J. Cent. South Univ. Technol. (2011) 18: 78-82
DOI: 10.1007/s11771-011-0662-1

Electrochemical performance of interfacially polymerized polyaniline nanofibres as electrode materials for non-aqueous redox supercapacitors
LI Jie(李劼), FANG Jing(方静), CUI Mu(崔沐), LU Hai(卢海),
ZHANG Zhi-an(张治安), LAI Yan-qing(赖延清)
School of Metallurgical Science and Engineering, Central South University, Changsha 410083, China
? Central South University Press and Springer-Verlag Berlin Heidelberg 2011
Abstract: H+ doped polyaniline nanofibre (PH) was synthesized by interfacial polymerization and polyanilines doped with Li salt (PLI and PHLI) were prepared by immersing emeraldine base (EB) and H+ doped polyaniline in 1 mol/L LiPF6/(EC-EMC-DMC), respectively. PH, PLI and PHLI were all characterized by scanning electron microscopy (SEM) and Fourier transform infrared (FT-IR) spectrometry. With 1 mol/L LiPF6/(EC-EMC-DMC) as electrolyte, PH, PHLI and PLI were used as the active materials of symmetric non-aqueous redox supercapacitors. PLI shows the highest initial specific capacitance of 120 F/g (47 F/g for PH and 66 F/g for PHLI) among three samples. After 500 cycles, the specific capacitance of PLI remains 75 F/g, indicating the good cycleability.
Key words: polyaniline nanofibre; redox supercapacitor; interfacial polymerization; lithium salt doping
1 Introduction
Supercapacitors have attracted growing attention for the use of electrical energy storage systems because of their higher specific power and longer cyclic life than batteries, and higher specific energy than conventional capacitors. Generally, supercapacitors can be divided into two types on the basis of charge storage mechanism: electric double-layer capacitor (EDLC) and redox pseudo-capacitor [1-3]. Transition metal oxide or conducting polymers can be used as electrode materials for the redox pseudo-capacitors. Among conducting polymers, polyaniline (PANI) has been most actively investigated due to its low cost, ease of synthesis, high conductivity and stability.
Different morphologies of PANI have been achieved with various methods. Generally, granular PANI can be obtained by conventional chemical or electrochemical polymerization in proton acids [4-5]; while for nanostructured polyaniline, it can be obtained by the methods of template synthesis [6], self-assembly [7], emulsion [8], electrochemical synthesis [9], interfacial polymerization [10], etc. Among them, interfacial polymerization is a simple, practical method in which fibrillar morphology polyaniline can grow at liquid/liquid interface [11]. With high surface area and high porosity, these polyaniline nanofibres can possibly provide better performances as the electrode materials of supercapacitors because of their distinctive characteristics of conducting pathways, surface interactions and nanoscale dimensions.
Polyaniline is conventionally doped with protonic acid, such as H2SO4 or HCl, to have fast charge-discharge kinetics in aqueous redox supercapacitors [12-13]. It has also been studied as electrode material in non-aqueous redox supercapacitors, which is regarded as the certain route to get higher specific energy by elevating the working voltage for redox supercapacitors. It is reported that Li salts doped polyaniline synthesized by chemical method has been used as electrode material in non-aqueous redox supercapacitors [14-16]. However, polyaniline nanofibres obtained by the interfacial polymerization for non-aqueous supercapacitors have been rarely studied.
In the present work, PANI nanofibres were obtained by interfacial polymerization, and lithium salt doped polyaniline nanofibres were prepared by treating the fibers with the non-aqueous solutions. The products were characterized by FT-IR and SEM. The electrochemical capacitance behaviors in 1 mol/L LiPF6 non-aqueous solution were tested by constant-current charge- discharge, cyclic voltammetry and impedance measurements.
2 Experimental
2.1 Interfacial polymerization of PANI nanofibres
Polyaniline nanofibre was synthesized by interfacial polymerization described as follows. 0.02 mol of aniline was added to 100 mL CCl4, while ammonium persulfate of 1.14 g was dissolved in 100 mL of 1 mol/L solution of HCl. The two solutions were carefully transferred into a beaker to form an interface between the two layers. After 1-3 min, green polyaniline was observed at the interface and then gradually diffused into the aqueous phase. The reaction system was kept undisturbed at 25 °C for 5 h. Meanwhile, the entire water phase was filled homogeneously with dark-green polyaniline. The product in water phase was filtered, washed with copious amounts of water until the filtrate became colourless, then dried in vacuum at 50 °C for 48 h to obtain the nanofibres of H+-doped polyaniline (PH). The PH nanofibres were immersed in 1 mol/L NH3·H2O for 24 h, then filtered, washed and dried at 80 °C in vacuum for 48 h to prepare the nanofibres of emeraldine base polyaniline (EB).
2.2 Lithium salt doped polyaniline nanofibres
The sample of PH was immersed in 1 mol/L LiPF6/(EC-EMC-DMC) for 72 h in inert atmosphere. After that, the product was filtered, washed with acetone, then dried in vacuum at 60 °C for 15 h to obtain the Li+- doped PH nanofibres, named PHLI. The sample of EB was treated with the same procedure to obtain Li+-doped EB nanofibres, named PLI.
2.3 Preparation of PANI electrode and cell assembly
The electrode was prepared by mixing the PANI powder (80%, mass fraction), carbon black (10%, mass fraction) and PTFE (10%, mass fraction). The mixture was stirred for 5 h at room temperature to obtain a slurry. The slurry was cast on a stainless steel foil using a doctor blade apparatus in a dry room, and dried in a vacuum oven at 50 °C for 20 h. The thickness of the coating layer was 80 μm.
Sandwich-type cells were assembled with two symmetric polyaniline electrodes. The electrodes and separator were soaked in 1 mol/L LiPF6/(EC-EMC-DMC) for redox supercapacitors, respectively. The symmetrical cell was enclosed in a glove box under N2 atmosphere.
2.4 Characterization and electrochemical test
The morphologies of the PANI were observed using a scanning electron microscope (SEM, JSM-5600LV). Fourier transform infrared (FT-IR) spectra were recorded on a FT-IR spectrometer (FT-IR, AVATAR360) in the wavenumber range from 4 000 to 400 cm-1.
All electrochemical tests were performed in the two-electrode system of sandwich-type cells, with one electrode as working electrode and the other electrode as counter electrode and reference electrode. Cyclic voltammogram measurement and AC impedance measurement were performed with Princeton applied research potentiostat (EG&G, 207A) and frequency response analyzer (EG&G, 5210). Constant-current charge-discharge and cycling life measurements were carried out using rapid sampling battery testing system (LAND, CT2001A-5V) at room temperature.
3 Results and discussion
3.1 Morphology and structure
The scanning electron micrographs of PH samples are presented in Fig.1. Highly uniform nanofibres with diameters of 50-100 nm are observed in sample of PH. The lengths of the fibers range from 500 nm up to several micrometers. A closer look at the nanofibres in Fig.1(b) reveals that they are twisted and interconnected. The nanofibrous and porous morphology is thought to be desirable as an electrode material for redox supercapacitors, because it enables effective and rapid access of the electrolyte, which results in the high charge storage and fast charge–discharge processes.
Fig.2 (a) and Fig.2(b) show the SEM images of PHLI and PLI samples, respectively. Very uniform nano-
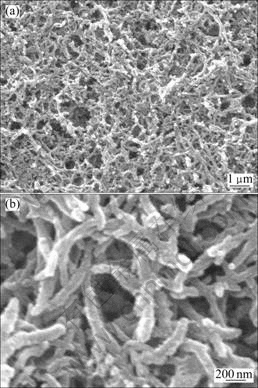
Fig.1 SEM images of PH nanofibres: (a) In low magnification; (b) In high magnification
fibres are also observed, indicating that the morphology of the nanofibres is unaffected when PANI is doped with Li salt.
FT-IR spectra of PH, EB, PHLI and PLI are shown in Fig.3. For EB, the bands at 1 590 cm-1 and 1 496 cm-1 are related to the quinonoid and the benzenoid structures, respectively. The bands at 1 301 cm-1 and 1 164 cm-1 are assigned to the C—N stretching in the quinonoid
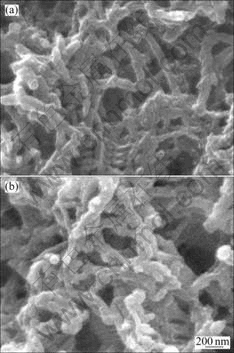
Fig.2 SEM images of PHLI (a) and PLI (b) samples
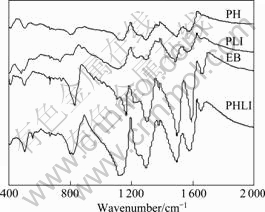
Fig.3 FT-IR spectra of EB, PH, PHLI and PLI samples
benzenoid rings and a mode of QNH+ B imine nitrogen, respectively. It can be seen from Table 1 that almost all peaks in EB red-shift to lower frequency after doping in this experiment. The peak at 1 164 cm-1 in EB red-shifts to 1 139 cm-1 in PH. It can be assigned to the doped PANI with H+ and is commonly observed in the spectra of EB protonated with strong acids [17]. However, the peak at 1 164 cm-1 in EB red-shifts to a little higher frequency in PHLI and PLI, probably because of doping with Li salt instead of doping with the protonic acid HCl.
3.2 Electrochemical performance of PANI in non- aqueous electrolyte
3.2.1 CV curves
To identify the oxidation and reduction potentials and electrochemical reactions, cyclic voltammetry in different potential ranges has been performed for polyaniline doped with LiPF6 capacitor in Fig.4. The oxidation and reduction peaks occur within 0-1 V, and if the potential range is 0-2.5 V, the small capacitance appears in rang of 1-2.5 V and its contribution to the total capacitance becomes negligibly small.
3.2.2 Charge-discharge curves
Fig.5 shows the charge-discharge curves of PH/PH, PHLI/PHLI, and PLI/PLI supercapacitors in 1 mol/L LiPF6/(EC-EMC-DMC). From these curves, it can be seen that in the same situation, the PLI supercapacitor shows the longest discharge time of 60 s and the highest specific capacitance of 120 F/g (47 F/g for PH and
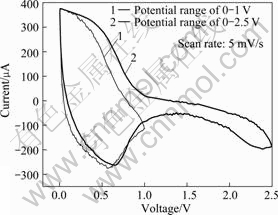
Fig.4 Cyclic voltammogram of PLI non-aqueous capacitor in different potential ranges
Table 1 Positions of vibration of FT-IR for PANI powders

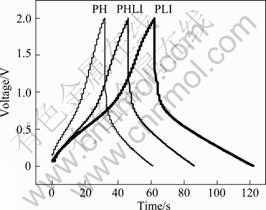
Fig.5 Charge-discharge curves of PH, PHLI and PLI in 1 mol/L LiPF6/(EC-EMC-DMC) non-aqueous electrolyte at 1 mA
66 F/g for PHLI). This indicates that doping by Li salt could improve the electrochemical properties of PANI in organic electrolyte. This result may be due to the fact that PLI sample has more active sites or reaction paths induced from electrochemical reaction by LiPF6 doping.
3.2.3 Impendence spectra
Impedance spectra for PH, PHLI and PLI redox supercapacitors are shown in Fig.6. Note that all spectra are almost similar, which contain a distorted semicircle in the high-frequency region due to the porosity of the electrode and a linear part at the low frequency end due to a diffusion-controlled doping and undoping of anions that result from Warburg behavior [18].
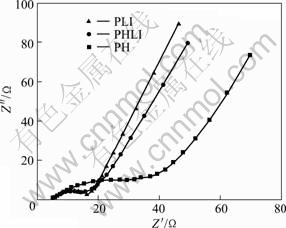
Fig.6 Nyquist plots of PH, PHLI and PLI supercapacitor in 1 mol/L LiPF6/(EC-EMC-DMC) electrolyte at 0 V
The interfacial resistance between the electrodes and the separator of three samples is the same, 3.5 Ω, and the total resistance is 37 Ω for PH capacitor, 27 Ω for PHLI capacitor and 26 Ω for PLI capacitor. The equivalent series resistance (ESR) values are 33.5, 23.5 and 22.5Ω at 1 kHz.
3.2.4 Cyclic life
The cycling results of the cell assembled with PH/PH, PHLI/PHLI and PLI/PLI, which are cycled in voltage range of 0–2 V at 1mA, are presented in Fig.7.
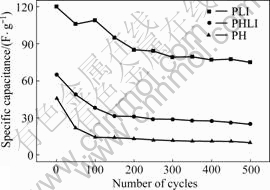
Fig.7 Cycle lives for PH, PHLI and PLI in 1 mol/L LiPF6/(EC-EMC-DMC) non-aqueous electrolyte at 1 mA
The specific capacitance of PLI is 120 F/g on the initial discharging and remains 75 F/g after 500 cycles. The discharge capacitance continuously decreases with the cycling number.
It can be seen that the cycle stability of PH, PHLI supercapacitor is not good after 500 cycles. The loss of capacitance of the PH and PHLI is very serious during 0-500 cycles. The initial specific capacitance is 66 F/g for PHLI and 47 F/g for PH, and it decreases to 25 F/g and 10 F/g. However, the capacitance of PLI/PLI experiences a smooth fading in the first 200 cycles, and the loss of capacitance of PLI/PLI is little during the following 300 cycles. Therefore, PLI is a better electrode material for the redox supercapacitor.
4 Conclusions
1) H+ doped polyaniline nanofibre (PH) was synthesized by interfacial polymerization and polyanilines doped with Li salt (PLI and PHLI) were prepared by immersing emeraldine base (EB) and H+ doped polyaniline in 1 mol/L LiPF6/(EC-EMC-DMC), respectively. With 1 mol/L LiPF6/(EC-EMC-DMC) as electrolyte, PH, PHLI and PLI were used as the active materials of the symmetric non-aqueous redox supercapacitors.
2) The interfacial resistance of PH, PHLI and PLI supercapacitors is the same, 3.5 Ω, and PLI supercapacitor exhibits the lowest total resistance of 26 Ω.
3) PLI shows the highest initial specific capacitance of 120 F/g (10 F/g for PH, 25 F/g for PHLI) among the three samples. After 500 cycles, the specific capacitance of PH and PHLI decreases to 10 F/g and 25 F/g. On the other hand, the specific capacitance of PLI remains 75 F/g after 500 cycles. Therefore, Li-salt doped polyaniline nanofibre is a good candidate material for supercapacitor in non-aqueous electrolyte.
References
[1] CONWAY B E, PELL W G, LIU T C. Diagnostic analyses for mechanisms of self-discharge of electrochemical capacitors and batteries [J]. Journal of Power Sources, 1997, 65(1/2): 53-59.
[2] LAI Yan-qing, LI Jing, SONG Hai-shen, ZHANG Zhi-an, LI Jie, LIU Ye-xiang. Preparation of activated carbons from mesophase pitch and their electrochemical properties [J]. Journal of Central South University of Technology, 2007, 14(5): 601-606.
[3] FRACKOWIAK E, BEGUIN F. Carbon materials for the electrochemical storage of energy in capacitors [J]. Carbon, 2001, 39(6): 937-950.
[4] RYU K S, KIM K M, HONG Y S, PARK Y J, CHANG S H. The polyaniline electrode doped with li salt and protonic acid in lithium secondary battery [J]. Bulletin of the Korean Chemical Society, 2002, 23(8): 1144-1148.
[5] DEARMITT C, ARMES S P. Colloidal dispersions of surfactant- stabilized polypyrrole particles [J]. Langmuir, 1993, 9(3): 652-654.
[6] YANG S M, CHEN K H, YANG Y F. Synthesis of polyaniline nanotubes in the channels of anodic alumina membrane [J]. Synthetic Metals, 2005, 152(1/2/3): 65-68.
[7] SONG Gen-ping HAN Jie, GUO Rong. Synthesis of polyaniline/NiO nanobelts by a self-assembly process [J]. Synthetic Metals, 2007, 157(4/5): 170-175.
[8] SARAVANAN C, PALANIAPPAN S, CHANDEZON F. Synthesis of nanoporous conducting polyaniline using ternary surfactant [J]. Material Letters, 2008, 62(6/7): 882-885.
[9] NEKRASOV A A, GRIBKOVA O L, EREMINA T V, ISAKOVA A A, IVANOV V F, TVERSKOJ V A, VANNIKOV A V. Electrochemical synthesis of polyaniline in the presence of poly(amidosulfonic acid)s with different rigidity of polymer backbone and characterization of the films obtained [J]. Electrochimica Acta, 2008, 53(11): 3789- 3797.
[10] HUANG J X, KANER R B. A general chemical route to polyaniline nanofibers [J]. Journal of the American Chemical Society, 2004, 126(3): 851-855.
[11] ZHANG X Y, ROCH C Y, JOSE A, MANOHAR S K. Nanofibers of polyaniline synthesized by interfacial polymerization [J]. Synthetic Metals, 2004, 145(1): 23-29.
[12] VINAY G, NORIO M. High performance electrochemical supercapacitor from electrochemically synthesized nanostructured polyaniline [J]. Materials Letters, 2006, 60(12): 1466-1469.
[13] ZHAO Guang-yu, LI Hu-Lin. Preparation of polyaniline nanowire arrayed electrodes for electrochemical supercapacitors [J]. Microporous and Mesoporous Materials, 2008, 110(2/3): 590-594.
[14] RYU K S, KIM K M. A hybrid power source with a shared electrode of polyaniline doped with LiPF6 [J]. Journal of Power Sources, 2007, 165(1): 420-426.
[15] RYU K S, LEEB Y, HANC K S, PARK Y J, KANG M G, PARK N G, CHANG S H. Electrochemical supercapacitor based on polyaniline doped with lithium salt and active carbon electrodes [J]. Solid State Ionics, 2004, 175(1/4): 765-768.
[16] RYU K S, KIM K M, PARK Y J, PARK N G, KANG M G, CHANG S H. Redox supercapacitor using polyaniline doped with Li salt as electrode [J]. Solid State Ionics, 2002, 152/153: 861-866.
[17] DRELIKIEWICZ A, HASIK M, CHOCZYNSKI M, The new oxygen-deficient fluorite Bi3NbO7: Synthesis, electrical behavior and structural approach [J]. Materials Research Bulletin, 1998, 33(1): 739-762.
[18] ZHENG J P, HUANG J, JOW T R. The limitations of energy density for electrochemical capacitors [J]. Journal of Electrochemical Society, 1997, 144(6): 2026-2031.
(Edited by YANG Bing)
Foundation item: Project(2008AA03Z207) supported by the National Hi-tech Research and Development Program of China
Received date: 2010-01-20; Accepted date: 2010-04-14
Corresponding author: LAI Yan-qing, Professor, PhD; Tel: +86-731-88876454; E-mail: 13975808172@126.com