
Microstructures and tensile properties of as-extruded Mg-Sn binary alloys
CHEN Dong(陈 冬), REN Yu-ping(任玉平), GUO Yun(郭 运),
PEI Wen-li(裴文利), ZHAO Hong-da(赵宏达), QIN Gao-wu(秦高梧)
Key Laboratory for Anisotropy and Texture of Materials of Ministry of Education,
Northeastern University, Shenyang 110004, China
Received 23 September 2009; accepted 30 January 2010
Abstract: The microstructure and mechanical properties of Mg-xSn (x=3, 7 and 14, mass fraction, %) alloys extruded indirectly at 300 ?C were investigated by means of optical microscopy, scanning electron microscopy and tensile test. The grain size of the α-Mg matrix decreases from 220, 160 and 93 μm after the homogenization treatment to 28, 3 and 16 μm in the three alloys after extrusion, respectively. The results show that the grain refinement is most remarkable in the as-extruded Mg-7Sn alloy. At the same time, the amount of the Mg2Sn particles remarkably increases in the Mg-7Sn alloy with very uniform distribution in the α-Mg matrix. In contrast, the Mg2Sn phase inherited from the solidification with a large size is mainly distributed along grain boundary in the Mg-14Sn alloy. The tensile tests at room temperature show that the ultimate tensile strength of the as-extruded Mg-7Sn alloy is the highest, i.e., 255 MPa, increased by 120% as compared with that of as-cast samples.
Key words: Mg-Sn alloy; extrusion; tensile property; microstructure
1 Introduction
Due to the strong requirement of reducing weight in vehicle to fulfill energy saving, there has been increasing research interest in the magnesium alloys of various structural components of spacecraft and automobiles. Among them, Mg-Al based alloys are widely used because they have excellent castability and reasonable mechanical properties at ambient environment[1-3]. However, Mg-Al based alloys have a poor creep resistance at the elevated temperature (120-200 ?C) since Mg17Al12 phase has a low melting point (462 ?C) and thus experiences grain growth under service at such high temperature mentioned above[4-5]. Mg-RE (RE: rare earth elements) alloys have a excellent creep-resistance property for the high melting point and stable precipitation intermetallics can stabilize grain boundaries of α-Mg matrix and impede motion of dislocations at elevated temperatures[6-9]. But considering the high cost of RE additions, it is necessary to explore novel heat resistant Mg alloys that are RE-free.
Because there exists a high Sn solubility limit and thermally stable Mg2Sn phase in the Mg-Sn binary system, Mg-Sn based alloys have been increasingly considered as the potential heat resistant Mg alloys[10-15]. LIU et al[12] studied the mechanical properties of as-cast Mg-Sn alloys and found that the ultimate tensile strength (UTS) is about 120 MPa in Mg-5%Sn (mass fraction) alloy. The corrosion resistance of Mg-Sn-Ca alloys after extrusion at 350 ?C was improved as compared with those of as-cast ones[13]. SASAKI et al[14] investigated the Mg-Sn-Zn-Al alloys that were extruded at 250 ?C. The extruded alloy exhibits UTS of 354 MPa. More recently, heat-treatable Mg-Sn-Zn wrought alloy was investigated. The results indicated that both room-temperature tensile and high-temperature (175 ?C) creep properties were enhanced by ageing at 200 ?C after extrusion at 260 ?C[15].
However, less research has been carried out on deformed Mg-Sn binary alloys. Therefore, in this work, the Mg-xSn (x=3, 7 and 14, mass fraction, %) alloys in as-homogenized and as-extruded states have been investigated by means of optical microscopy, scanning electron microscopy and tensile test at room temperature in order to establish the relationship between the microstructure and mechanical properties of the deformed Mg-Sn alloys and thus to provide the basic information for designing new deformed heat-resistant Mg-Sn based alloys.
2 Experimental
Nominal compositions of the Mg-Sn alloys used in this study are listed in Table 1. Pure magnesium (99.95%, mass fraction) and pure tin (99.99%, mass fraction) were melted in electric resistance furnace at 760 ?C with mechanical stirring under a flowing protective gas (N2+2‰SF6). The ingots have a diameter of 60 mm, which were homogenization treated at 480 ?C for 12 h. The extrusion samples with a diameter of 46 mm were machined out from the homogenized ingots. Before extrusion, the extrusion ingots were held at 300 ?C for 30 min. As-extruded bars were indirectly extruded out of a mould in the diameter of 12 mm at a speed of about 7 mm/s, and then were machined into tensile specimens of 5 mm in diameter and 30 mm in length. The tensile test was carried out at room temperature on a tensile test machine (CMT5105) with a speed of 2 mm/min. Three samples of each alloy were employed to obtain averaged data of mechanical properties.
The microstructures of alloys, etched by acetic glycol, were observed by OLYMPUS GX71 optical microscopy (OM). Scanning electron microscopy (SEM) (JSM-7001F) was used to identify the precipitated Mg2Sn phase. The grain size of the alloys was measured by an average liner intercept method.
Table 1 Nominal chemical compositions of Mg-Sn alloys (mass fraction, %)
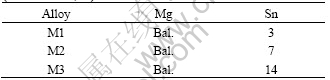
3 Results
3.1 Microstructure of Mg-Sn alloys
Microstructures of the as-homogenized specimens of alloys M1, M2 and M3 are shown in Fig. 1. The three alloys are composed of equiaxed grains. The grain sizes of three alloys are 220, 160 and 93 μm, respectively, as shown in Fig.2. This indicates that the grains are refined with the increase of Sn content. Most of Mg2Sn phase dissolves in Mg matrix in the M1 and M2 alloys and only a little Mg2Sn phase exists at grain boundaries after homogenization treatment at 480 ?C for 12 h (Figs.1(a) and (b)). In contrast, in the alloy M3, a large number of large Mg2Sn phase inherited from solidification remains at grain boundaries of α-Mg after homogenization treatment.
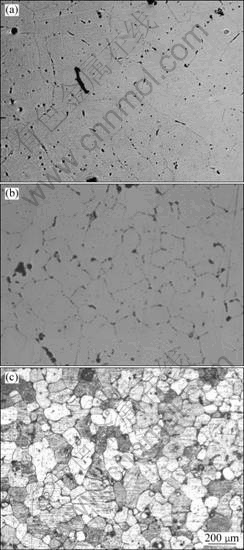
Fig.1 Optical images of as-homogenized specimens: (a) Alloy M1; (b) Alloy M2; (c) Alloy M3
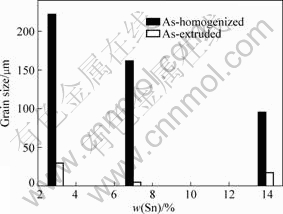
Fig.2 Grain sizes of as-homogenized and as-extruded alloys
Fig.3 shows the optical microstructures of three as-extruded alloys along the longitudinally- and transversely-extruded directions, respectively. They are
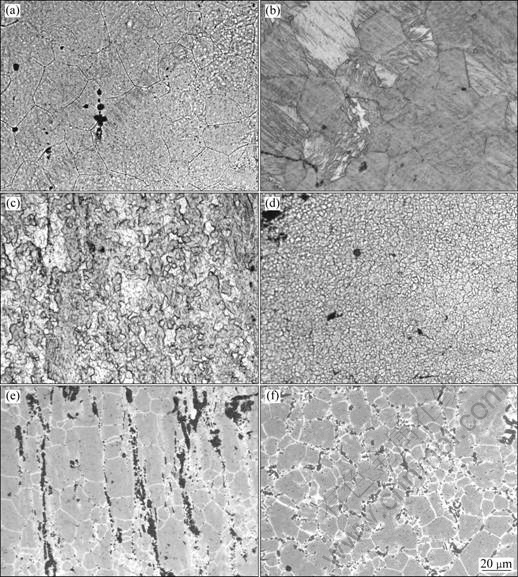
Fig.3 Optical images of as-extruded alloys: (a), (b) Alloy M1; (c), (d) Alloy M2; (e), (f) Alloy M3; (a), (c) and (e) Along longitudinally extruded direction; (b), (d) and (f) Along transversely-extruded direction
equiaxed grains in both longitudinal and transverse directions, indicating that complete dynamic recrystallization happens during hot extrusion at 300 ?C. The grain sizes of as-extruded alloys shown in Fig.2 decrease to 28, 3 and 16 μm, respectively, for the three alloys after extrusion, which are obviously refined compared with those of the as-homogenized alloys. Very interestingly, the grain refinement is most remarkable in the as-extruded alloy M2, reaching 3 μm.
At the same time, the Mg2Sn phase precipitates during hot extrusion, as shown in Fig.4. A little Mg2Sn particles are found in the alloy M1 (Fig.4(a)), but the amount remarkably increases in the alloy M2 with very uniform distribution in the α-Mg matrix (Fig.4(b)). In contrast, very large sized Mg2Sn phase exists in the alloy M3, as shown in Fig.4(c), which is expected to be inherited from the solidification and it cannot be dissolved sufficiently during the homogenization treatment. After extrusion, the Mg2Sn phase distributes at the grain boundaries of α-Mg in the transverse direction in the alloy M3, but changes to strip-shape along the longitudinally extruded direction.
3.2 Mechanical properties of extruded Mg-Sn alloys
Tensile properties of as-extruded specimens at room temperature are shown in Fig.5. The ultimate tensile strengths of three alloys are 208, 255 and 215 MPa, respectively. The results indicate that UTS increases remarkably after extrusion compared with that of the as-cast ones. The alloy M2 has the highest UTS, i.e., 255 MPa, increased by 120% as compared with that of as-cast samples. Elongations of three alloys are 6.8%, 4.4% and 3.3%, respectively, and decrease with increasing Sn content of the Mg-Sn alloys.
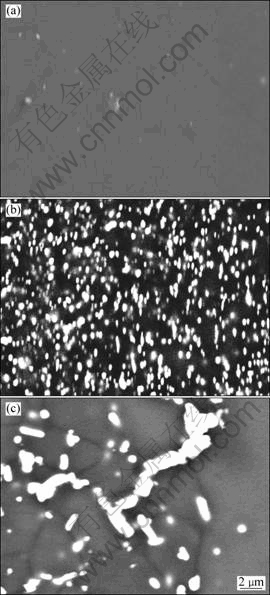
Fig.4 SEM-BSE images of as-extruded specimens in transverse direction: (a) Alloy M1; (b) Alloy M2; (c) Alloy M3
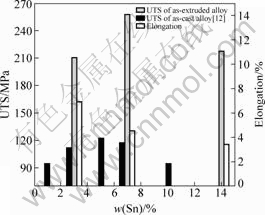
Fig.5 Tensile properties of as-extruded specimens at room temperature compared with those of as-cast Mg-Sn alloys[12]
4 Discussion
The present study shows that grain size decreases significantly after the hot extrusion at 300 ?C, from original 220, 160 and 93 μm to 28, 3 and 16 μm, for the Mg-3Sn, Mg-7Sn and Mg-14Sn alloys, respectively. According to previous reports[16-18], the refinement of grains is attributed to dynamic recrystallization during the extrusion, among which, the precipitation of Mg2Sn phase plays an important role in refining the α-Mg grains. Here, the grain size of the Mg-7Sn alloy is the smallest after extrusion. After homogenization, the Mg2Sn phase in the cast alloys can effectively dissolve in the α-Mg matrix but only little in the Mg-14Sn alloy. During the hot extrusion, many dislocations and sub-grain boundaries are generated, which provides more sites for the precipitation of the Mg2Sn phase. Only a little quantity of Mg2Sn phase precipitates in the Mg-3Sn alloy, but larger quantity of Mg2Sn phase with small size and uniform distribution in the Mg-7Sn alloy. In contrast, the large size Mg2Sn phase with non-uniform distribution, inherited from the as-cast state, exists in the Mg-14Sn alloy, which is not effective to hinder the growth of recrystallized grains of α-Mg matrix and thus its grain size is not as small as that of the Mg-7Sn alloy, as shown in Fig.3. In other words, the recrystallized grain size of α-Mg matrix during the hot extrusion depends on the volume fraction, size and size distribution of the Mg2Sn phase.
Fig.5 shows the mechanical properties of the as-extruded Mg-Sn binary alloys, as compared with those of the as-cast ones. It is indicated that UTS of the extruded alloys is improved greatly after hot extrusion. In particular, the UTS of the Mg-7Sn alloy is 255 MPa, more than two times that of the as-cast one. This is due to the fine microstructure formed through dynamic recrystallization and Mg2Sn precipitates during hot extrusion. According to the Hall-Petch relationship[19-20], grain refinement is an effective way to enhance strength of crystalline materials. Especially for the Mg alloys, the grain size effect on strength is more obvious than that of Al alloys since the strengthening coefficient K is much larger. The smaller size and more uniform distribution of the Mg2Sn phase in the Mg-7Sn alloy, precipitated in the α-Mg matrix during the hot extrusion, play also a precipitation-strengthening effect to contribute to the improvement of the strength. Both effects are expected to make the as-extruded Mg-7Sn alloy have the highest strength, as shown in Fig.5. This finding is interesting for development of new Mg-Sn based alloys in the future with further appropriate alloying and processing to control both size and size distribution of the second phase.
5 Conclusions
1) The α-Mg grain sizes of as-homogenized Mg-3Sn, Mg-7Sn and Mg-14Sn alloys are 220, 160 and 93 μm, respectively and this indicates that the grain is obviously refined with increasing Sn content.
2) Hot extrusion can significantly refine the grain size of α-Mg phase, and the grain sizes of the three as-extruded Mg-Sn alloys decrease to 28, 3 and 16 μm, respectively. A little Mg2Sn phase can be observed in Mg-3Sn alloy, but in Mg-7Sn alloy much more small Mg2Sn particles distribute uniformly in the α-Mg matrix. Large size Mg2Sn phase, inherited from solidification, exists in the Mg-14Sn alloy, making the grain refinement less effective.
3) Tensile strengths of the three alloys are obviously enhanced by extrusion, and the ultimate tensile strength of the as-extruded Mg-7Sn alloy is the highest, 255 MPa, increased by 120% as compared with that of the as-cast one.
References
[1] ZHANG H, YAN Q Q, LI L X. Microstructures and tensile properties of AZ31 magnesium alloy by continuous extrusion forming process [J]. Materials Science and Engineering A, 2008, 486(1/2): 295-299.
[2] RAVI KUMAR N V, BLANDIN J J, DESRAYAUD C, MONTHEILLER F, SU?RY M. Grain refinement in AZ91 magnesium alloy during thermomechanical processing [J]. Materials and Engineering A, 2003, 359(1/2): 150-157.
[3] WANG X F, ZHAO J Z, HE J, HU Z Q. Hot rolling characteristics of spray-formed AZ91 magnesium alloy [J]. Transactions of Nonferrous Metals Society of China, 2007, 17(2): 238-243.
[4] HORT N, HUANG Y D, LEIL T A, MAIER P, KAINER K U. Microstructural investigations of the Mg-Sn-xCa system [J]. Advanced Engineering Materials, 2006, 8(5): 359-364.
[5] WEI S H, CHEN Y G, TANG Y B, LIU H M, XIAO S F, NIU G, ZHANG X P, ZHAO Y H. Compressive creep behavior of as-cast and aging-treated Mg-5wt% Sn alloys [J]. Materials Science and Engineering A, 2008, 492: 20-23.
[6] PENG Q M, HOU X L, WANG L D, WU Y M, CAO Z Y, WANG L M. Microstructure and mechanical properties of high performance Mg-Gd based alloys [J]. Materials and Design, 2009, 30(2): 292-296.
[7] BETTLES C J, GIBSON M A, ZHU S M. Microstructure and mechanical behaviour of an elevated temperature Mg-rare earth based alloy [J]. Materials Science and Engineering A, 2009, 505(1/2): 6-12.
[8] MORDIKE B L. Development of highly creep resistant magnesium alloys [J]. Journal of Materials Processing Technology, 2001, 117(3): 391-394.
[9] MORDIKE B L. Creep-resistant magnesium alloys [J]. Materials Science and Engineering A, 2002, 324(1/2): 103-112.
[10] BAMBERGER M, DEHM G. Trends in the development of new Mg alloys [J]. Annual Review of Materials Research, 2008, 38: 505-533.
[11] LIM H K, SOHN S W, KIM D H, LEE J Y, KIM W T, KIM D H. Effect of addition of Sn on the microstructure and mechanical properties of Mg-MM (misch-metal) alloys [J]. Journal of Alloys and Compounds, 2008, 454(1/2): 515-522.
[12] LIU H M, CHEN Y G, TANG Y B, WEI S H, NIU G. The microstructure, tensile properties, and creep behavior of as-cast Mg-(1-10)%Sn alloys [J]. Journal of Alloys and Compounds, 2007, 440(1/2): 122-126.
[13] ABU LEIL T, HORT N, DIETZEL W, BLAWERT C, HUANG Y, KAINER K U, RAO K P. Microstructure and corrosion behavior of Mg-Sn-Ca alloys after extrusion [J]. Transaction of Nonferrous Metals Society of China, 2009, 19(1): 40-44.
[14] SASAKI T T, YAMAMOTO K, HONMA T, KAMADO S, HONO K. A high-strength Mg-Sn-Zn-Al alloy extruded at low temperature [J]. Scripta Materialia, 2008, 59(10): 1111-1114.
[15] SASAKI T T, JU J D, HONO K, SHIN K S. Heat-treatable Mg-Sn-Zn wrought alloy [J]. Scripta Materialia, 2009, 61(1): 80-83.
[16] LIU K, ZHANG J H, ROKHLIN L L, ELKIN F M, TANG D X, MENG J. Microstructures and mechanical properties of extruded Mg-8Gd-0.4Zr alloys containing Zn [J]. Materials Science and Engineering A, 2009, 505(1/2): 13-19.
[17] ZHAO Z D, CHEN Q, WANG Y B, SHU D Y. Microstructures and mechanical properties of AZ91D alloys with Y addition [J]. Materials Science and Engineering A, 2009, 515(1/2): 152-161.
[18] DING H L, LIU L F, KAMADO S, DING W J, KOJIMA Y. Study of the microstructure, texture and tensile properties of as-extruded AZ91 magnesium alloy [J]. Journal of Alloys and Compounds, 2008, 456(1/2): 400-406.
[19] YAMASHITA A, HORITA Z, LANGDON T G. Improving the mechanical properties of magnesium and a magnesium alloy through severe plastic deformation [J]. Materials Science and Engineering A, 2001, 300(1/2): 142-147.
[20] KIM H K, LEE Y I, CHUNG C S. Fatigue properties of a fine-grained magnesium alloy produced by equal channel angular pressing [J]. Scripta Materialia, 2005, 52(6): 473-477.
(Edited by LI Xiang-qun)
Foundation item: Project(2008S089) supported by the Key Laboratory Program of Liaoning Province, China; Project(2007010303025) supported by the Shenyang Talents Supporting, China; Project(50731002) supported by the National Natural Science Foundation of China
Corresponding author: QIN Gao-wu; Tel: +86-24-83683772; Fax: +86-24-83686455; E-mail: qingw@smm.neu.edu.cn
DOI: 10.1016/S1003-6326(09)60298-3