
Electrochemical performances of
AB5-type hydrogen storage alloy modified with Co3O4
ZHANG Qing-qing1, SU Geng2, LI Ao-sheng1, LIU Kai-yu1
1. School of Chemistry and Chemical Engineering, Central South University, Changsha 410083, China;
2. State Key Laboratory of Powder Metallurgy, Central South University, Changsha 410083, China
Received 12 July 2010; accepted 24 March 2011
Abstract: Anodic electrodes with the mixture of hydrogen storage alloys and different contents of Co3O4 (2%, 4%, 6% and 8%, mass fraction) powders were made. The effects of Co3O4 on the electrochemical performance of the alloy electrodes were studied. The constant charge-discharge tests show that the discharge capacity of alloy electrodes with Co3O4 significantly increases, and the maximum discharge capacities of electrodes with 2%, 4%, 6% and 8% Co3O4 are higher than the electrode with no Co3O4 by 0.83%, 4.86%, 7.18% and 9.21%, accordingly. Linear polarization (LP) and electrochemical impedance spectroscopy (EIS) tests suggest that charge-transfer resistance decreases by the addition of Co3O4. Cyclic voltammogram (CV), scanning electron microscopy (SEM) and energy dispersive spectrum (EDS) tests indicate that Co3O4 can partly dissolve and experience a reversible oxidation-reduction process of Co to Co (OH)2, leading to the improvement in the electrochemical performance of hydrogen storage alloy.
Key words: Co3O4; hydrogen storage alloy; capacity; electrochemical performance
1 Introduction
Commercial AB5-type rare earth based hydrogen storage alloys are widespread used as anodic material for Ni-MH batteries due to their inherent advantages, such as high electrochemical capacity, long cycle life, better over charge/discharge performance and excellent environmental compatibility [1-3]. However, there is still a gap to meet the requirements of power source used in electric vehicle (EV) or hybrid electric vehicle (HEV). Hence, the electrochemical performances of the hydrogen storage alloy need to be improved.
Various methods have been applied to improving the electrochemical performance of AB5-type rare earth based hydrogen storage alloys during the past decades, such as element optimization of alloy composition [4-6], melioration of preparation technics [7-8], surface treatments [9-10] and modification of metallic oxides additives [11-12]. Modification of metallic oxides additives is the easiest method, which can also obtain rather good performance improvement of hydrogen storage alloy. It was found that some transitional metal oxides such as MnO2, V2O5, CoO, CuO, Co3O4 and RuO2 have a catalytic effect on the solid-gas reaction [13-14]. They can be used as catalysts or modified hydrogen storage alloy to improve the hydriding and dehydriding kinetics. The initial capacity and high rate discharge ability of La1.3CaMg0.7Ni9 modified with TiO2, Cr2O3, and ZnO were improved significantly [11]. CuO was added into AB5-type hydrogen alloy to improve electrode conductibility and increase specific energy [12]. Co3O4 was thought as a good kind of catalyst and electrode material, which has been used in lithium ion battery and electrochemical capacitor. Nevertheless, there is few study about Co3O4 used in Ni-MH battery.
In this work, hydrogen storage alloy MlNi3.9Co0.4Mn0.3Al0.3Cu0.2 is modified by different contents of Co3O4 (2%, 4%, 6% and 8%, mass fraction). The overall electrochemical performances of the modified electrodes were investigated in detail.
2 Experimental
2.1 Preparation of electrodes
Commercial alloy MlNi3.9Co0.4Mn0.3Al0.3Cu0.2 (The La-rich Mm contains 65.25% La, 25.20 % Ce, 8.43 % Pr, and 1.12 % Nd), and nickel powder as conductive agents and Co3O4 were weighed accurately at different mass ratios of 100:200:0,100:200:2, 100:200:4, 100:200:6, 100:200:8. The mixture was cold pressed into a circular pellet of 13 mm in diameter under a pressure of 20 MPa. Afterwards, the circular pellets were pressed with two foam nickel sheets in two sides and a foam nickel lead was attached to the pressed sheet for tests.
2.2 Electrochemical measurements
Galvanostatic charge/discharge tests were performed on a CT2001A battery-testing instrument (LAND, China). The open simulated cell was assembled with a metal hydride alloy electrode as working electrode, a sintering Ni(OH)2 electrode as counter electrode and a 6 mol/L KOH solution as electrolyte. It should be noted that the capacity of the sintering Ni(OH)2 electrode is higher than that of the working electrode. The cells were circularly charged at a current density of 60 mA/g-1 for 6 h, followed by a 5 min rest and then discharged at the same current density to a cut-off 1 V.
The cyclic voltammetric (CV), polarization and electrochemical impedance spectroscopy (EIS) experiments were all carried out on a PARSTAT 2273 advanced electrochemical system. A standard three-electrode system was utilized in all these electrochemical tests with a metal hydride alloy electrode as working electrode, a sintering Ni(OH)2 electrode as counter electrode and a Hg/HgO electrode as reference electrode in a 6 mol/L KOH solution. Cyclic voltammetric tests were performed in the scanning potential range from -1.2 V to -0.3 V. The linear polarization curves were recorded over a shifting potential from -5 mV to 5 mV at scan rate of 1 mV/s at 50% depth of discharge (DOD). The EIS studies were conducted from 100 kHz to 1 mHz with the amplitude of 5 mV at 50% DOD. All the tests were carried out at room temperature.
2.3 Physical characterization
The surface configuration was characterized by using scanning electron microscope (SEM, JSM-6360LV) and surface elements were analyzed by energy dispersive X-ray spectroscopy (EDX).
3 Results and discussion
3.1 Electrochemical performances
Figure 1 illustrates the discharge capacity of hydrogen storage alloy electrodes with different contents of Co3O4 in the first 10 cycles. As shown in Fig. 1, the electrodes with Co3O4 need less cycles to be activated than that with no Co3O4. And the discharge capacity of the former is much higher than the latter. It can also be observed that the discharge capacity increases with increasing the content of Co3O4. The maximum discharge capacities of electrodes with different contents of Co3O4 are reported in Table 1. The maximum discharge capacities of electrodes with 2%, 4%, 6% and 8% Co3O4 are 317.3, 330, 337.3, 343.7 mA?h/g-1, respectively, which are higher than the electrode with no Co3O4 by 0.83%, 4.86%, 7.18% and 9.21%, accordingly.
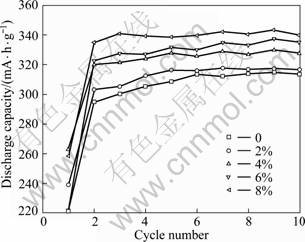
Fig. 1 Discharge capacity of hydrogen alloy electrodes with different contents of Co3O4 additive in 10 cycles
Table 1 Maximum discharge capacity of hydrogen storage alloy electrode with different contents of Co3O4
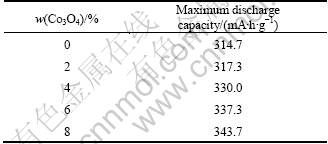
Figure 2 presents charge-discharge curves of hydrogen storage alloy electrodes with different contents of Co3O4 at the 15th cycle. All electrodes show a characteristic of good charge-discharge platform voltage, while the discharge curves of the electrodes with Co3O4 present a second discharge platform around 1.15 V, indicating the occurrence of another electrode reaction. The higher the content of Co3O4 added in electrode is, the more obvious the second discharge platform and the higher platform voltage are. XIA et al [15] thought that the cobalt oxide which was put in the hydrogen storage alloy electrode can form the oxygen cycle in the course of over charge and discharge, which led to the formation of the second discharge plateau to increase discharge capacity of hydrogen storage alloy electrode.
The exchange current density (J0) and polarization resistance (Rp) are used to evaluate electrocatalytic activity for charge-transfer reaction on the surface of the hydride alloy electrode. The higher exchange current density always means a better electrocatalytic activity of charge-transfer reaction. When the over-potential is within 20 mV around the equilibrium potential, the polarization current and over-potential of the electrode are approximate to a linear function, and it can be expressed as [16]

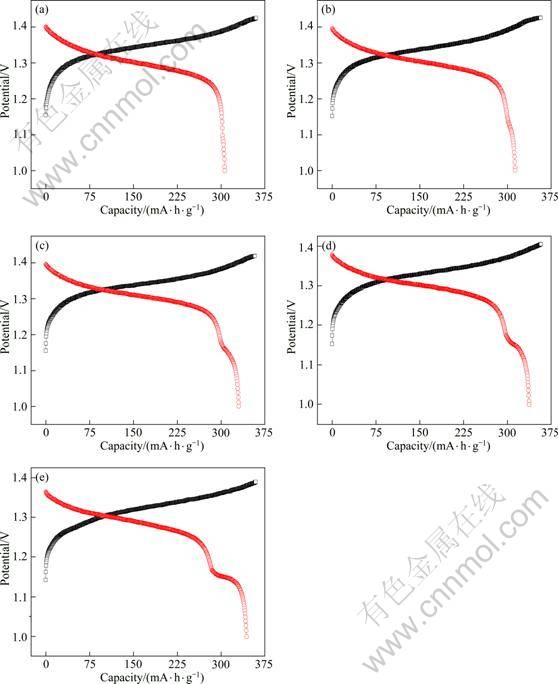
Fig. 2 Charge-discharge curves of hydrogen storage alloy electrodes at the 15th cycle with different contents of Co3O4: (a) Without Co3O4; (b) 2%; (c) 4%; (d) 6%; (e) 8%
Rp can be obtained from the slop of linear polarization curves and written as

Thus we can rewrite the formula as

where n is the number of electron in the reaction; F is the Faraday constant; R is the gas constant; T is the thermodynamic temperature; I(t) is the polarization current; h(t) is the over-potential. Rp is the polarization resistance and J0 is the exchange current density.
Figure 3 displays the linear polarization curves of hydrogen storage alloy electrodes with different contents of Co3O4. The results calculated by the above formula are listed in Table 2. It is clear that the Rp of electrodes reduces obviously and J0 increases significantly with the addition of Co3O4, suggesting that Co3O4 is beneficial to decreasing polarization and increasing the electrocatalytic activity of hydrogen storage alloy electrode. However, Rp gradually increases and J0 decreases with increasing the content of Co3O4. This is due to the fact that Co3O4 is a semiconductor, resulting in a poor conductivity of the hydrogen storage alloy electrode.
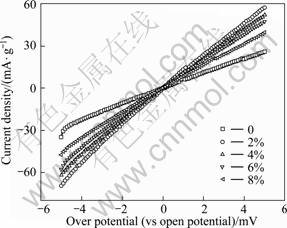
Fig. 3 Linear polarization curves of hydrogen storage alloy electrodes with different contents of Co3O4 additive
Table 2 Rp and J0 of hydrogen storage alloy electrodes with different contents of Co3O4
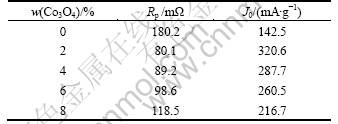
Figure 4 exhibits EIS diagrams of the alloy electrodes with different contents of Co3O4 at 50% DOD. From Fig. 5, all the EIS curves consist of a semi-circle in high frequency region, a semi-circle in high-medium frequency region and a straight line in low frequency region, which are ascribed to the contact resistance between the current collector and the alloy electrode pellet, the charge-transfer reaction resistance (Rct) and the Warburg resistance, respectively [17]. Rct of electrodes with Co3O4 decreases rapidly as compared with the electrode without Co3O4. While Rct gradually increases with increasing the content of Co3O4, which is consistent with the results of linear polarization.
3.2 Electrode process analysis
The primary electrochemical reaction occurring at hydrogen storage alloy electrode in alkaline electrolyte is

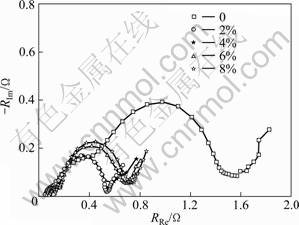
Fig. 4 Electrochemical impedance spectra of hydrogen storage alloy electrodes with different contents of Co3O4
Figure 5 (a) shows the cyclic voltammograms of hydrogen storage alloy electrodes with different contents of Co3O4 after 10 charge-discharge cycles. Among all the cyclic voltammograms, there are two peaks located at -0.65 V in anodic branch and -0.90 V in cathodic branch, which are attributed to the oxidation of adsorbed hydrogen on the electrode surface and hydrogen evolution reaction, respectively. With increasing the content of Co3O4, the anodic current at -0.65 V increases slightly and the cathodic current of -0.90 V is also enhanced. Furthermore, the cathodic peak potential moves towards a negative direction, indicating that the reaction irreversibility reduces to a certain extent. Another anodic peak near -0.75 V is also observed and the peak current increases obviously with increasing the content of Co3O4. It is well established that the peak at -0.75 V corresponds to the reaction of Co to Co2+, and its cathodic peak coincides with hydrogen evolution reaction [18]. It can be concluded that Co3O4 dissolves partly in alkaline electrolyte through electrochemical reaction and forms a reversible oxidation-reduction reaction of Co to Co (OH)2 during charge-discharge process. And the probable mechanism could be expressed as
Co3O4+H2O+OH-→3CoOOH+e;
CoOOH+H2O+e→Co(OH)2+OH-;
Co(OH)2+2e
Co+2OH-
The reactions not only increase the discharge capacity of hydrogen storage alloy electrode, but also decrease polarization resistance and charge reaction resistance.
Figure 5 (b) gives CV curves of hydrogen storage alloy electrodes with different contents of Co3O4 after the 50th charge-discharge cycle. Peak current, especially around the peak of -0.75 V in anodic branch, exhibits much higher than that of the oxidation of adsorbed hydrogen reaction. While the potential remained essentially unchanged. It is suggested that with increasing the charge-discharge cycle, the reaction of Co2+ to Co plays a more and more important role in the electrode reaction.
Figure 6 shows the SEM image and EDS of electrode without Co3O4 and with 8% Co3O4 after 50 charge-discharge cycles. We can see that the surface of electrode with 8% Co3O4 is rougher than that without Co3O4. Element contents of electrode surface are listed in Table 3. The Co content on electrode surface with 8% Co3O4 is higher than that without Co3O4 by 0.6%, revealing that the electrochemical dissolved reaction of Co3O4 has happened.
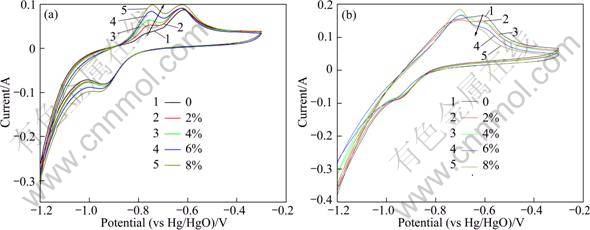
Fig. 5 CV curves of hydrogen storage alloy electrodes with different contents of Co3O4 after different charge-discharge cycles: (a) 10 cycles; (b) 50 cycles
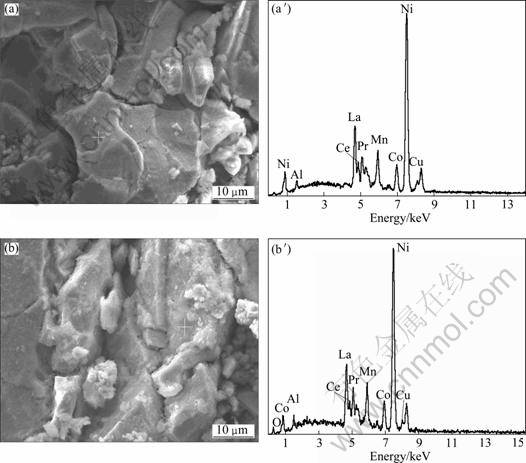
Fig. 6 SEM images (a, b) and EDS (a′, b′) of electrode: (a), (a′) without Co3O4+; (b), (b′) 8% Co3O4
Table 3 Element contents of electrode surface
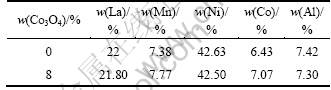
4 Conclusions
1) The discharge capacity of hydrogen storage alloy electrodes with Co3O4 is much higher than that without Co3O4. With increasing the content of Co3O4, discharge capacity of electrodes increases.
2) A second discharge platform around 1.15 V in the electrodes with Co3O4 is observed. The higher the content of Co3O4 added in electrode is, the more obvious the second discharge platform and the higher platform voltage are.
3) The electrocatalytic activity, polarization resistance and charge-reaction resistance of electrodes with Co3O4 additive are improved obviously. But with increasing the content of Co3O4, Rp and Rct gradually increase and J0 decreases. The reason is that Co3O4 as a kind of semiconductor decreases the conductivity of the hydrogen storage alloy electrode.
4) The improved electrochemical performance of electrodes with Co3O4 is for the reason that Co3O4 can partly dissolve and form a reversible oxidation-reduction reaction of Co to Co(OH)2.
References
[1] SAKAI T, MIYAMURA H, KURIYAMA N, KATO A, OGURO K, ISHIKAWA H. Metal hydride anodes for nickel-hydrogen secondary battery [J]. Journal of the Electrochemical Society, 1990, 137(3): 795-799.
[2] PAN Hong-ge. Influence of Co content on kinetic properties of MlNi4.3-xCoxAl0.7 hydrogen storage alloys [J]. The Chinese Journal of Nonferrous Metals, 1999, 9(3): 453-457. (in Chinese)
[3] AYARI M, PAUL-BONCOUR V, LAMLOUMI J, MATHLOUTHI H, PERCHERON-GUEGAN A. Study of the structural, thermodynamic and electrochemical properties of LaNi3.55Mn0.4Al0.3(Co1-xFex)0.75 (0≤x≤1) compounds used as negative electrode in Ni-MH batteries [J]. Journal of Alloys and Compounds, 2006, 420(1-2): 251-255.
[4] CUSCUETA D J, MELNICHUK M, PERETTI H A, SALVA H R, GHILARDUCCI A A. Magnesium influence in the electrochemical properties of La-Ni base alloy for Ni-MH batteries [J]. International Journal of Hydrogen Energy, 2008, 33(13): 3566-3570.
[5] BEN MOUSSA M, ABDELLAOUI M, KHALDI C, MATHLOUTHI H, LAMLOUMI J, PERCHERON G A. Effect of substitution of Mm for La on the electrochemical properties of the LaNi3.55Mn0.4Al0.3Co0.75 compound [J]. Journal of Alloys and Compounds, 2005, 399(1-2): 264-269.
[6] ANANTH M V, RAJU M, MANIMARAN K, BALACHANDRAN G, LEKSHMI M N. Influence of rare earth content on Mm-based AB5 metal hydride alloys for Ni-MH batteries—An X-ray fluorescence study [J]. Journal of Power Sources, 2007, 167(1): 228-233.
[7] ZHAO Xiang-yu, MA Li-qun, DING Yi, SHEN Xiao-dong. Effect of particle size on the electrochemical properties of MmNi3.8Co0.75Mn0.4Al0.2 hydrogen storage alloy [J]. International Journal of Hydrogen Energy, 2009, 34(8): 3389-3394.
[8] ZHANG Yang-huan, WANG Guo-qing, DONG Xiao-ping, GUO Shi-hai, WANG Xin-lin. Effect of rapid quenching on the microstructures and electrochemical performances of Co-free AB5-type hydrogen storage alloys [J]. International Journal of Hydrogen Energy, 2005, 30(10): 1091-1098.
[9] ZHAO Xiang-yu, DING Yi, YANG Meng, MA Li-qun. Effect of surface treatment on electrochemical properties of MmNi3.8Co0.75Mn0.4Al0.2 hydrogen storage alloy [J]. International Journal of Hydrogen Energy, 2008, 33(1): 81-86.
[10] HUANG Bing, SHI Peng-fei, LIANG Zhen-chen, CHEN Meng, GUAN Yun-fei. Effects of sintering on the performance of hydrogen storage alloy electrode for high-power Ni/MH batteries [J]. Journal of Alloys and Compounds, 2005, 394(1-2): 303-307.
[11] ZHANG Peng, WEI Xue-dong, LIU Yong-ning, ZHU Jie-wu, YU Guang. Effects of metal oxides addition on the performance of La1.3CaMg0.7Ni9 hydrogen storage alloy [J]. International Journal of Hydrogen Energy, 2008, 33(4): 1304-1309.
[12] ZHANG Sen, SHI Peng-fei, DENG Chao. Characteristics of hydrogen storage alloy electrode with cupric oxide additive [J]. Solid State Ionics, 2006, 177(13-14): 1193-1197.
[13] GUO Xian-feng, LV Dong-sheng, ZHOU Liu, LI Wei-shan. Study on performances of hydrOgen storage alloy modified with V2O5 [J]. Rare Metal Materials and Engineering, 2007, 36(7): 1287-1290. (in Chinese)
[14] SU Zhen-hua, LIU Kai-yu, ZHANG Si-fang, LI Ao-sheng. Effect of CoO on the electrochemical properties of rare earth based hydrogen storage alloy [J]. Rare Metal Materials and Engineering, 2008, 37(6): 1004-1007. (in Chinese)
[15] XIA Bao-jia, YIN Ge-ping, CHENG Xin-qun, LI Jian-ling. Regulation of the discharge storage capacity of negative electrodes in MH/Ni batteries [J]. Battery Bimonthly, 2000, 30(2): 59-61. (in Chinese)
[16] NOTTEN P H L, HOKKELING P. Double-phase hydride forming compounds a new class of highly electrocatalytic materials [J]. Journal of the Electrochemical Society, 1991, 138(7): 1877-1885.
[17] KURIYAMA N, SAKAI T, MIYAMURA H, UEHARA I, ISHIKAWA H, IWASAKI T. Electrochemical impedance spectra and deterioration mechanism of metal hydride electrodes [J]. Journal of the Electrochemical Society, 1992, 139(7): 72-73.
[18] IWAKURA C, MATSUOKA M, KOHNO T. Mixing effect of metal oxides on negative electrode reactions in the nickel-hydride battery [J]. Journal of the Electrochemical Society, 1994, 141(9): 2306-2309.
AB5型贮氢合金电极添加Co3O4的电化学性能
张青青1, 苏 耿2, 李傲生1, 刘开宇1
1. 中南大学 化学化工学院,长沙 410083;2. 中南大学 粉末冶金国家重点实验室,长沙 410083
摘 要:将不同含量的Co3O4(2%,4%,6%,8%,质量分数)作为添加剂加入到储氢合金中,采用机械混合法进行改性处理。对添加Co3O4的合金电极的电化学性能和电极过程进行研究。结果表明:放电容量有了较大增加,添加2%、4%、6%、8% Co3O4的电极放电容量比空白电极容量分别增加0.83%, 4.86%, 7.18% 和 9.21%。线性极化曲线和电化学阻抗谱测试表明,添加Co3O4降低了电极的电荷转移电阻。循环伏安、扫描电镜和EDS测试表明,添加的Co3O4可部分溶解,发生Co-Co(OH)2可逆氧化-还原反应,从而改善储氢合金的电化学性能。
关键词:Co3O4;储氢合金;容量;电化学性能
(Edited by LI Xiang-qun)
Foundation item: Projects (21071153, 20976198) supported by the National Natural Science Foundation of China
Corresponding author: LIU Kai-yu; Tel: +86-731-88830886; E-mail: kaiyuliu@263.net
DOI: 10.1016/S1003-6326(11)60877-7