changes of reactions (1), (2) and (3) are marked with
and
respectively.
(4)
RE used in this research consists of Ce, La and Nd. For the three different kinds of rare earth, the relations between
and reaction temperature T are as follows.
Ce:
-7 742+3TlgT-479.70T (5)
La:
14 182+3TlgT-452.0T (6)
Nd:
2 258+3TlgT-485.85T (7)
When the temperature is 730 ℃, i.e. T=1 003 K, the results of Eqs.(5), (6) and (7) are as follows.
Ce:
-479 850.19 J/mol,
La:
-430 213.3J/mol,
Nd:
-476 018.64 J/mol.
According to the isothermal equation of chemical reaction,
(8)
where
denotes the activity of matter, whose value is 1 to solid matter. Plugging 
=1 into Eq.(8), the following equation is concluded:
(9)
When
is increased, ΔG3 decreases. According to the previous analysis, RE congregates on the surface of the molten alloy, which indicates that the activity of RE on the surface is much higher than that inside. The concentrations of the elements on the alloy surface are shown in Table 1 and the composition at point 2 can be regarded as the concentrations of RE and Mg when reaction (3) occurs. In the dilute solution of ZM5- 0.1%RE at 1 003 K, the solute (RE) follows Henry law, and K (Henry law constant) can be recognized as 0.14. Thus, there exist
and
At the same time, the solvent (Mg) follows Raoult law, and
[18]. Thus, according to Eq.(9) it can be deduced that ΔG3 is negative whether RE is Ce, La or Nd, which indicates that reaction (3) is a spontaneous process. So only 0.1% addition of RE can form tightly coherent and protective film on the surface of ZM5-0.1%RE alloy.
3.3 Model of oxidation film
As shown in Table 1, the element distribution in the depth direction of the oxidation film is uneven. The more oxygen and Mg atoms appear at position close to the surface of oxidation film (point 1), which indicates that the surface has more MgO and a little Al2O3. At point 2, the contents of RE elements increase greatly, that is to say, the content of (RE)2O3 increases at this point. At points 3 and 4, while Mg atoms increase, the number of oxygen and RE atoms reduces greatly, which proves that the oxide decreases. At point 4, the element composition is very close to the matrix. It is shown from the forming process and the elements’ distribution that the oxidation film can be approximately divided into two layers: the outer layer formed mainly by the initial oxidation of Mg is loose and consists of MgO and little Al2O3; the inner layer formed when oxygen infiltrates in through the outer layer is compact and consists of MgO, RE2O3 and Al2O3. The good ignition-proof effect is mainly attributed to the inner layer. The oxidation model of ZM5-0.1%RE alloy is shown in Fig.5.
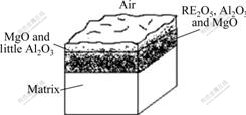
Fig.5 Oxidation model of ZM5-0.1%RE alloy
3.4 Ignition-proof failure of oxidation film
The oxidation film of the metal, whose η value is greater than 1, will expand continually with the growth of the film. It can generate not only compressive internal stress paralleling with the direction of the alloy surface but also tensile stress that is vertical to the surface and can make oxidation film stay away from base metal. If the compressive stress goes beyond the intensity of the film, the cracks of film layer will appear. If the tensile stress is larger than the adhesive force, the film will fall from the metal surface.
Consequently, the quality of oxidation film is different with different amounts of RE in Mg alloy. When RE is less, the RE oxide cannot fill in the holes of the beginning MgO film completely, so the effect of ignition-proof is limited. When RE is excessive, the enrichment of RE elements on the surface is more serious, the oxidation film will swell excessively with the growth of the film. When the internal stress goes beyond the film’s intensity, the cracks of film will appear, leading to ignition-proof failure. So only the appropriate addition has good ignition-proof effect.
3.5 Effect of RE on microstructure and mechanical properties of ZM5 alloy
As shown in Fig.6, the microstructure of ZM5 alloy is remarkably refined by the addition of 0.1% RE. The mean grain size of the ZM5-0.1%RE alloy is about 25 ?m and much less than that of the ZM5 alloy. It is also seen that more eutectic phases are formed at the grain boundaries of the ZM5-0.1%RE alloy and the distance of the secondary dentrite is shorter due to the increasing supercooling, which indicates a little enhancement in tensile strength (σb) and elongation (δ5) compared with ZM5 alloy. Table 2 shows the mechanical properties of ZM5 and ZM5-0.1%RE alloys. It is distinct that the tensile strength of ZM5-0.1%RE is higher than that of ZM5 alloy without RE, and the elongation is also enhanced obviously. RE elements have so great effects on the performance of magnesium alloy, which can not only form the protective oxidation film but also improve the microstructure and mechanical properties.
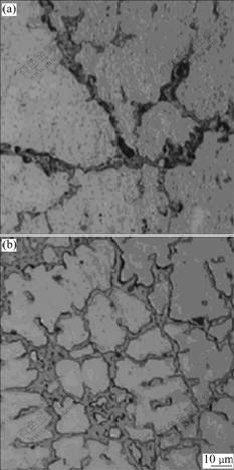
Fig.6 Microstructures of ZM5 alloys without RE (a) and with 0.1%RE (b)
Table 2 Mechanical properties of as-cast specimens
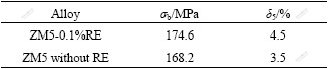
4 Conclusions
(1) A compact oxidation film is formed on the ZM5 alloy with 0.1% RE exposed to the high temperature air, which can isolate the inner metal from the outer oxygen and prevent further oxidation.
(2) The oxidation film is 2.5-3.5 ?m in thickness, and can be regarded as double-layer structure. The outer layer consists of MgO and little Al2O3, and is loose; the inner layer consists of MgO, RE2O3 and Al2O3, and is compact. The good ignition-proof effect is mainly attributed to the inner dense layer.
(3) RE elements congregate on the surface of molten Mg alloy obviously, which provides favorable thermodynamic condition to form compact oxidation film, so even just a small amount of addition (0.1%) of RE can form a layer of protective film.
(4) The mechanical properties are also improved by the addition of RE due to the increase of ignition resistance.
(5) An oxidation model of ZM5-0.1%RE alloy is established. In the future research, the regeneration ability of the protective oxidation film after being destroyed will be investigated in order to perfect the ignition-proof mechanism of magnesium alloy added with RE.
References
[1] RAVI N V, BLANDIN J J. Effect of alloying elements on the ignition resistance of magnesium alloys [J]. Scripta Materialia, 2003, 49(3): 225-230.
[2] XIONG Chuang-xian, ZHANG Xin-ming. Effects of cryogenic treatment on mechanical properties of extruded Mg-Gd-Y-Zr(Mn) alloys [J]. Journal of Central South University of Technology, 2007, 14(3): 305-309.
[3] YI Jian-long, ZHANG Xin-ming. Corrosion resistance of cerium conversion film electrodeposited on Mg-Gd-Y-Zr magnesium alloy [J]. Journal of Central South University of Technology, 2009,16(1): 38-42.
[4] SHIH T S, WANG J H. Combustion of magnesium alloys in air [J]. Materials Chemistry and Physics, 2004, 85: 302-309.
[5] SHIH T S, LIU J B. Oxide films on magnesium and magnesium alloys [J]. Materials Chemistry and Physics, 2007, 104(2): 497-504.
[6] LEE J K, JO H H, KIM S K. Effect of CaO addition on ignition behavior in molten AZ31 and AZ91D magnesium alloys [J]. Rare Metals, 2006, 25(S): 155-159.
[7] MEBARKI N, RAVI N V. Correlation between ignition and oxidation behaviours of AZ91 magnesium alloy [J]. Material Science and Technology, 2005, 21(10): 1145-1151.
[8] ZENG Xiao-qing, WANG Qu-dong. Behavior of surface oxidation on molten Mg-9Al-0.5Zn-0.3Be alloy [J]. Material Science and Engineering A, 2001, 301: 154-161.
[9] BLANDIN J J, GROSJEAN E. Ignition resistance of various magnesium alloys [C]// Magnesium Technology 2004. Charlotte: Minerals, Metals and Materials Society, 2004: 235-240
[10] CHEN Zhi-hong, REN Xue-ping. Effect of RE on the ignition-proof, microstructure and properties of AZ91D magnesium alloy [J]. Journal of University of Science and Technology Beijing: Mineral, Metallurgy, Materials, 2005, 12(6): 540-544.
[11] WU W H, XIA C Q. Microstructures and mechanical properties of Mg-Ce-Zn-Zr wrought alloy [J]. Journal of Central South University of Technology, 2004, 11(4): 367-370.
[12] LIN Peng-yu, ZHOU Hong. Interactive effect of cerium and aluminium on the ignition point and the oxidation resistance of magnesium alloy [J]. Corrosion Science, 2008, 50: 2669-2675.
[13] LI Hua-ji, XUE Han-song. Effects of lanthanum, cerium and lanthanum rich mischmetal on ignition temperature of magnesium alloy [J]. Journal of Rare Earths: English Edition, 2001, 19(S): 188-122.
[14] ZOU Yong-liang, LI Hua-ji. Effect of mischmetal additions on the ignition temperature of molten ZM5 magnesium alloy [J]. Journal of Chongqing University, 2003, 26(5): 33-37. (in Chinese)
[15] LIU Gui-li. Theoretical study of ignition-proof mechanism of RE element in Mg alloys [J]. Acta Physica Sinica, 2008, 57(1): 434-437. (in Chinese)
[16] HU Zhong, ZHANG Qi-xun, GAO Yi-xi. Casting technology and quality controlling of aluminum and magnesium alloy [M]. Beijing: Aerospace Industry Press, 1990. (in Chinese)
[17] LIU Jin-ping, LI Hua-ji, XUE Han-song. Study on ignition-proof AZ91D magnesium alloy added with rare earth [J]. Journal of Rare Earths: English Edition, 2004, 22(S): 117-121.
[18] LUO Zhi-ping, ZHANG Shao-qing. Thermodynamic analysis of rare earth in magnesium alloy [J]. Journal of Rare Earths, 1995, 13(2): 119-122. (in Chinese)
Foundation item: Project(2004BB8429) supported by Chongqing Municipal Science and Technology Commission, China
Received date: 2009-02-10; Accepted date: 2009-05-11
Corresponding author: RAO Jin-song, Doctoral candidate; Tel: +86-23-65102345; E-mail: rjs@cqu.edu.cn
(Edited by YANG You-ping)