
Fabrication of polypyrrole/TiO2 nanocomposite via
electrochemical process and its photoconductivity
OUYANG Mi(欧阳密)1, 2, 3, BAI Ru(白 茹)3, 4, XU Yi(徐 意)1, ZHANG Cheng(张 诚)1,
MA Chun-an(马淳安)1, WANG Mang(汪 茫)3, CHEN Hong-zheng(陈红征)3
1. State Key Laboratory of Breeding Base of Green Chemistry-Synthesis Technology,
Zhejiang University of Technology, Hangzhou 310032, China;
2. College of Chemical Engineering and Materials Science, Zhejiang University of Technology,
Hangzhou 310032, China;
3. Department of Polymer Science and Engineering, State Key Laboratory of Silicon Materials,
Zhejiang University, Hangzhou 310029, China;
4. School of Electronic Information, Hangzhou Dianzi University, Hangzhou 310037, China
Received 10 August 2009; accepted 15 September 2009
Abstract: Size-controllable and vertically-oriented TiO2 nanotube (TNT) arrays were fabricated using anodic oxidation method from pure titanium sheets in electrolyte solutions. Then with the TNT arrays as the working electrode, a thin film of polypyrrole (Ppy) was synthesized into the TNT arrays via the electrochemical polymerization. During the process, the appearance of redox peaks in cyclic voltammetry curves of the resulting films indicates the occurrence of polymerization. The morphology, the molecular structure, crystallization and optical properties of the TNT arrays and the resulting polymer were investigated by field-emission scanning electron microscopy (FESEM), X-ray diffractometry (XRD), Fourier transform infrared spectroscopy (FTIR), and UV-vis spectrum analysis, respectively. A dual-layered photoreceptor containing the nanocomposite film as the charge generation layer (CGL) was designed and fabricated. It is found that the photoreceptor based on Ppy/TiO2 nanotubes as CGL exhibits remarkable photoconductive performance.
Key words: polypyrrole; titania; nanocomposite; electrochemical polymerization; photoconductivity
1 Introduction
The research on the organic optoelectronic materials, especially polymer semiconductors, has aroused increasing attention, and great progress has been made [1-2]. The properties of these organic semiconducting materials, such as photoconductivities, photovoltaic characteristics, and photogating abilities, are particularly of great interest[3]. Compared with inorganic semiconductor materials, polymeric optoelectronic materials possess unique advantages of low-cost, easy processing, flexible substrates, etc. Furthermore, the energy band structure, as well as the optoelectronic properties of conjugated polymers can be fine-tuned via modifying their molecular structure[4] or varying their aggregate states, such as different nanostructures. Thus, as a series of functional materials, conjugated polymeric optoelectronic materials have been widely used in the fields of polymer solar cells (PSCs)[5], electrochromic devices[6], sensors[7], polymer light emitting diodes (PLEDs)[8], and so on.
Generally, conjugated polymers are synthesized by either chemical or electrochemical polymerization. Although chemical routes are easy to get products in large scale, the obtained conjugated polymers are usually insoluble powders, which are difficult to be processed during the film coating in devices. Compared with the chemical routes, electrochemical polymerization can obtain conjugated polymer films on conductive substrates directly, which not only enlarges the scope of candidate polymers[9], but also simplifies the procedure of the film coating[10]. Meanwhile, the introduction of nanotechnology improves the performance of materials significantly[11]. Using template-assistant-synthesis method, a variety of organic or organic/inorganic nanowires or nanotubes have been successfully fabricated[12-14], which showed better performance than their bulk counterparts. Therefore, a scientific study on fabrication of polymer/inorganic nanoarray composites is of great interest, and has become one of the focuses in polymer optoelectronics field.
With these considerations, we reported the fabrication of nanocomposite film of polypyrrole (Ppy) and TiO2 nanotube (TNT) arrays via electrochemical methods. A novel dual-layered photoreceptor based on the nanocomposite film as charge generation layer (CGL) was designed and fabricated.
2 Experimental
TiO2 nanotubes were prepared by using an electrochemical anodization method similar to that described previously[15]. Briefly, the cleaned titanium foils (0.2 mm in thickness, 99.9% in purity, purchased from Hongtai Rare Metal Inc., Shanghai, China) were anodized in the electrolyte consisting of 0.5% HF and acetic acid mixed in 7?1 of volume ratio using a platinum counter-electrode (99.9% purity, Alfa-Aesar Corporation). The resulting TiO2 samples were annealed at temperatures ranging from 28 ℃ to 580 ℃ in oxygen ambient for 6 h with heating and cooling rates of 2 ℃/min.
Electrosyntheses and measurements were performed in a three-compartment cell with a CHI 660 C electrochemical analyzer. The working electrode for cyclic voltammetry (CV) experiments was a TNT arrays or ITO glass with width of 0.8 cm and length of 1.5 cm. A platinum flake was used as a counter electrode and an Ag/AgCl (saturated KCl) was used as a quasi-reference electrode. All of the electrochemical experiments were performed under N2 atmosphere at 20 ℃. Pyrrole (Acros Organics, 99%) was electrochemically polymerized on the surface of ITO or TNT arrays with 0.1 mol/L tetrabutylammonium perchlorate (TBAP, Acros Organics, 95%)/acetonitrile (ACN, Shanghai Chemical Reagent Company, China) as a supporting electrolyte. The concentration of pyrrole in electrolytes used for polymerization was 25 mmol/L. For the further characterization and analysis, the resulting films were dried under vacuum at 80 ℃ for 24 h.
The morphologies of samples were studied by using a field-emission scanning electron microscope (FESEM, Hitachi S-4700). The crystal structure of films was detected by X-ray diffraction (XRD) on a D/Max-2550PC diffractometer (Rigaku, Japan). Infrared spectra were recorded using Bruker Vertex 70 FTIR spectrometer with KBr pellets. UV-vis spectra were performed on a Varian Cary 100 UV-vis spectrophotometer.
A simple dual-layered photoreceptor was prepared as follows. The composite films served as the CGL, and then polycarbonate matrix containing 50% phenylhydrazone (PH) was coated on the surface of CGL as a charge transportation layer (CTL). The photosensitivity measurement was carried out on a GDT-II model photoconductivity measuring device by a standard photoinduced discharge technique[16], from which several parameters for photoreceptors were derived: φo, Rd, and φr, defined as initial surface charge potential, dark decay, and residual surface charge potential, respectively. The photosensitivity was characterized by photosensitivity (S) which is defined as the reciprocal of the product of the half decaying time (t1/2) and the intensity of exposure light (I), that is, S = [I?t1/2]-1. The bigger the S value is, the higher the photosensitivity of the photoreceptor is.
3 Results and discussion
3.1 Fabrication of TiO2 nanotube arrays
Fig.1 presents the FESEM images of as-prepared TNT arrays anodized at 18 V for 30 min. The diameter and length of the TiO2 nanotubes were found from Figs.1(a) and (b) as 80 nm and 300 nm, respectively. Moreover, it was found that the nanotube pore size was proportional to the applied potential and independent of the anodization time. The XRD patterns of TiO2 nano- tubes annealed at different temperatures up to 580 ℃ are given in Fig.2. The as-prepared nanotubes were found to be amorphous (only reflections from titanium substrate can be seen). The anatase phase appeared at a temperature near 280 ℃. With increasing the annealing temperature further to 580 ℃, rutile phase obtained predominance as evident from the higher intensity ratio of rutile peak to anatase peak.
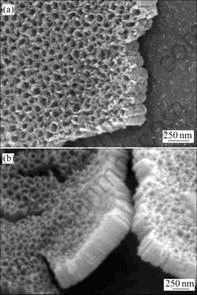
Fig.1 FESEM images of as-prepared TiO2 nanotube arrays: (a) Top view; (b) Cross-sectional image
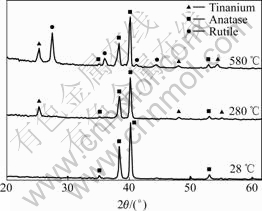
Fig.2 XRD patterns of TiO2 nanotube arrays annealed at temperatures ranging from 28 to 580 ℃ in air for 6 h
3.2 Characterization of polypyrrole films
The polarization curve of pyrrole in 0.1 mol/L TBAP/ACN is shown in Fig.3(a). The onset potential for anodic current was 1.0 V. The successive CV curves of pyrrole in 0.1 mol/L TBAP/ACN between -0.8 and 1.3 V are illustrated in Fig.3(b). The oxidation current increases with increasing scanning cycle, and the observable polymer films can be formed on the working electrode for the systems. The polymerization of pyrrole presents an oxidation peak potential at 0.8 V and a reduction peak at 0.2 V (see Fig.3(b)), indicating the formation of a new polymer synthesized by electrochemical polymerization.
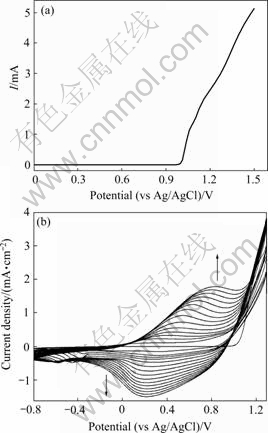
Fig.3 Polarization curve (a) and successive CV curves (b) of 25 mmol/L pyrrole in 0.1 mol/L TBAP/ACN (Scanning rate: 5 mV/s and 50 mV/s, respectively)
Fig.4 shows the FT-IR and UV-vis spectra of polypyrrole, which was prepared on the surface of ITO. According to Fig.4(a), a skeletal vibration of pyrrole ring appeared at 1 544 cm-1. The bands at 1 090 and 1 037 cm-1 were assigned to the in-plane deformation vibration of pyrrole ring; and the bands at 3 450 and 1 173 cm-1 were originated from the stretching modes of N—H and C—C in the pyrrole ring, respectively[17]. The appearance of redox peaks in successive CV curves of polymer (see Fig.3(b)) and the features from Fig.4(a) confirm that the obtained polymer is polypyrrole. UV-vis spectrum of polypyrrole is shown in Fig.4(b). Ppy presents the wide and strong absorption peaks in 600- 800 nm and another obviously absorption peak in 340- 500 nm, respectively. The broad absorption of the Ppy in the visible and infrared region makes them ideal candidates for photovoltaic application.
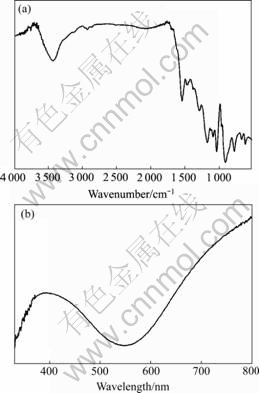
Fig.4 FT-IR spectrum (a) and UV-vis spectrum (b) of Ppy film synthesized from 0.1 mol/L TBAP/ACN on ITO surface
XRD patterns of Ppy film were recorded to investigate the crystal structure of the as-prepared Ppy. Fig.5 shows the XRD patterns recorded for the Ppy electrochemically polymerized on the surface of ITO and for blank ITO substrate without polymer film. The diffraction peaks at 2θ=8? was observed for Ppy/ITO film, indicating a crystal structure with low crystallinity for Ppy film. The observations reveal that Ppy film is polycrystalline.
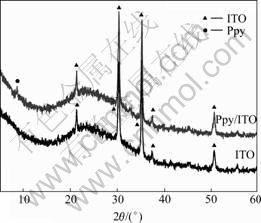
Fig.5 XRD patterns of Ppy synthesized on ITO surface
3.3 Photoconductivity of Ppy/TNT arrays nano- composite films
With the TiO2 nanotube arrays as the working electrode, a thin film of Ppy was synthesized into the TiO2 nanotubes via the electrochemical polymerization. Fig.6 presents the FESEM images of as-prepared nanocomposite film of Ppy and TiO2 nanotube arrays at various CV cycles. By comparing with the pure TiO2 nanotube arrays (Fig.1(a)), the successful polymerization of Ppy on the TiO2 nanotube arrays was confirmed by the obvious deposition layer. The average thickness of the resulting polymer film increased obviously with increasing the CV cycles from 12 to 48.
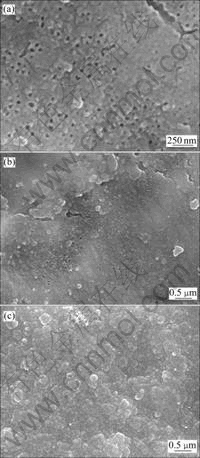
Fig.6 Morphologies of Ppy/TiO2 nanotubes composite films at various CV cycles: (a) 12; (b) 24; (c) 48
A dual-layered photoreceptor containing the above nanocomposite as the charge generation layer were designed and fabricated (see Fig.7(a)). Its photo- conductivities under illumination of visible light were investigated using photoinduced xerographic discharge technique (see Fig.7(b)). The photoconductivity of the photoreceptors based on the Ppy/TiO2 nanofilm was measured by exposing to a visible light with an intensity of 1.1 mW/cm2 from a halogen lamp. The results are summarized in Table 1.
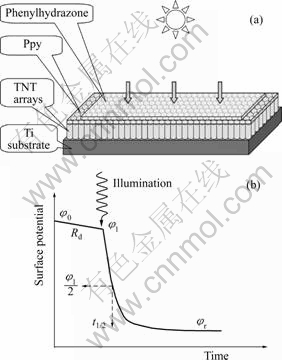
Fig.7 Configuration of double-layered photoreceptor with Ppy/TiO2 as CGL, phenylhydrazone as CTL, and Ti conductive substrate(a) and typical photoinduced xerographic discharge curve(b)
Table 1 Photoconductivities of dual-layered photoreceptors based on Ppy/TNT arrays nanocomposite film and TNT arrays
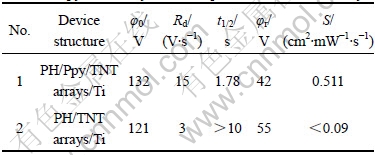
It was found from Table 1 that the photoreceptor from Ppy/TiO2 nanocomposite film could be readily negatively charged to a surface potential of 132 V (φ0), and gave a low dark decay (Rd) of 15 V/s (Table 1, No.1). Upon exposure to light, the half-discharge time (t1/2) was 1.78 s, the residual potential (φr) on the surface of the photoreceptor was 42 V, and the photosensitivity (S) was 0.511 cm2/(mW?s). For the purpose of comparison, the photosensitivity of the photoreceptor based on the TNT arrays was also measured (Table 1, No.2), and the results demonstrated that the photosensitivity of the photoreceptor based on the Ppy/TiO2 nanocomposite film was enhanced by over 5.7 times compared with that from TiO2 nanotube arrays.
The reason why Ppy/TNT arrays exhibited a higher photosensitivity than the single component of TiO2 nanotubes was thought to be related to the larger contact area between TiO2 nanotues and Ppy, as well as the one dimensional alignment of TiO2 nanotubes. The large contact area between TiO2 and Ppy facilitated the photoinduced charge transfer from Ppy to TiO2, which together with the directional alignment of TiO2 nanotubes was favorable to the following charge separation and transport processes. Photoreceptors from donor-acceptor nanocomposites with high photo- sensitivities owing to the photoinduced charge transfer between the donor and the acceptor were also reported in Refs.[16, 18].
4 Conclusions
1) TiO2 nanotube arrays were prepared in fluoride containing acidic electrolytes. The nanotubes with desired sizes and crystallization could be achieved by altering anodization conditions and annealing temperature.
2) With the electrochemical method, a series of Ppy films were successfully synthesized in 0.1 mol/L TBAP/ACN solution via cyclic voltammetry. The average thickness of the resulting polymer film increased obviously with the increase of the CV cycles.
3) A dual-layered photoreceptor based on Ppy/TiO2 nanotube arrays was designed and fabricated. The excellent photoconductivity of the photoreceptor based on Ppy/TiO2 nanotubes was ascribed to the large donor- acceptor contact area and the directional alignment of TiO2 nanotubes, which enhanced the photoinduced charge transfer efficiency between Ppy and TiO2 nanotubes.
References
[1] KIM J Y, KIM S H, LEE H H, LEE K, MA W, GONG X, HEEGER A J. New architecture for high-efficiency polymer photovoltaic cells using solution-based titanium oxide as an optical spacer [J]. Adv Mater, 2006, 18: 572-576.
[2] PAN H, LI Y, WU Y, LIU P, ONG B S, ZHU S, XU G. Low-temperature, solution-processed, high-mobility polymer semiconductors for thin-film transistors [J]. J Am Chem Soc, 2007, 129: 4112-4113.
[3] HUYNH W U, DITTMER J J, ALIVISATOS A P. Hybrid nanorod-polymer solar cells [J]. Science, 2002, 295: 2425-2427.
[4] YAMAMOTO T, KOIZUMI T. Synthesis of pi-conjugated polymers bearing electronic and optical functionalities by organometallic polycondensations and their chemical properties [J]. Polymer, 2007, 48: 5449-5472.
[5] KIM J Y, LEE K, COATES N E, MOSES D, NGUYEN T Q, DANTE M, HEEGER A J. Efficient tandem polymer solar cells fabricated by all-solution processing [J]. Science, 2007, 317: 222-225.
[6] YILDIRIM A, TARKUC S, AK M, TOPPARE L. Syntheses of electroactive layers based on functionalized anthracene for electrochromic applications [J]. Electrochim Acta, 2008, 53: 4875-4882.
[7] CHEN J, TOO C, WALLACE G, BURRELL A, COLLIS G, OFFICER D, SWEIGERS G. Preparation, characterisation and biosensor application of conducting polymers based on ferrocene substituted thiophene and terthiophene [J]. Electrochim Acta, 2002, 47: 2715-2724.
[8] FRIEND R H, GYMER R W, HOLMES A B, BURROUGHES J H, MARKS R N, TALIANI C, BRADLEY D D C, DOS SANTOS D A, BR?DAS J L, L?GDLUND M, SALANECK W R. Electro- luminescence in conjugated polymers [J]. Nature, 1999, 397: 121-128.
[9] CHANG C C, HER L J, HONG J L. Copolymer from electro- polymerization of thiophene and 3,4-ethylenedioxythiophene and its use as cathode for lithium ion battery [J]. Electrochim Acta, 2005, 50: 4461-4468.
[10] LI M, TANG S, SHEN F, LIU M, XIE W, XIA H, LIU L, TIAN L, XIE Z, LU P, HANIF M, LU D, CHENG G, MA Y. Highly luminescent network films from electrochemical deposition of peripheral carbazole functionalized fluorene oligomer and their applications for light-emitting diodes [J]. Chem Commun, 2006, 32: 3393-3395.
[11] BAI R, OUYANG M, LI Z, SHI M, WU G, WANG M, CHEN H Z. A facile nanotemplate preparation method for [60]fullerene nanowires: Surface-wetting [J]. Journal of Materials Chemsitry, 2008, 18: 4318-4323.
[12] XIAO R, CHO S, LIU R, LEE S B. Controlled electrochemical synthesis of conductive polymer nanotube structures [J]. J Am Chem Soc, 2007, 129: 4483-4489.
[13] OUYANG M, BAI R, CHEN L, YANG L G, WANG M, CHEN H Z. High photoconductive copper phthalocyanine-coated titania nanoarrays via secondary deposition [J]. J Phys Chem C, 2008, 112: 11250-11256.
[14] BAI R, OUYANG M, ZHOU R J, SHI M M, WANG M, CHEN H Z. Well-defined nanoarrays from N-type organic perylene-diimide derivative for photoconductive devices [J]. Nanotechnology, 2008, 19: 055604.
[15] OUYANG M, BAI R, YANG L G., CHEN Q, HAN Y G, WANG M, YANG Y, CHEN H.Z. High photoconductive vertically-oriented TiO2 nanotube arrays and their composites with copper phthalocyanine [J]. J Phys Chem C, 2008, 112: 2343-2348.
[16] CAO L, CHEN H Z, WANG M, SUN J Z. Photoconductivity study of modified carbon nanotube/oxotitanium phthalocyanine composites [J]. J Phys Chem B, 2002, 106: 8971-8975.
[17] JIN S, LIU X R, ZHANG W, LU Y, XUE G. Electrochemical copolymerization of pyrrole and styrene [J]. Macromolecules, 2000, 33: 4805-4808
[18] CAO L, CHEN H Z, ZHOU H B, ZHU L, SUN J Z, ZHANG X B, XU J M, WANG, M. Carbon-nanotube-templated assembly of rare-earth phthalocyanine nanowires [J]. Adv Mater, 2003, 15: 909-913.
Foundation item: Project(NCET-06-0536) supported by the Program for New Century Excellent Talents in University of China; Project(Y4090260) supported by the Program for Zhejiang Provincial Natural Sicence Foundation of China
Corresponding author: ZHANG Cheng; Tel/Fax: +86-571-88320253; E-mail: czhang@zjut.edu.cn
DOI: 10.1016/S1003-6326(09)60072-8
(Edited by YANG Hua)