
Interfacial characteristics and dynamic mechanical properties of Wf/Zr-based metallic glass matrix composites
MA Wei-feng(马卫锋), KOU Hong-chao(寇宏超), CHEN Chun-sheng(陈春生), LI Jin-shan(李金山),
HU Rui(胡 锐), XING Li-qian(刑力谦), ZHOU Lian(周 廉), FU Heng-zhi(傅恒志)
State Key Laboratory of Solidification Processing, Northwestern Polytechnical University,Xi’an 710072, China
Received 6 March 2007; accepted 23 August 2007
Abstract: Tungsten fiber reinforced Zr41.25Ti13.75Cu12.5Ni10Be22.5 metallic glass matrix composites were fabricated by means of melt infiltration casting. Their dynamic compressive tests were performed using a Hopkinson bar. The relationship between the interfacial characteristics and the dynamic compressive behavior was investigated. The results indicate that the interface characteristics of composites include interfacial diffusion and interfacial reaction, and the interfacial shear strength increases when the interfacial reaction is serious. The dynamic plastic performance are improved obviously if the suitable interface reaction occurs. The failure occurs by shear and the fibers split longitudinally if there is no interface reaction or a little reaction; in contrast, holistic failure occurs if there is too much interface reaction.
Key words: metallic glasses; push-out method; dynamic mechanical properties; interface reaction
1 Introduction
Bulk metallic glasses(BMGs) have excellent mechanical properties such as high yield strength and unique fracture toughness[1-4]. A plane strain fracture toughness higher up to 20-55 MPa?m1/2 has been reported in the Zr41.25Ti13.75Cu12.5Ni10Be22.5 bulk metallic glass[5]. However, most BMGs are prone to catastrophic failure upon yielding in tension or compression test at room temperature, which severely limits their use in structural applications. In order to overcome this drawback, two different approaches have been put forward for improving the toughness. One is to introduce foreign particles or fibers such as SiC, WC, Ta, Mo, and W into the metallic glass matrix[6-9]. The other is to make the metallic glass grow into in-situ composite which is achieved by partially crystallizing with cooling or subsequent heating. Previous work showed that the reinforcements by any one approach above indeed restrict the propagation of shear bands and promote the generation of multiple shear bands and additional fracture surface area. The BMGs matrix composites exhibited significantly improved plastic strain and dynamic deformation in comparison with BMG alloys [10-11].
Furthermore, the quality of BMGs matrix composite is not only defined by the properties of the matrix and the reinforcement material but also by the controlling of infiltration conditions, such as infiltration temperature, pressure and infiltration time. In fact, these parameters are essentially affected by the wetability and interface reaction of the metallic melt with the fibers. SCHROERS[12] investigated the reaction of the bulk glass forming alloy Zr41Ti14Cu12Ni10Be23 (Vit 1) with W substrate by using sessile drop technology. They found that there are good wetability and interface reaction between W and metallic glass matrix. It is well known that the structure and properties of interface determine the mechanical properties of composite. However, no relevant research has been reported to date. In the present work, the Wf/Zr-based metallic glass matrix composites were prepared by melt infiltration casting at different infiltration temperature and same infiltration time. The relationship between the interfacial characteristic and the dynamic compressive behavior was investigated.
2 Experimental
2.1 Samples preparation
Ingots of Zr41.25Ti13.75Cu12.5Ni10Be22.5 were prepared by arc-melting the constituents with a purity ranging from 99.5% to 99.99% in a titanium-gettered argon atmosphere. Tungsten fibers of 250 μm in diameter were straightened and cut to 50 mm in length. The tungsten fibers were cleaned in an ultrasonic bath of acetone, followed by ethanol after dipping in hydrofluoric acid for 12 h to remove surface impurity. Composite samples of 6 mm in diameter and 50 mm in length were fabricated at the same infiltration time but different infiltration temperatures. The details of the casting may be found in Ref.[13].
The parameters of melt infiltration casting used in the current experiments are listed in Table 1. The castings were first centerless ground to a 6 mm nominal diameter, then cut to length of about 6 mm for dynamic compression samples. Dynamic compression tests were performed using the Split Hopkinson Pressure Bar (SHPB) at strain rates of 102-103 s-1. In order to protect the end of the bars and limit the final strain of the specimen, some modifications such as steel inserts and lantern ring were used in dynamic compression tests.
Table 1 Melting point of matrix alloy and parameters of melt infiltration casting

2.2 Properties characterization
X-ray diffraction patterns of a cross sectional slice of each composite were taken using a PHILIPS APD-10 diffractometer with Cu Kα radiation. The interface reaction, diffusion and the fracture surface were analyzed by using LEO1450 scanning electron microscope(SEM). The interfacial shear strength τd of the composites was determined by the push-out method. Slices approximately 100 μm in height were cut from the as-cast rod. The schematic diagram of the push-out method is shown in Fig.1. Note that the value of τd is average one given by the principle as
τd =Pmax/(2πRfL) (1)
where Pmax is the maximum load, Rf is the radius of the fiber and L is the length of the interaction zone[14-15].
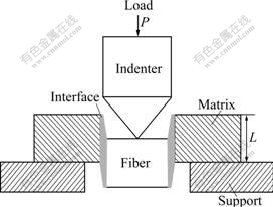
Fig.1 Schematic diagram of push-out method for measuring interfacial shear strength of composites
3 Results and discussion
3.1 Interfacial characteristics
Fig.2 shows the X-ray diffraction patterns of the composites reinforced with 60% tungsten. The patterns of an unreinforced sample of the matrix material and the tungsten are included for comparison. The composite shows diffraction peaks from tungsten fiber superimposed on the broad diffusion scattering maxima of the amorphous matrix phase. No other phases are detected within the sensitivity limit of X-ray diffraction. The microstructure of the tungsten is not affected during processing because of its high melting temperature. The diffraction patterns suggest that the Zr41.25Ti13.75Cu12.5- Ni10Be22.5 matrix is amorphous after processing and quenching.
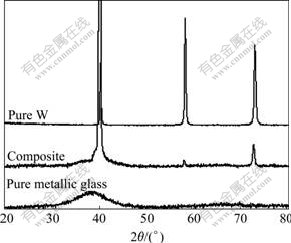
Fig.2 XRD patterns of cross-sectioned uniaxially reinforced bulk metallic glass composite processed at infiltration temperature of 984 K (Pattern of unreinforced sample of matrix material and tungsten are included for comparison)
Fig.3 shows the SEM micrographs of the composites that are processed at the same infiltration time but different infiltration temperatures. In these photographs, the lighter regions are W fiber, the darker regions are matrix, and the gray regions are interfacial reaction layers. It can be seen that the infiltration temperature has obvious effect on the interface of W fiber and metallic glass matrix. Small crystals formed adjacent to the fiber reinforcement are detected. The interface reaction between W fibers and matrix becomes more serious and the crystalline phase within the matrix increases with increasing the infiltration temperature from 984 K to 1 084 K. The interfacial reaction product is identified as W5Zr3 phase by electron probe analysis, which could not be detected by X-ray diffraction due to the small fraction.
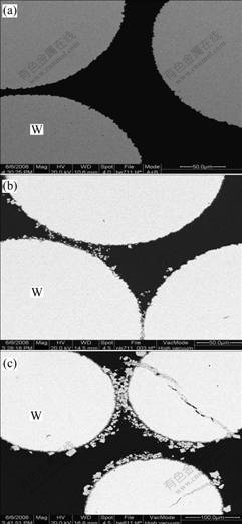
Fig.3 SEM photographs of W fiber reinforced Zr41.25Ti13.75- Cu12.5Ni10Be22.5 composites processed at same infiltration time of 15 min and different infiltration temperatures of 984 K (a), 1 034 K (b) and 1 084 K (c)
Fig.4 shows the line scanning across interface of W fiber reinforced Zr41.25Ti13.75Cu12.5Ni10Be22.5 bulk metallic glass matrix composites. The line scanning suggests that the interface of composites has a diffusion-dissolution layer. There exists not only solid phase diffusion from the matrix alloy to tungsten fibers but also solution-diffusion from the solid phase W fiber to the matrix alloy. The reaction layer has a thickness of about 3 μm. The interface is actually the result of both interface diffusion and interface reaction. The interfacial bilateral diffusion is the prerequisite of the interface reaction[16]. The interface diffusion mainly occurs below 1 034 K, and thus the interface reaction appears above 1 034 K.
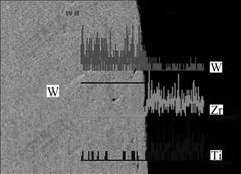
Fig.4 EPMA line scanning across interface of W fiber reinforced Zr41.25Ti13.75Cu12.5Ni10Be22.5 composites processed at infiltration temperature of 984 K and infiltration time of 15 min
The push-out tests were performed on tungsten fiber reinforced Zr41.25Ti13.75Cu12.5Ni10Be22.5 bulk metallic glass matrix composites at different infiltration temperatures (Fig.5). The applied load increases when the interface reaction is serious. The values of τd of the composites are 84, 142 and 235MPa respectively. At the same time, the different stages are involved in a typical push-out test. When a compressive load is applied to the top face of the W fiber, shear stresses are introduced at the interface with maximum value occurring at the region near the top face. When the applied load reaches Pa (choose Ti=1 034 K as typical curve), the shear stress in this region reaches the critical value, and debonding initiates, causing a change in slope in Fig.5. The shear stress in the debonded zone drops and the region of maximum shear stress moves away from the top face when the applied load is increased. The debonding progression, where P represents load between Pa and Pb, when the load reaches Pb, the maximum shear stress reaches the critical value at the bottom face. As a result the entire length of the W fiber gets debonded, and the fiber is pushed out of the matrix. This result in a sudden drop in the load from Pb to Pc because of the resistance to further movement of the fiber is mainly due to friction and surface roughness. Finally, a load increase takes place at the end of the push-out experiment when the indenter contacts the matrix surrounding the pushed-out fiber.
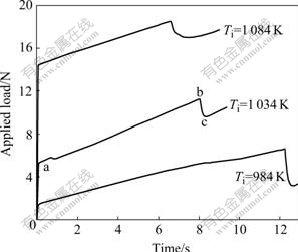
Fig.5 Curves of push-out applied load vs time of W fiber reinforced Zr41.25Ti13.75Cu12.5Ni10Be22.5 composites processed at different infiltration temperatures
3.2 Dynamic mechanical properties
Dynamic compressive tests were performed on W fiber reinforced bulk metallic glass matrix composites processed at infiltration temperatures of 984 K (a), 1 034 K (b) and 1 084 K (c) respectively (Fig.6). At least three samples of each infiltration temperature were tested. It is easily seen that infiltration temperature has obvious effect on dynamic mechanical properties. The dynamic compressive strength of the composites is about 2 660 MPa which has no obvious change with 60% (volume fraction) W fiber reinforced. The material exhibits elastic perfectly plastic deformation at strain rate of 102-103 s-1, with strains up to 14%. The dynamic compressive stress—strain curves of composites show a change in hollowness, which indicates that the yielding occurs. The plastic deformation of composites occurs after the yielding. The stress—strain curves have a flat stress region broadly, then the curves will attenuate rapidly. The dynamic compressive properties of composites reach the optimal value when the infiltration temperature increases to 1 034 K.
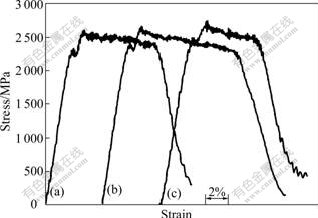
Fig.6 Dynamic compressive stress—strain curves for 60% W fiber reinforced Zr41.25Ti13.75Cu12.5Ni10Be22.5 composites processed at same infiltration time of 15 min and different infiltration temperatures of 984 K (a), 1 034 K (b) and 1 084 K (c)
The dynamic compressive fracture of composite with 60% tungsten is illustrated in Fig.7. Fig.7(a) shows a fracture surface of no interface reaction composite that fails in shear and the fibers split longitudinally. This fracture mode suggests that the fibers first yield, which makes the fibers unstable and unable to prevent buckling in the composite. The fibers split longitudinally and the samples failed in shear occur at the same time. Fig.7(b) shows a fracture surface of a little interface reaction composite that fails in split longitudinally and localizes shear. The shear fracture surface is like a slurry flow in the inset image that is the area around the fiber at higher magnification, and it appears that the temperature in the shear band becomes high enough to cause the matrix to show viscous flow. The pattern left by the matrix material that spreads over the fiber indicates that shear band travels from bottom to top. When the fiber and the matrix have severe interface reaction, the samples fail in holistic split and break into pieces (Fig.7(c)). This fracture mode suggests that severe interface reaction cause the interface strength too high to transfer load effectively. So that, the W fiber cannot support the compressive load and arrest crack propagation. From the observation above, it can be concluded that the failure mode of composites shifts form shear and the fibers split longitudinally to holistic fail with the interfacial shear strength increasing.
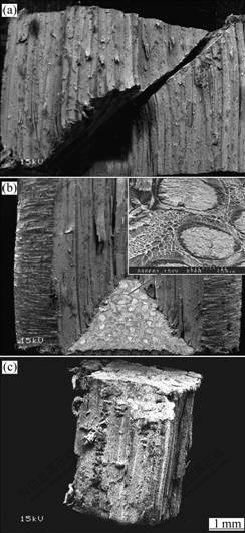
Fig.7 SEM photographs of dynamic compressive fracture surface of 60%W fiber reinforced Zr41.25Ti13.75Cu12.5Ni10Be22.5 composites processed at same infiltration time of 15 min and different infiltration temperatures of 984 K (a), 1 034 K (b) and 1 084 K (c)
From the experimental results above, it can be concluded that the interfacial bond of metallic glass matrix composites has close relation with the dynamic compressive deformation behavior. Because the infiltration temperature is above the melting temperature of matrix alloy, the interfacial reactions between W fiber and matrix often unavoidably occur. The interface is simply a diffusion-dissolution layer only and there is no interfacial reaction between W fibers and matrix at low infiltration temperature, but the interface between W fibers and matrix exhibits bond flimsily and cannot transfer load effectively. It is proved that the suitable interface reaction is necessary and favourable to the bond of W fiber and matrix. But the excessive interface reaction is bad to the composite, and there isn’t propagation of the cracks along the interface if the interfacial bond is very strong. In this case the plastic deformation of composite tends to trail off and fails in holistic mode.
4 Conclusions
1) The interface reaction of W fiber reinforced Zr41.25Ti13.75Cu12.5Ni10Be22.5 composites processed at the same infiltration time and different infiltration temperatures were analyzed. With increasing the infiltration temperature, the interface reaction between W fibers and matrix gradually becomes more serious. The interfacial reaction process consists of both diffusion and chemical reaction. The interface strength increases when the degree of interface reaction is more serious. The dynamic compressive properties of composites reach the optimal value when the infiltration temperature increases to 1 034 K.
2) The dynamic plastic performance are improved obviously if the suitable interface reaction occurs, with strains up to 14% at strain rates of 102-103 s-1. The failure mode shifts from shear and the fibers split longitudinally with no interface reaction or a little reaction to holistic fail with too much interface reaction.
References
[1] Inoue A. Stabilization of metallic supercooled liquid and bulk amorphous alloys [J]. Acta Mater, 2000, 48: 279-306.
[2] Wang W H, Dong C, Shek C H. Bulk metallic glasses [J]. Mater Sci Eng R, 2004, 44: 45-89.
[3] Inoue A, Takeuchi A. Recent progress in bulk glassy, nanoquasicrystalline and nanocrystalline alloys [J]. Mater Sci Eng A, 2004, 375/377: 16-30.
[4] Schuh c a, Hufnagel t c, RAMAMURTY U. Mechanical behavior of amorphous alloys [J]. Acta Mater, 2006, 2007, 55: 4067- 4109.
[5] Peker A, Johnson W L. A highly processable metallic glass: Zr41.2Ti13.8Cu12.5Ni10.0Be22.5 [J]. Appl Phys Lett, 1993, 63(17): 2342-2344.
[6] Choi-Yim H, Conner R D, FRIGYYES S. Quasistatic and dynamic deformation of tungsten reinforced Zr57Nb5Al10Cu15.4Ni12.6 bulk metallic glass matrix composites [J]. Scripta Mater, 2001, 45: 1039-1045.
[7] Wang G, Chen D M, Shen J, Stachurski Z H, Qin Q H, Sun J F, Zhou B D. Deformation behaviors of a tungsten-wire/bulk metallic glass matrix composite in a wide strain rate range [J]. J Non-Cryst Solids, 2006(35): 3872-3878.
[8] Tan h, Zhang Y, Li Y. Synthesis of La-based in-situ bulk metallic glass matrix composites [J]. Intermetallics, 2002, 10: 1203-1205.
[9] Zhang H, Zhang Z F, Wang Z G, Qiu K Q, Zhang H F, Zang Q S, Hu Z Q. Fatigue damage and fracture behavior of tungsten fiber reinforced Zr-based metallic glassy composite [J]. Mater Sci Eng A, 2006, 418: 146-154.
[10] Qiu K Q, Wang A M, Zhang H F, Ding B Z, Hu Z Q. Mechanical properties of tungsten fiber reinforced ZrAlNiCuSi metallic glass matrix composite [J]. Intermetallics, 2002, 10: 1283-1288.
[11] Zhang H, Zhang Z F, Wang Z G, Qiu K Q, Zhang H F, Zang Q S. Effects of tungsten fiber on failure mode of Zr-based bulk metallic glassy composite [J]. Metall Mater Trans A, 2006, 37A: 2459-2469.
[12] Schroes J. Characterization of interface between the bulk glass forming alloy ZrTiCuNiBe with pure metals and ceramics [J]. J Mater Res, 2000, 15(7): 1617-1621.
[13] Dandlike R B, Conner R D, Johnson W L. Melt infiltration casting of bulk metallic glass matrix [J]. J Mater Res, 1998, 13(10): 2898-2901.
[14] Chandra N, Ghonem H. interfacial mechanics of push-out tests: Theory and experiments [J]. Composites Part A, 2001, 32: 575-584.
[15] Dinter J, Peters P W M, Hemptenmacher J. Finite element modelling of the push-out test for fiber-reinforced titanium alloys [J]. Composites Part A, 1996, 27: 749-753.
[16] Zhang G D. Metallic matrix composites [M]. Shanghai: Shanghai Jiaotong University Press, 1996. (in Chinese)
Foundation item: Project(2005K06-G2) supported by the New Century Excellent Person Supporting Project of Ministry of Education of China
Corresponding author: MA Wei-feng; Tel.: +86-29-88493484; Fax: +86-29-88480294; E-mail: weifeng1016@yahoo.com.cn
(Edited by LI Xiang-qun)