
J. Cent. South Univ. (2018) 25: 1-8
DOI: https://doi.org/10.1007/s11771-018-3711-1

Effects of directional solidification parameters and crystal selector on microstructure of single crystal of Ni-base superalloys
LI Chong-he(李重河)1, 2, 3, WEI Chao(魏超)3, ZHANG Ru-lin(张如林)4,
WANG Hong-bin(汪宏斌)1, 2, REN Zhong-ming(任忠鸣)1, LU Xiong-gang(鲁雄刚)1,2
1. State Key Laboratory of Advanced Special Steel & Shanghai Key Laboratory of Advanced
Ferrometallurgy & School of Materials Science and Engineering, Shanghai University,Shanghai 200072, China;
2. Shanghai Special Casting Engineering Technology Research Center, Shanghai 201605, China;
3. Shanghai Aircraft Manufacturing Co. Ltd., Shanghai 200436, China;
4. Shanghai Dianji University, Shanghai 201306, China
Central South University Press and Springer-Verlag GmbH Germany,part of Springer Nature 2018
Abstract: The single crystal of nickel-base super alloy is widely used for making turbine blades. The microstructure of the alloy, especially the deviation of preferred orientation of single crystal, possesses the most important effects on the mechanical properties of the blades. In this study, the single crystal ingot and blade of DZ417G alloy are prepared by means of the spiral crystal selector as well as the directional solidification method, and the effect of the parameters (i.e., the shape of samples, the withdrawal rate) and the structure of the spiral crystal selector on the formation of single crystal and the crystal orientation are investigated. This method can prepare not only the single crystal ingot with simple shape but also the single crystal blades with the complex shape, the simple with rod-shape can form the single crystal easily with a relatively fast withdrawal rate, but the blade with complex shape requires much slower withdrawal rate to form single crystal. The length of the crystal selector almost has no effect on the crystal orientation. However, the angle of selector plays an obvious role on the orientation; the selector with a smaller angle can effectively reduce the deviation of preferred orientation; the appropriate angle of selector to obtain optimal orientation is found to be around 30°and the deviation of preferred orientation is about 30° for this selector.
Key words: DZ417G alloy; high rapid solidification; crystal orientation; single crystal superalloy; spiral grain selector
Cite this article as: LI Chong-he, WEI Chao, ZHANG Ru-lin, WANG Hong-bin, REN Zhong-ming, LU Xiong-gang. Effects of directional solidification parameters and crystal selector on microstructure of single crystal of Ni-base superalloys [J]. Journal of Central South University, 2018, 25(1): 1–8. DOI:https://doi.org/10.1007/s11771-018-3711-1.
1 Introduction
The blade of nickel-base super alloy attracts a lot of attention in the world due to its excellent properties for making turbine blade [1]. The performance of the super alloy is strongly affected by the microstructure which is determined by melting and casting process [2]; historically, the solidification structures of turbine blades have developed and progressed from the equiaxed microstructure to directionally solidified (DS) and then to single crystal (SX) [3]. The transition from DS to SX can be achieved by introducing a crystal selector which consists of two parts: a starter block referring to the grain orientation optimization and a spiral part (as shown in Figure 1) to ensure that only one grain can eventually survive and grow into the blade.
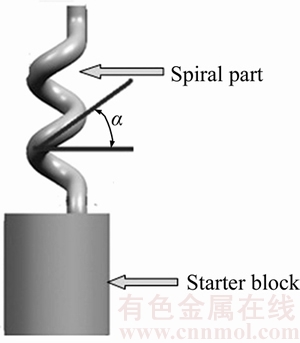
Figure 1 Schematic of spiral grain selector
It is well known that the mechanical properties of the Ni-based super alloys are not isotropic [4]. For instance, blades with [001] crystal index have higher temperature creep strength compared to those with [011] or [111] index. During the growth of grains, the preferred growth direction is known to be [001]. The goal of single crystal casting is to align the [001] single crystals along the length of the turbine blade. However, this unidirectional process does not always assemble such turbine blades. The deviation of preferred orientation of single crystal super alloys from [001] often occurs and it becomes one of the most important defects. In general, the product with less than 10° deviation of preferred orientation is judged to be well oriented [5].
DZ417G alloy [6] is a directionally solidified Ni-base super alloy without any expensive elemental additions, such as the elements W, Nb, Ta and Hf. It is the candidate material for the blades of advanced air engines because of its good integrated properties, including LCF (low cycle fatigue), HCF (high cycle fatigue) and oxidation resistant at various temperatures. It is designed solely for unidirectional solidification.
In this work, by means of the spiral crystal selector as well as the directional solidification method, the single crystal ingot and blade of DZ417G alloys are prepared. The effects of the parameters (including the shape of specimen, the withdrawal rate, and the shape of the spiral grain selection device) on the microstructure of the super alloy are studied. In order to get a smaller deviation of preferred orientation, the angle of the crystal selector (α, as shown in Figure 1) and the length of the spiral part are also studied in this work.
2 Experiments
Experiments are carried out in the home-made vacuum directional solidification furnace [7] (The schematic is shown in Figure 2). In the recent experiments, the DZ417G alloy is used, with composition (mass fraction) as follows: C 0.18%, Cr 8.96%, Mo 3.08%, Co 9.72%, V 0.86%, B 0.015%, A1 5.41%, Ti 4.50%, Fe 0.23%, P 0.002%, S 0.002%, Si 0.04%, Mn 0.05%, and the balance Ni. The shell mold is made of the corundum and the spiral grain selection device is located at the bottom of the mold.
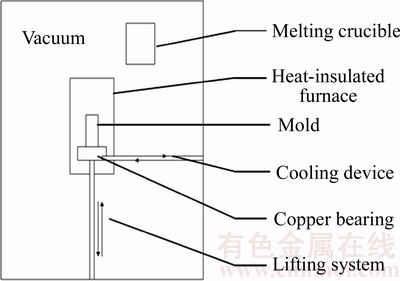
Figure 2 Schematic of vacuum unidirectional solidification furnace
In order to obtain single crystal ingots and blades, three withdrawal rates (R), 6.8, 4.8, and 1.5 mm/min, are used. Each mold contains an ingot of d15 mm×120 mm and a blade, as shown in Figure 3. The melt of alloys is heated and held at 1520 °C.
In order to investigate the relationship between the crystal orientation and the structure of the spiral crystal selector, six ingots (d15 mm×120 mm) are assembled in one mold and the differences between the six ingots are the angle and length of the selector, as shown in Table 2. The low withdrawal rate (1.5 mm/min) is adopted and other conditions are kept constant to ensure that the single crystal can be obtained for each ingot.
After the process of unidirectional solidification is finished, the ceramic shell is broken and the ingots and blades of the alloy are obtained.
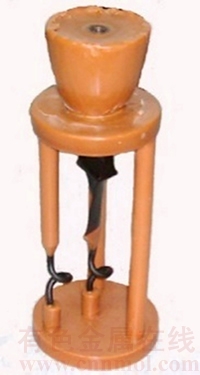
Figure 3 Wax pattern of mold
Table 1 Judgments of macro pictures
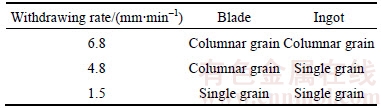
Table 2 Differences between six ingots
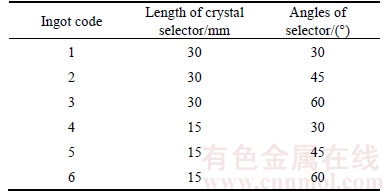
They are then eroded by the macro-corrosion reagent: H2O2 (1.5 mL)+HCl (5 mL, 36.5%). The macro-structures are shown in Figure 4. The micro-corrosion reagent is CuSO4 (20 g)+HCl (50 mL, 36.5%)+H2O (100 mL). The LEICA optical microscope is used to observe the microstructure. Electron backscattered diffraction (EBSD) and X-ray diffraction (XRD) are used to detect the crystal orientation.
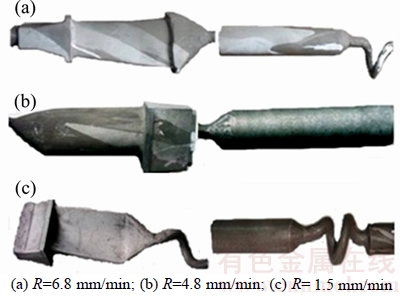
Figure 4 Macro pictures of blades and test rods after corrosion:
3 Results and discussion
3.1 Mechanism of crystal selection
By analyzing the macro-structure of the cross- sections of different parts of the spiral crystal selector, the mechanism of the crystal selection can be better understood. At the top of the starter area, there are a number of grains. The different colors correspond to different orientations, as shown in Figure 5. When the crystals grow into the spiral crystal selector, there are about three to five grains; when the crystal selection behavior occurs, the number of grains is reduced, and finally only one grain leaves at the exit of the crystal selector. This grain grows into a single crystal ingot, as shown in Figure 6. This is consistent with ZHENG’s simulation of the grain selection behavior [8].
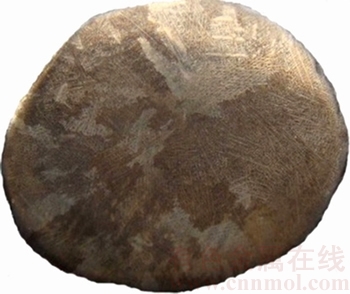
Figure 5 Macro picture of top of starter block after corrosion
HU [9] suggested that the selector can only perform crystal selection and eliminate unwanted grains if the length of the grain selection device is longer than 20 mm.
In this study, the length of spiral grain selection device is 50 mm. By observing the macro pictures of the cross-section of the crystal selector at 1/4, 1/2, and 3/4 height of three specimens with different withdrawing rates (Figure 6), it is found that at 1/4 height of the crystal selector, there are two or three grains; at 1/2 position, there is only one grain for all three samples; at 3/4 position, the sample with a faster withdrawing rate forms a stray grain, and the grains of the other two sample form columnar crystals. These results imply that when the withdrawing rate is low, then it has no effect on the behavior of the spiral grain selector. The formation of the single crystal depends on the length of the grain selection device.
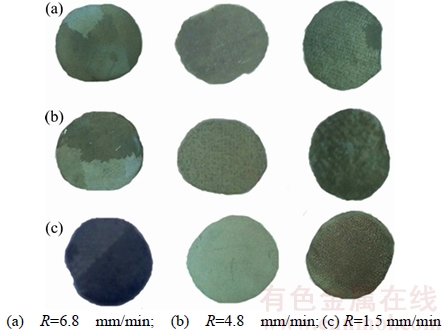
Figure 6 Macro picture of cross-section of crystal selector at 1/4, 1/2, and 3/4 height (ordered from left to right):
3.2 Effects of withdrawal rates on formation of single crystal
The ingot and blade are eroded by macro- corrosion reagent for the macro-structure analysis and their macrostructure are shown in Figure 4. The experiment results indicate: 1) the ingot and blade prepared with the withdrawal rate 6.8 mm/min are constituted of a number of grains and their structure are columnar crystal; 2) the blade with the withdrawal rate 4.8 mm/min is constituted of a number of grains and is columnar crystal, but no grain boundary is observed for the ingot with the same rate and it is a single crystal; 3) both the ingot and blade obtained with the withdrawing rate 1.5 mm/min have no surface grain boundary and they are single crystal.
After analyzing the mechanism of formation of stray grain, TIEN and COPLEY [10] indicated that the stray grain could not be formed when the solidifying potential heat could be conducted through the solid part as no residual heat accumulated around the solid/liquid interface. Single crystals could be formed when the solidification rate R satisfies the following relationship:
RTGS/△H (1)
where KT is solid conductivity; GS is temperature grade in the solid phase around interface; △H is latent heat.
For the DZ417G alloy, KT and △H are constant, and the temperature gradient in the solid phase around interface, GS also remains unchanged. One can only reduce the withdrawal rate R (equivalent to the solidification rate R) to satisfy the relationship of the above formula.
From the macro-structure analysis in Figure 4, it may be seen that for the DZ417G alloy, either the rod or the blade can form the single crystal structure by using the directional solidification method as well as the spiral grain selector, but the different withdrawal rate is required. The rod with the simple shape can form single crystal easily with relatively fast withdrawal rate (R=4.8 mm/min); however, the blade with the complex shape needs slower withdrawal rate (R=1.5 mm/min) to form the single crystal. This is reasonable and can be explained as follows: it is well-known that two conditions are required to form the single crystal, one is that the crystal must grow from one nucleus [11], another is that the thermal transfer is perpendicular to the solid/liquid interface of growth of samples and there is no new nucleation in the liquid ahead of solid/liquid interface. The stray crystals appeared on the turbine blade where there are large sections changes of the mold, therefore, the blade needs more slow rate to suppress the formation of stray crystal and finally forms single crystal.
This work is in accordance to the RAPPAZ’s CAFE model [12]. RAPPAZ believes that the formation of the single crystal cast turbine blade is related to: the crystal selection mechanisms in the columnar zone of DS (directional solidification) turbine blades, the transition from columnar-to- equiaxed structures, the formation of stray crystals at section changes of the mold, and the influence of the thermal gradient direction on the shape of the grain boundaries. In this work, the grain selection mechanisms and the transition from columnar-to- equiaxed structures occurred in the spiral crystal selector, and stray crystals appeared on the turbine blade where there are large section changes of the mold.
The micro-structures of the cross-section of ingots with different withdrawal rates are shown in Figure 7, and show dendrite morphologies. When the withdrawing rate is reduced, the primary dendrite arm spacing is increased.
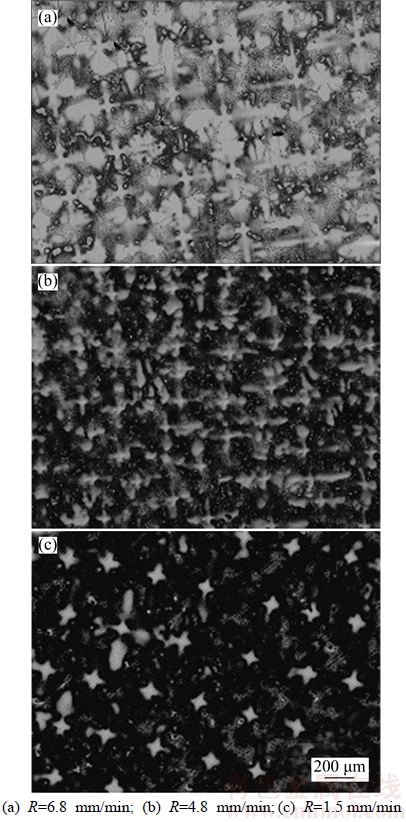
Figure 7 Dendrite morphologies at different withdrawal rates:
The primary dendrite arm spacing is measured by Planimetry:
(2)
where A is the area of the field; n is the number of dendrites in the field.
For each sample, the area of the field and the numbers of dendrites are measured in the different fields. They are then used to calculate the primary dendrite arm spacing, and the average value of the fields is defined as the primary dendrite arm spacing of this sample. The primary dendrite arm spaces at different withdrawal rates are shown in Figure 8. When the withdrawal rate is reduced, the primary dendrite arm spacing is increased.
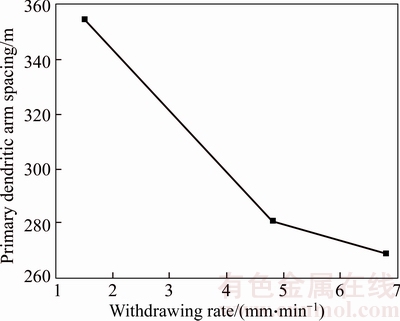
Figure 8 Effect of withdrawing rates on primary dendrite arm spacing
In the solidification of dendrite, the primary dendrite arm spacing is proportional to the term GL-1/2 R-1/4 (GL is the temperature gradient in liquid phase around interface and R is the solidification rate) [13]. When the withdrawal rate R is decreased, the temperature gradient in liquid phase around interface GL is increased. However, at low withdrawal rate, when the withdrawal rate R is reduced, the temperature grade GL is almost unchanged. Therefore, the value of GL-1/2 R-1/4 is increased when the withdrawing rate is decreased, and the primary dendrite arm spacing is increased when the withdrawing rate is reduced, as shown in Figure 8.
According to the theory of super cooling solidification [14], the shape of the solid–liquid interface can be estimated by the following formula:
(3)
where GL is the temperature gradient in liquid-phase around the interface; R is the solidification rate; mL is the liquid phase slope in alloy phase diagram; C0 is the solute content of the alloy; k is the solute partition coefficient; DL is the liquid diffusion coefficient of the solute.
When the value of GL/R is larger than the right term in the formula, the solid-liquid interface is planar. When the temperature gradient GL is unchanged, and/or changes a little, the value of GL/R decreases as the solidification rate R is increased. As GL/R gradually become smaller than the right term, the planar interface becomes unstable, and the solidification interface shape changes from planar to cellular or dendritic. The value of GL/R in our experiment is small, so the solidification structure is assumed to be dendritic. When the withdrawal rate V is decreased, R decreases, GL/R increases, and the growth of dendrites may be promoted. This will lead to the increasing of secondary dendrites.
The reduced solidification rate at fixed gradient increases the solidification time and the secondary dendrite arm spacing, thereby increasing the permeability of the dendritic network. However, in our experiments, the samples are as-cast. The dendritic network formed a mushy zone, so the secondary dendrite is not seen in the microstructure observation.
3.3 Effects of parameters of grain selector on crystal orientation
The ingots prepared using different angles of the crystal selector are shown in Figure 9. All ingots are metallurgical single crystals and no grain boundary on the surface can be found. The grain orientation is measured by EBSD and XRD.
The XRD pole figures of all samples are shown in Figure 10. Almost all of six samples seem to illustrate the microstructure of single crystal,except that the sample 4 has two grains. The samples 3 and 5 have a trend to be polycrystalline but they are single crystals. This result is consistent with the theory of spiral geometries [15]. When the angle of the grain selector (α) is too small, the growth of all grains may be prevented and almost all grains disappear on the walls of the grain selector. On the other hand, when α is too large, the grain selection may take place in the channel, but the selector may not efficiently eliminate non-need grain on the walls of the grain selector and may not guarantee only one grain growing. In the recent study, the angles are 30°, 45° and 60°, which are neither too large nor too small. The mould with this three angle crystal selector can be used to successfully prepare single crystal.
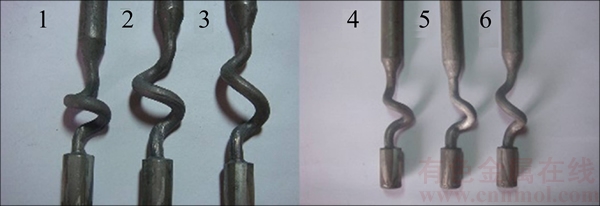
Figure 9 Macro pictures of ingots after corrosion
The EBSD inverse pole figures of six ingots are showed in Figure 11 and the angular deviation from preferred orientation [001] is calculated and shown in Table 3. All samples possess deviation from preferred orientation, the samples with same angle and different length of crystal selector have almost the same angular deviation from preferred orientation [001]. This fact may hint that the length of selector does not affect the deviation. On the other hand, keeping the length of the selector constant, the deviation from the preferred orientation [001] increases with increasing the angle of selector and the crystal selector with a smaller angle can effectively reduce the deviation from preferred orientation. In this study, the appropriate angle of the selector is found to be around 30°.
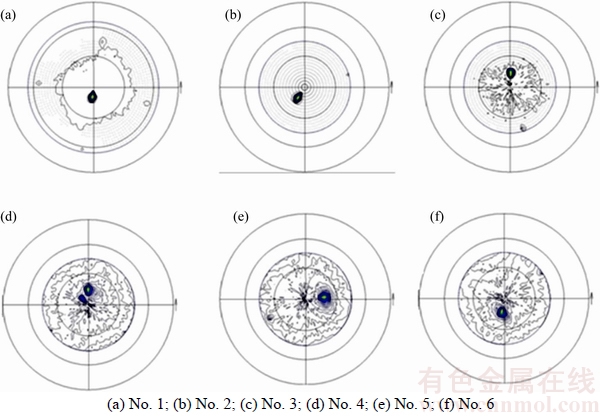
Figure 10 XRD pole figures of six ingots:
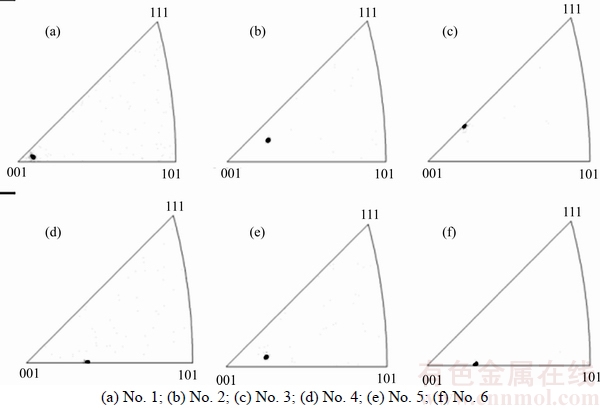
Figure 11 Inverse pole images of six ingots:
Table 3 Angles deviated from preferred orientation [001] of samples 1–6
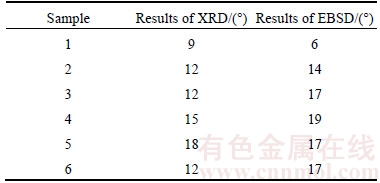
4 Conclusions
Using the directional solidification method and the spiral grain selector, the single crystal ingot and blades of DZ417G are prepared under proper process conditions, and the following conclusions are obtained:
1) The formation of the single crystal alloys was related to the withdrawal rate during the directional solidification. The more complex the shape of ingot is, the lower the withdrawal rate is needed.
2) The length of the grain selector had a great influence on the formation of the single crystal though it had nothing to do with crystal orientation.
3) The angle of selector may play an obvious role on the orientation and the appropriate angle of selector to obtain optimal orientation was found to be around 30° and the deviation of preferred orientation is about 30° for this selector.
References
[1] HU Zhuang-lin, LIU Li-rong, JIN Tao, SUN Xiao-feng. Development of the Ni-base single crystal superalloys [J]. Aero Engine, 2005, 31(3): 1–7. (in Chinese)
[2] CHEN Rong-zhang. Development status of single crystal superalloys [J]. Journal of Materials Engineering, 1995(8): 3–12. (in Chinese)
[3] SEO S M, KIM I S, LEE J H, JO C Y, MIYAHARA H, OGI K. Grain structure and texture evolutions during single crystal casting of the Ni-base superalloy CMSX-4 [J]. Metals and Materials International, 2009, 15(3): 391–398.
[4] DONG H B. Analysis of grain selection during directional solidification of gas turbine blades [C]// Proceedings of the World Congress on Engineering. London, UK: WCE, 2007: 1257–1262.
[5] ESAKA H, SHINOZUKA K, TAMURA M. Analysis of single crystal casting process taking into account the shape of pigtail [J]. Materials Science and Engineering A, 2005, s413–414(6): 151–155.
[6] GUO Jian-ting. A directionally solidified nickel-base superalloy DZ417G with excellent properties and low cost [J]. Acta Metallurgica Sinica, 2002, 38(11): 1163–1174. (in Chinese)
[7] LI C H, GUO Z M, ZHU M, LU X G, YE X S, ZHANG P X, ZHAI Q J. Preparation of single crystal of TiNi alloy and its shape memory performance [J]. Processing of SPIE- The International Society for Optical Engineering, 2009, 478(1, 2): 404–410.
[8] ZHENG Qi, HOU Gui-cheng, TIAN Wei-min, JIN Tao, SUN Xiao-feng, GUAN Heng-rong, HU Zhuang-lin. Grain selection behavior of single crystal superalloy [J]. The Chinese Journal of Nonferrous Metals, 2001(2): 176–179. (in Chinese)
[9] HU H Z, GUO J J, LIU L, LI J S. Progress of directional solidification and processing of advanced materials [M]. Beijing: Science Press, 2008: 538. (in Chinese)
[10] TIEN J K, COPLEY S M. The effect of uniaxial stress on the periodic morphology of coherent gamma prime precipitates in nickel-Base superalloy crystals [J]. Metallurgica Trans- actions, 1971, 2: 215–219.
[11] BOISTELLE R, ASTIER J P. Crystallization mechanisms in solution [J]. Journal of Crystal Growth, 1988, 90(1–3): 14–30.
[12] RAPPAZ M, GANDIN C A, DESBIOLLES J L, THEVOZ P. Prediction of grain structures in various solidification processes [J]. Metallurgical and Materials Transactions A, 1996, 27(3): 695–705.
[13] HU Han-qi. Principle of metal solidification [M]. Beijing: Mechanical Industry Press, 1992.
[14] TILLER W A, JACKSON K A, RUTTER J W. The redistribution of solute atoms during the solidification of metals [J]. Acta Metallurgica, 1953, 1(4): 428–437.
[15] CARTER. P, COX D C, GANDIN C A, REED R C. Process modelling of grain selection during the solidification of single crystal superalloy castings [J]. Materials Science and Engineering A, 2000, 280(2): 233–246.
(Edited by HE Yun-bin)
中文导读
定向凝固参数和选晶器对镍基高温合金单晶组织的影响
摘要:镍基单晶超合金由于其优异的性能,被广泛运用于制备航空涡轮叶片。本文采用快速凝固法(HRS)和螺旋选晶法相结合的办法,通过改变螺旋选晶器的角度,制备不同取向和形状的单晶试棒。研究了样品形状,抽拉速率以及选晶器结构对单晶形成及取向的影响。结果表明:简单棒状和复杂叶片形状的镍基单晶均可通过该技术手段获得。同时,棒状合金能在较快的抽拉速率下获得单晶组织,而复杂的叶片形状则需要在较慢的抽拉速率才能获得所需的单晶组织。选晶器的长度对于晶粒取向无影响,但是选晶器角度对晶粒取向有很重要的影响,小角度选晶器能减少晶粒的偏移,很显然,30°是较为合适的选晶器角度。
关键词:DZ417合金;晶体取向;单晶超合金;螺旋选晶器
Foundation item: Project(51074105) supported by the National Natural Science Foundation of China; Projects(08DZ1130100, 10520706400) supported by the Science and Technology Commission of Shanghai Municipality, China; Project(2007CB613606) supported by the National Basic Research Program of China
Received date: 2016-05-10; Accepted date: 2017-12-01
Corresponding author: LI Chong-he, PhD, Professor; Tel: +86–21–566332934; E-mail: chli@staff.shu.edu.cn; ORCID: 0000-0002- 9150-4479