
Synthesis and characterization of metallic Pd embedded TiO2 nanoparticles by reverse micelle and sol-gel processing
Jeoung-Ho JIN1, Min-Cheol CHU2, Seong-Jai CHO2, Dong-Sik BAE1
1. School of Nano & Advanced Materials Science Engineering, Changwon National University,
Gyeongnam, 641-773, Korea;
2. Division of Industrial Metrology, Korea Research Institute of Standards and Science, 1 Doryong-Dong,
Yuseong-Gu, Daejeon, 305-340, Korea
Received 2 March 2009; accepted 30 May 2009
Abstract: Metallic Pd clusters were embedded into TiO2 nanoparticles that were synthesized within reverse micelle via a solution reduction of Pd(NO3)2 by hydrazine hydrate. The size of the particles can be controlled by manipulating the relative rates of the hydrolysis and condensation reactions of titanium tetra-isopropoxide within the micro-emulsion. The spherical equivalent size for the Pd clusters formed in TiO2 matrix was estimated to be around (3±1) nm. The presence of Pd in the matrix was demonstrated by EDS spectroscopy. The effects of synthesis parameters, such as the concentration of Pd solution, the molar ratio of water to TIP, and the molar ratio of water to surfactant, were discussed.
Key words: catalyst; nanomaterial; powder technology; sol-gel preparation
1 Introduction
Recently, the synthesis of nanometer-sized particles of metals, semiconductors and metal oxides has been investigated extensively because of their novel electrical, optical, magnetic, and chemical properties[1-10]. The effect of particle size on the electrical and optical properties of these nanosized particles during the growth of the crystallite from the molecular level to the bulk material is an area of fundamental interest[1]. Numerous approaches have been explored for the preparation of spherical nanosized particles, including the use of colloids, polymers, glasses, and micelles to successfully control aggregation[2-4]. Many new and unusual physical and chemical properties also arise as particles attain nanosized dimensions[5-6]. Compared with conventional solid-state reaction methods, solution-based synthesis results in higher levels of chemical homogeneity. Also, mixing of the starting materials at the molecular level is achieved in solution-based system, which is especially important when multi-component oxides are being prepared. In addition, surface coating or surface modification of nanometer semiconductor and metal particles offers a new challenge to synthesis. Not only metal/silica nanocomposites, but also semiconductor/oxide and even semiconductor/insulator/ metal multiple-layer heterostructures can be prepared using this method [9].
There has been intense study of TiO2 as a photo catalyst because of its high chemical stability, nontoxicity, low cost, and excellent degradation for organic pollutants[11-15]. However, the large band gap and low quantum yields of TiO2 prevent it from practical applications. Noble metals, such as Ag, Au, Pt, and Pd deposited on a TiO2 surface, could enhance the photo catalytic efficiencies because they act as an electron trap promoting interfacial charge transfer processes in the composite systems[16-19]. But this type of catalyst structure, though effective, results in exposing noble metal to reactants and the surrounding medium[20]. Metals on the surface of the semiconductor will be easily corroded and dissolved. An efficient way to overcome these drawbacks is to exploit a core–shell-type structure in which the noble metal particles are introduced as cores, and the semiconductor dioxide such as TiO2 and SiO2 act as shells[21-24].
The object of this study is to prepare Pd clusters that were embedded into a TiO2 nanoparticles by a combined reverse micelle and sol-gel technique.
2 Experimental
Typically, microemulsions of total volume of 20 mL were prepared at ambient temperature in a 30 mL vial with rapid stirring, and they consisted of 4 mL Igepal, 10 mL cyclohexane, 0.65-1.32 mL 2×10-2 mol/L Pd(NO3)2×xH2O solution, and deionized water. The size of the resultant particles was controlled by the molar ratio of water to surfactant. The microemulsion was mixed rapidly, and after 5 min of equilibration, one drop (~0.05 mL) of hydrazine hydrate (9 mol/L N2H4×xH2O, Aldrich Chemical Co.) was added as a reducing agent. After nanosized water droplets were formed while stirring, TIP was added into the stirred microemulsion. NH4OH was injected into the microemulsion to accelerate the condensation reaction of metal alkoxide precursors. Reverse micelles were prepared from nonionic surfactant which was poly(oxyethylene) nonylphenyl ether (Igepal CO-520, Aldrich Chemical Co.). The surfactant was used without further purification. Other chemicals, such as titanium isopropoxide(TIP, Aldrich Chemical Co.), cyclohexane, and NH4OH (29%) (all from Fisher Scientific) were used as received. The structure, size and morphology of the resulted composites were examined by transmission electron microscope(TEM). For TEM studies, samples were prepared by adding drops of freshly prepared cluster solution on a carbon film supported on a Cu grid.
3 Results and discussion
Metallic Pd clusters were embedded into TiO2 nanoparticles that were obtained in reverse micelles followed by in situ hydrolysis and condensation in the microemulsion. The average size of the cluster was found to depend on the micelle size, the nature of the solvent, and the concentration of reagent. The morphologies of the Pd embedded TiO2 nanoparticles are illustrated in Fig.1. The distinct contrast change under bright field mode indicates insertion of Pd species. The dark areas in particle aggregates reflect a Pd-rich TiO2 particulate matrix while the gray areas are more similar to pure TiO2 aggregates. The average size of the synthesized Pd embedded TiO2 particles did not change with increasing water-to-surfactant molar ratio. The spherical equivalent size for the Pd clusters formed in TiO2 matrix is estimated to be around (3±1) nm. The nucleation and growth of palladium particles is likely to be a diffusion-controlled process through interaction between micelles, but it can be influenced by many other factors such as phase behavior and solubility, average occupancy of reacting species of the aqueous pool, and the dynamic behavior of the microemulsion.
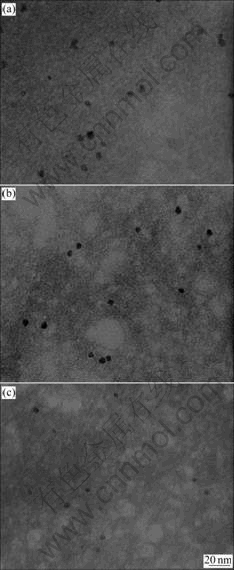
Fig.1 TEM micrographs of synthesized Pd embedded TiO2 nanoparticle by reverse micelle and sol-gel processing as function of R at x=1 and H=100: (a) R=4; (b) R=6; (c) R=8
Fig.2 shows that water-to-TIP molar ratio(H) influenced the particle sizes and distribution of the synthesized Pd embedded TiO2 nanoparticles. The average size of the synthesized Pd embedded TiO2 nanoparticles slightly changed with increasing H at R=4. The chemical composition analysis performed by EDS revealed the successfully embedding of Pd in TiO2 matrix (Fig.3).
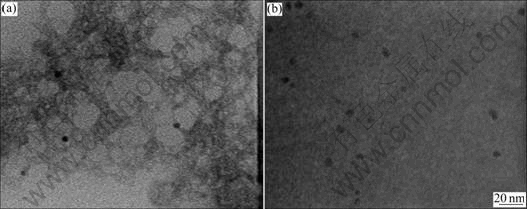
Fig.2 TEM micrographs of synthesized Pd embedded TiO2 nanoparticle by reverse micelle and sol-gel processing as function of H at x=1 and R=4: (a) H=100; (b) H=200
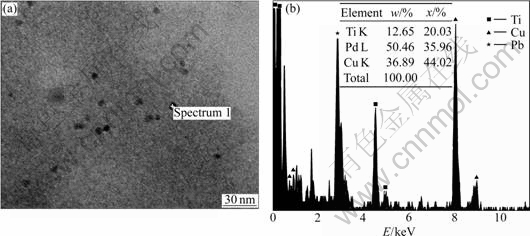
Fig.3 TEM micrographs and EDS spectrum of Pd embedded TiO2 nanoparticle by reverse micelle and sol-gel processing at R=4, H=50, and x=1
4 Conclusions
Metallic Pd clusters were embedded into a TiO2 nanoparticles that were synthesized within reverse micelle via a solution reduction of Pd(NO3)2 by hydrazine hydrate. The spherical equivalent size for the Pd clusters formed in TiO2 matrix was estimated to be around (3±1) nm. The average size of the synthesized metallic Pd clusters embedded TiO2 nanoparticles did not change with increasing water-to-surfactant molar ratio. The average size of the synthesized Pd embedded TiO2 nanoparticles slightly changed with increasing water-to- TIP molar ratio at R=4. The chemical composition analysis performed by EDS revealed the successfully embedding of Pd in TiO2 matrix. TEM studies of particle formation indicate that the reaction process in the complex system containing reverse micelles and TIP is governed by a diffusion-controlled process.
Acknowledgement
This research was financially supported by the Ministry of Education, Science Technology(MEST) and Korea Industrial Technology Foundation(KOTEF) through the Human Resource Training Project for Regional Innovation.
References
[1] POOL R. Science, 1990, 248: 1186-1189.
[2] WANG Y, HERRON N. Nanometer-sized semiconductor clusters: Materials synthesis, quantum size effects, and photophysical properties [J]. J Phys Chem, 1991, 95(2): 525-532.
[3] TRICOT Y M, FENDLER J H. In situ generated colloidal semiconductor CdS particles in dihexadecyl phosphate vesicles: Quantum size and asymmetry effects [J]. J Phys Chem, 1986, 90(15): 3369-3374.
[4] BORELLI N F, HALL D W, HOLLAND J H, SMITH W D. Photoluminescence and relaxation dynamics of cadmium sulfide superclusters in zeolites [J]. J Phys Chem, 1988, 92: 4988-4994.
[5] WANG Y, MAHLER W. Degenerate four-wave mixing of CdS/polymer composite [J]. Opt Comm, 1987, 61: 233-236.
[6] ICHINOSE N. Introduction to fine ceramics [M]. New York: Wiley, 1987.
[7] ICHINOSE N, OZAKI Y, KASHU S. Superfine particle technology [M]. New York: Springer-Verlag, 1988.
[8] ADAIR J H, LI T, KIDO T, HAVEY K, MOON J, MECHOLSKY J, MORRONE A, TALHAM D R, LUDWIG M H, WANG L. Recent developments in the preparation and properties of nanometer-size spherical and platelet-shaped particles and composite particles [J]. Mater Sci Eng R, 1998, 23(4/5): 139-242.
[9] LI T, MOON J, MORRONE A A, MECHOLSKY J J, TALHAM D R, ADAIR J H. Preparation of Ag/SiO2 nanosize composites by a reverse micelle and sol-gel technique [J]. Langmuir, 1999, 15: 4328- 4334.
[10] OSSEO-ASARE K, ARRIAGADA F J. Preparation of SiO2 nanoparticles in a non-ionic reverse micellar system [J]. Colloids and Surfaces, 1990, 50: 321-339.
[11] FUJISHIMA A, RAO TATA N, TRYK D A. TiO2 photocatalysts and diamond electrodes [J]. Electrochimica Acta, 2000, 45(28): 4683-4690.
[12] HU C, YU J C, HAO Z, WONG P K. Effects of acidity and inorganic ions on the photocatalytic degradation of different azo dyes [J]. Appl Catal B, 2003, 46: 35-47.
[13] HOFFMANN M R, MARTIN S T, CHOI W, BAHNEMANN D W. Environmental applications of semiconductor photocatalysis [J]. Chem Rev, 1995, 95: 69-96.
[14] YU J C, HO W, YU J, YIP H, WONG P K, ZHAO J. Efficient visible-light-induced photocatalytic disinfection on sulfur-doped nanocrystalline titania [J]. Environ Sci Technol, 2005, 39: 1175.
[15] KAMAT P V. Kinetics of the photo electrochemical evolution of hydrogen at p-type Si [J]. Chem Rev, 1993, 93: 267.
[16] BEHAR D, RABANI J. Kinetics of hydrogen production upon reduction of aqueous TiO2 nanoparticles catalyzed by Pd0, Pt0, or Au0 coatings and an unusual hydrogen abstraction: Steady state and pulse radiolysis study [J]. J Phys Chem B, 2006, 110: 8750.
[17] KIM S K, HWANG S J, CHOI W. Synergetic effects of nitrogen doping and Au loading on enhancing the visible-light photocatalytic activity of nano-TiO2 [J]. J Phys Chem B, 2005, 109: 24260.
[18] LI X Z, LI F B. Study of Au/Au3+-TiO2 photocatalysts toward visible photo oxidation for water and wastewater treatment [J]. Environ Sci Technol, 2001, 35: 2381-2387.
[19] YOU X, CHEN F, ZHANG J, ANPO M. A novel deposition precipitation method for preparation of Ag-loaded titanium dioxide [J]. Catal Lett, 2005, 102: 247-250.
[20] HIRAKAWA T, KAMAT P V. Charge separation and catalytic activity of Ag@TiO2 core-shell composite clusters under UV- irradiation [J]. J Am Chem Soc, 2005, 127: 3928-3934.
[21] TOM R T, NAIR A S, SINGH N, ASLAM M, NAGENDRA C L, PHILIP R, VIJAYAMOHANAN K, PRADEEP T. Freely dispersible Au@TiO2, Au@ZrO2, Ag@TiO2, and Ag@ZrO2 core-shell nanoparticles: One-step synthesis, characterization, spectroscopy, and optical limiting properties [J]. Langmuir, 2003, 19: 3439-3445.
[22] UNG T, LIZ-MARZAN L M, MULVANEY P. Controlled method for silica coating of silver colloids: Influence of coating on the rate of chemical reactions [J]. Langmuir, 1998, 14: 3740-3748.
[23] PASTORIZA-SANTOS I, KOKTYSH. D S, MAMEDOV A A, GIERSIG M, KOTOV N A, LIZ-MARZAN L M. One-pot synthesis of Ag@TiO2 core-shell nanoparticles and their layer-by-layer assembly [J]. Langmuir, 2000, 16: 2731-2735.
[24] CHAN S C, BARTEAU M A. Preparation of highly uniform Ag/TiO2 and Au/TiO2 supported nanoparticle catalysts by photodeposition [J]. Langmuir, 2005, 21: 5588-5595.
Corresponding author: Dong-Sik BAE; Tel: +82-55-279-7625; Fax: +82-55-262-6486; E-mail: dsbae7@changwon.ac.kr
(Edited by YANG Hua)