Trans. Nonferrous Met. Soc. China 24(2014) s134-s140
White luminescence of Ho3+/Tm3+/Yb3+-codoped CaWO4 synthesized via citrate complex route assisted by microwave irradiation
Hyun CHO1, Seok Min HWANG2, Jae Bin LEE2, Dong Hyun KA2, Tae Wan KIM2, Bo Seul LEE2, Ji Young LEE2, Jung-Il LEE2, Jeong Ho RYU2
1. Department of Nanomechatronics Engineering, Pusan National University, 50 Cheonghak-ri, Miryang, Gyeongnam 627-706, Korea;
2. Department of Materials Science and Engineering, Korea National University of Transportation, Chungju, Chungbuk 380-702, Korea
Received 18 June 2013; accepted 15 October 2013
Abstract: The nanocrystalline Ho3+/Tm3+/Yb3+ co-doped CaWO4 upconversion (UC) phosphors were successfully synthesized by a modified citrate complex method using microwave irradiation. The citrate complex precursors were heat-treated at temperature ranging from 300 to 700 °C for 3 h. Crystallization of the Ho3+/Tm3+/Yb3+ co-doped CaWO4 was detected at 400 °C, and entirely completed at 600 °C. The Ho3+/Tm3+/Yb3+ co-doped CaWO4 heat-treated at 600 °C showed primarily spherical and homogeneous morphology. Under the laser excitation of 980 nm, Ho3+/Tm3+/Yb3+ co-doped CaWO4 shows the bright white upconversion (UC) emission visible to the naked eye, which is composed of a blue emission at 475 nm from Tm3+, and green and red emissions at 543 and 651 nm respectively from Ho3+. The coordinates of Ho3+/Tm3+/Yb3+ co-doped CaWO4 in the Commission International De’eclairage (CIE) chromaticity diagram could be controlled from a cool to a warm white color depending on the Tm3+ and Ho3+ concentrations. The UC luminescent properties on Tm3+ and Ho3+ concentrations and related mechanism based on laser pump power were discussed in detail.
Key words: upconversion; CaWO4; microwave irradiation; white luminescence
1 Introduction
In recent years, research on upconversion (UC) materials, particularly the lanthanide-doped UC phosphors, has attracted much attention because of their unique properties that can convert low energy such as infrared (IR) radiation to high energy such as visible or UV light [1]. In particular, white UC luminescence has been extensively studied, because white luminescent UC phosphors can replace conventional lighting sources in optical devices and three-dimensional backlighting for color displays [2]. Also, white UC phosphors can be used as bio-medical probes with extended simultaneous detection, a capability unmatched by conventional bio-labeling [3]. For white UC luminescence, Er3+, Tm3+, and Ho3+ ions have been used as luminescent centers because they have unique electronic energy states, suitable for pumping by near infrared (NIR) radiation to generate desired visible emissions such as red, green, and blue colors [4]. In particular, Ho3+ and Tm3+ ions are considered candidates for white UC luminescent centers due to their unique UC properties such as yellow emission combined by green and red emissions in Ho3+ and blue emission from Tm3+ [5].
Displays and illuminations require UC luminescent materials that can emit controllable white colors with high efficiency. Also, these materials should have high chemical, physical, and mechanical stabilities. Although white UC luminescence was achieved with lanthanide- doped fluorides via combinations of blue emission from Tm3+ and green or red from Er3+ under NIR (generally 980 nm) excitation [6], fluorides have low physicochemical stabilities, which have made applications difficult in industry [7]. Therefore, it has become important to find new host materials with high stabilities and UC efficiency. Calcium tungstate (CaWO4) with scheelite structure has been studied as a potential electro optic material because of its high density and stable physicochemical properties compared with other oxide materials [8]. However, the white UC luminescence in Tm3+ and Ho3+-codoped CaWO4 has yet to be researched.
In general, calcium tungstate phosphors were synthesized by conventional solid-state reaction method [9]. This approach usually requires heat-treatment at high temperatures for several hours and subsequent grinding. The grinding process damages the phosphor surfaces, resulting in the loss of emission intensity. In addition, calcium tungstate compounds with inhomogeneous composition might be easily formed because of high vapor pressure of WO3 [10]. It was also shown that phosphors prepared by an wet chemical method have higher uniformity in particle size distribution with good crystallinity and exhibit higher photoluminescence intensity than those prepared by the solid-state reaction [11]. Thus, efforts had been made to develop alternative synthesis methods such as hydro-thermal method or sol-gel process.
Microwave irradiation as a heating source has been found and developed for a number of applications in chemical and ceramic processing [12]. Compared with the usual methods, microwave assisted synthesis has the advantage of shortening the reaction time, giving products with small particle size, narrow particle size distribution, and high purity, which can be attributed to fast homogeneous nucleation and easy dissolution of the gel [13]. In this work, we report the intense white UC luminescence of Tm3+ and Ho3+ co-doped CaWO4 nanoparticle prepared by a citrate complex precursor using microwave irradiation. The precursors and powders are evaluated for the crystallization process, thermal decomposition and particle morphology. The color index of white UC emission is modulated by controlling the blue, green, and red components depending on the doping concentrations of Tm3+ and Ho3+ ions. The effects of Ho3+, Tm3+, and Yb3+ ions on the crystal structure of CaWO4, photoluminescence properties, and possible UC mechanism are discussed in detail.
2 Experimental
2.1 Synthesis of Ho3+/Tm3+/Yb3+-codoped CaWO4 powder
The Ho3+/Tm3+/Yb3+-codoped CaWO4 powders were synthesized by the complex citrate-gel method with microwave treatment. The starting materials were calcium nitrates (Ca(NO3)2·4H2O, 99.99%; Junsei Chemical Co. Ltd., Japan), ammonium paratungstate pentahydrate ((NH4)10W12O41·5H2O, 99.99%; Wako Chemical Co. Ltd., Japan), holmium nitrate hydrate (Ho(NO3)3·5H2O, 99.99%; Alfa aesar), thulium nitrate hydrate (Tm(NO3)3·5H2O, 99.99%; Alfa aesar) and ytterbium nitrate hydrate (Yb(NO3)3·6H2O, 99.99%; Alfa aesar). They were used as the metallic cations and were dissolved in D.I. water with citric acid. The molar ratios of the metallic cations were as follows: (1-x-y-z)Ca2++ xHo3++yTm3++zYb3++W6+; x, y=0, 0.002, 0.005, 0.008, and 0.01; z=0.16. The solution was kept at 100 °C for 10 min under constant stirring until it became viscous. The solution was treated with microwave irradiation for 30 min in ambient atmosphere under constant stirring until the solution became a brown gel. No visible precipitation was observed during the heating process. As this solution condensed, the brown product was converted into powders after grinding with a Teflon bar. Thermal analysis was performed on this powder, hereinafter referred to as the precursor. After the microwave treatment, the obtained gel was heat treated in an oven at 250 °C for 24 h to remove organic substances and to evaporate moisture. Finally, the dried powders were calcined from 300 to 700 °C for 3 h in ambient atmosphere.
2.2 Characterization
The crystallization process of the polymeric precursor was evaluated by thermogravimetry- differential thermal analysis (TG-DTA, SETRAM, France), using a sample mass of about 8 mg and a heating rate of 5 °C/min. The existing phase in the particles after heat-treatment was identified by ordinary X-ray diffraction (XRD, Rigaku, Japan; Cuα, λ=1.5046
) with a scan rate of 3 (°)/min. The microstructure and surface morphology of the nanocrystalline powders were observed by scanning electron microscope (SEM, JSM-35CF, JEOL) and transmission electron microscope (TEM, JEM 2010, JEOL). Room- temperature UC luminescent spectra were evaluated with a photoluminescence spectrophotometer (LS55 with 100 mW laser diode, Perkin Elmer, USA) in the wavelength range from 400 to 750 nm under single-wavelength NIR laser excitation of 980 nm. The pump-power-dependence was calculated for irradiation power from 20 to 110 mW (SPEX, 1404P, France).
3 Results and discussion
3.1 Crystallization and phase evolution
The crystallization process of the precursor was evaluated by XRD, TG-DTA and electronic diffraction pattern (EDP). Figure 1 shows the phase identification of the Ho3+/Tm3+/Yb3+-codoped CaWO4 (0.5Ho3+, 0.5Tm3+ and 16Yb3+; mole fraction) according to heat-treatment temperature from 300 to 700 °C for 3 h using XRD. In Fig. 1(a), the Ho3+/Tm3+/Yb3+-codoped CaWO4 powder heat-treated at 300 °C had amorphous and unreacted phases. Above 400 °C in Figs. 1(b)-(e), the powders could be identified as CaWO4 phases, and unreacted or secondary phases were not detected. The XRD patterns of all the samples were in good agreement with the standard pattern of JCPDS (41-1431), indicating that the Ca2+ ions of CaWO4 were successfully substituted by Ln3+ ions (Ln = Ho3+, Tm3+, and Yb3+) up to 17% (mole fraction) without any charge compensator such as Li+ or Na+ ions. This agreement also means that the charge difference between the doped trivalent ions and bivalent host ions did not affect the CaWO4 crystal structure.
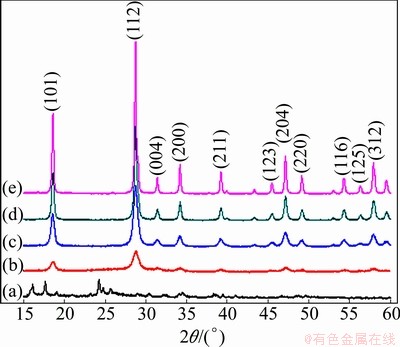
Fig. 1 XRD patterns of Ho3+/Tm3+/Yb3+-codoped CaWO4 (0.5Ho3+, 0.5Tm3+ and 16Yb3+; mole fraction, %) precursors heat-treated at 300 (a), 400 (b), 500 (c), 600 (d) and 700 °C (e) for 3 h
Figure 2 shows the TG-DTA curves for the Ho3+/Tm3+/Yb3+-codoped CaWO4 (0.5Ho3+, 0.5Tm3+ and 16Yb3+) precursor. In Fig. 2, with the increase of temperature, the mass loss occurs in the TG curve up to 500 °C. Thereafter the mass remains constant, indicating that the decomposition of all organic materials continued in the precursor, their combustion and crystallization of CaWO4 have been completed before 500 °C. No significant plateau, corresponding to well-defined intermediate products, appeared in the heating process. The DTA curve in Fig. 2 could be interpreted two physical meanings: 1) increase of DTA curve at 350 °C corresponds to initial decomposition of the precursor and formation of the nucleus of the Ho3+/Tm3+/Yb3+- codoped CaWO4 nanoparticles and 2) exothermic peak at 440 °C corresponds to the crystallization of the Ho3+/Tm3+/Yb3+-codoped CaWO4. Under 350 °C, the resultant particles were dark brown and porous in structure, denoting an amorphous phase. It is attributed to contain a lot of carbons and ignitable organics. When the temperature further increases above 350 °C, crystal nuclei begin to form, and consequently the primary crystallization process is completed accompanying the combustion of the residual carbons and ignitable organics.
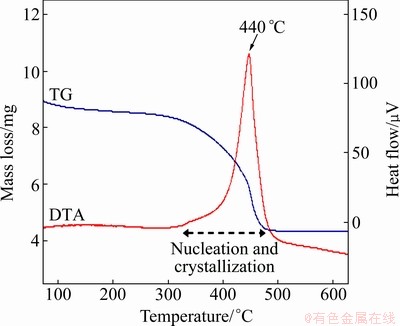
Fig. 2 TG-DTA curves of Ho3+/Tm3+/Yb3+-codoped CaWO4 precursor in flowing air
Figure 3(a) shows TEM and electronic diffraction pattern (EDP) of the Ho3+/Tm3+/Yb3+-codoped CaWO4 (0.5Ho3+, 0.5Tm3+ and 16Yb3+; mole fraction, %) powder heat-treated at 600 °C. The EDP of the powder sample heat-treated at 300 °C showed only diffuse hollow rings, corresponding to an amorphous phase. With increasing temperature, at 400 °C dotted rings were observed, signifying the nanocrystalline particle formation. The Ho3+/Tm3+/Yb3+-codoped CaWO4 (0.5 Ho3+, 0.5 Tm3+ and 16 Yb3+; mole fraction, %) heat-treated at 600 °C showed primarily spherical as shown in Fig 3(a). Figure 3(b) shows a surface morphology of the Ho3+/Tm3+/Yb3+- codoped CaWO4 powder heat-treated at 600 °C using SEM. The Ho3+/Tm3+/Yb3+-codoped CaWO4 particles heat-treated at 600 °C show spherical and agglomerated particles of 30-50 nm, which corresponds to the TEM result of Fig. 3(a).
3.2 Upconversion and white luminescence
Figure 4 illustrates the upconversion (UC) luminescence of Ho3+/Tm3+/Yb3+-codoped CaWO4 heat- treated at 600 °C for 3 h and the variations of emission intensities with Tm3+/Ho3+ concentration under excitation at 980 nm. As presented in Figs. 4(a) and 4(b), the Tm3+/Yb3+-codoped CaWO4 has a strong blue emission peak near 470 nm induced by 1G4 → 3H6 transition with a weak red emission peak near 650 nm induced by 3F2 → 3H6 transition [14]. Moreover, the Ho3+/Yb3+-codoped CaWO4 shows green (540 nm) and red (650 nm) emission peaks generated by 4F4, 5S2 → 5I8 and 5F5 → 5I8 transitions, respectively. The UC luminescence appears yellowish due to a combination of green and red emissions from Ho3+ [15]. At higher ratios of concentration between two dopants (Tm3+/Ho3+), the intensity of blue emission is higher than that of the green or red emission. However, as the Ho3+ concentration increases, the relative intensities of the green and red emission components increase. Therefore, the relative intensities of the blue, green, and red components can be controlled by modulating the Ho3+ and Tm3+ concentrations to display multicolor and white lighting.
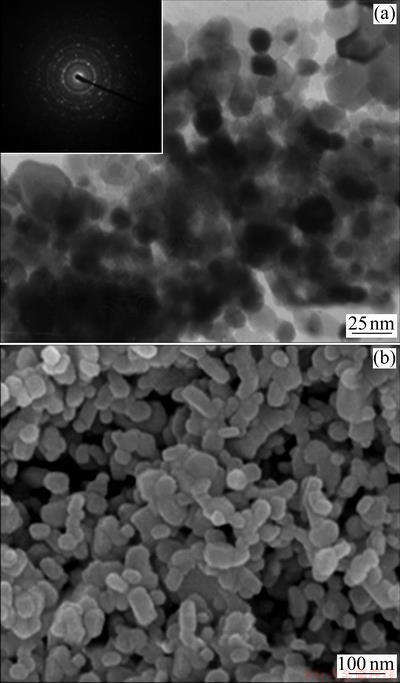
Fig. 3 TEM (a) and SEM (b) micrographs of nanocrystalline Ho3+/Tm3+/Yb3+-codoped CaWO4 (0.5Ho3+, 0.5Tm3+ and 16Yb3+; mole fraction, %) heat-treated at 600 °C for 3 h
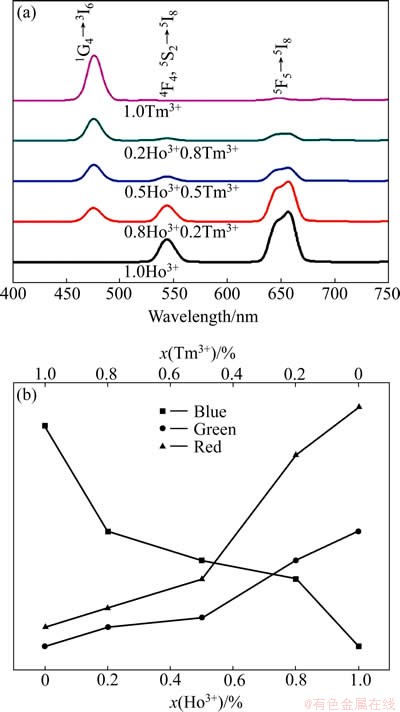
Fig. 4 UC luminescence of Ho3+/Tm3+/Yb3+-codoped CaWO4 in 400-750 nm (a), variations of emission intensities with Tm3+/Ho3+ concentrations under excitation at 980 nm (b)
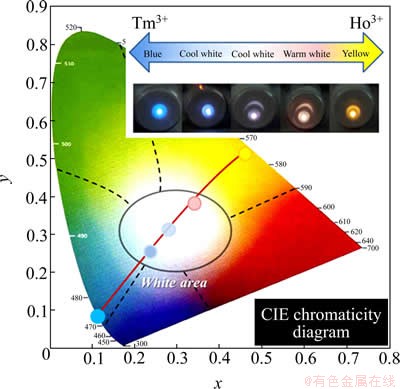
Fig. 5 CIE chromaticity diagram and digital photographs (inset) of Tm3+/Ho3+/Yb3+-codoped CaWO4 according to Ho3+ and Tm3+ concentrations
Figure 5 shows commission international De’eclairage (CIE) chromaticity diagrams and the digital photographs of Ho3+/Tm3+/Yb3+-codoped CaWO4 heat- treated at 600 °C for 3 h. As shown in Fig. 5, the combination of RGB colors from Tm3+ and Ho3+ ions can generate a wide range of white emissions by modulating the Ho3+ and Tm3+ concentrations. The chromaticity coordinates (x, y) are obviously changed from the cool white to the warm white region as shown in the inset of Fig. 5. Tm3+/Yb3+-codoped CaWO4 shows bright blue UC emission. The corresponding chromaticity coordinates are x=0.116 and y=0.082. However, 0.8% Tm3+/0.2% Ho3+-codoped CaWO4 produces cool white UC emission plotted at x=0.255 and y=0.247. Moreover, with increasing ratio of Ho3+ to Tm3+, UC emissions gradually move to warm white emission at 0.2% Tm3+/ 0.8% Ho3+ (x=0.368, y=0.0.371). Finally, Ho3+/Yb3+- codoped CaWO4 shows bright yellow UC luminescence plotted at x=0.445, y=0.530. These results mean that a wide range of white UC emissions can be achieved by Ho3+/Tm3+/Yb3+-codoped CaWO4 by controlling the relative intensities of the blue, green, and red components according to the different Ho3+ and Tm3+ concentrations.
Figure 6(a) shows the logarithmically plotted UC emission intensities of CaWO4 codoped with 0.5%Ho3+/ 0.5%Tm3+/16%Yb3+ as a function of laser pump power. For the unsaturated UC process, the number of photons required to populate the upper energy state can be explained using the following relation [16]:
I ∝ Pn (1)
where n is the number of pumping photons required to excite the upper emitting state, I is the UC luminescent intensity, and P is the laser pumping power. The calculated slopes (n) were 2.80, 1.75, and 1.70 for blue, green, and red emissions, respectively. These results mean that the UC mechanism of blue emission can be populated by the three-photon process, while those of the green and red emissions by the two-photon process [17].
Based on this result, the possible UC emissions and populating mechanisms in Ho3+/Tm3+/Yb3+-codoped CaWO4 are schematically presented in Fig. 6(b). For blue emission from Tm3+, nonresonant energy transfer (ET) occurs from the 2F5/2 state in the Yb3+ to the 3H5 level in the Tm3+, and/or the ground state absorption (GSA) process can be attributed to the 3H6 → 3H5 transition. Subsequent 3H5 → 3F4 transition can be generated by phonon-assisted nonradiative transition. Then, the 3F3 state can be excited from the 3F4 state via the excited state absorption (ESA) or energy transfer (ET), which can generate the 3H4 state from the nonradiative transition that plays an important role in blue emission. The 3H4 state is further excited to the 1G4 state through the ESA or ET process. Lastly, the 1G4→3H6 transition generates the blue emission at around 470 nm [18].
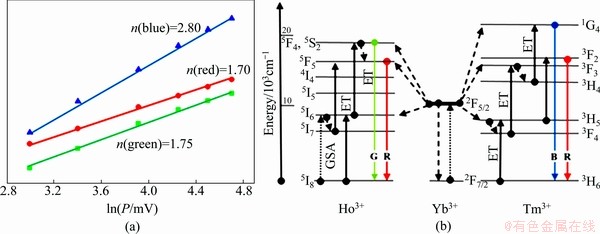
Fig. 6 Effect of pump-power-dependent emission on working current from 20 to 110 mW for green, red, and blue UC emissions (a), and possible UC mechanism under excitation at 980 nm (b)
Moreover, in the case of green and red emissions of Ho3+, the ground state absorption (GSA) (5I8→5I6) and energy transfer (ET) of 2F5/2 (Yb3+)+5I8 (Ho3+)→ 2F7/2(Yb3+)+5I6 (Er3+) are responsible for the population at 5I6 level in Ho3+. For the green emission, the 5I6 state in Ho3+ involves two possible processes: 1) ESA: 5I6 + a photon (980 nm) → 5F4/5S2 and/or 2) ET: 2F5/2 (Yb3+) + 5I6 (Ho3+) → 2F7/2 (Yb3+) + 5F4/5S2 (Ho3+). The two possible processes generate the 5F4/5S2 state of Ho3+, which then relaxes to the ground state at 5I8 of Ho3+. As a result, the process produces green emission near 540 nm corresponding to 5F4/5S2 → 5I8 transitions [19]. In red emission, there are two possible UC mechanisms. First, the red emission is initiated through the population of the 5I6 state. The 5I6 state can be populated through ET and GSA processes from the 5I8 state to the 5I6 state. The relaxation of photons from the 5I6 state to the 5I7 state results in a nonradiative process. Then, the 5F5 state can be populated through the 5I7 → 5F5 transition via the ESA or ET process from the 5F5/2 (Yb3+) + 5I7 (Ho3+) →5F7/2 (Yb3+)+5F5 (Ho3+) transition. In the second process for the red emission, the 5F5 state can be populated by the nonradiative transition from 5F4/ 5S2 → 5F5. Finally, the radiative process by relaxation from the 5F5 state to the ground state of 5I8 generates the red emission around 650 nm [20, 21].
4 Conclusions
1) The nanocrystalline Ho3+/Tm3+/Yb3+-codoped CaWO4 powders were successfully synthesized by a modified citrate complex method using microwave irradiation. Crystallization of the Ho3+/Tm3+/Yb3+- codoped CaWO4 was detected at 400 °C, and entirely completed at 600 °C.
2) Controllable white UC luminescence was successfully achieved from Ho3+/Tm3+/Yb3+-codoped CaWO4. The blue, green, and red emissions as components of white UC emission can be controlled by modulating the Ho3+ and Tm3+ concentrations.
3) The calculated CIE coordinates can be located in the white region and can be changed from the cool to the warm white region. Therefore, it is concluded that Ho3+/Tm3+/Yb3+-codoped CaWO4 can be a promising UC phosphor as a white light source for next-generation display devices and biomedical applications.
Acknowledgement
The research was supported by a grant from the LINC (Leaders in INdustry-university Cooperation) Program of Korea National University of Transportation in 2013.
References
[1] VENNERBERG D, LIN Z. Upconversion nanocrystals: Synthesis, properties, assembly and applications [J]. Science of Advanced Materials, 2011, 3(1): 26-40.
[2] LIU Y, XU C, YANG Q. White upconversion of rare-earth doped ZnO nanocrystals and its dependence on size of crystal particles and content of Yb3+ and Tm3+ [J]. Journal of Applied Physics, 2009, 105: 084701.
[3] CHATTERJEE D K, GNANSAMMANDHAN M K, ZHANG Y. Small upconverting flourescent nanoparticles for biomedical applications [J]. Small, 2010, 6(24): 2781-2795.
[4] WANG F, LIU X. Recent advances in the chemistry of lanthanide-doped upconversion nanocrystals [J]. Chemical Society Reviews, 2009, 38: 976-989.
[5] VETRONE F, MAHALINGAM V, CAPOBIANCO J A. Near-infrared-to-blue upconversion in colloidal BaYF5:Tm3+,Yb3+ nanocrystals [J]. Chemistry of Materials, 2009, 21: 1847-1851.
[6] WANG G, PENG Q, LI Y. Upconversion luminescence of monodisperse CaF2:Yb3+/Er3+ nanocrystals [J]. Journal of the American Chemical Society, 2009, 131: 14200-14201.
[7] LIU Z, PENG R. Inorganic nanomaterials for tumor angiogenesis imaging [J]. European Journal of Nuclear Medicine and Molecular Imaging, 2010, 37(Suppl 1): S147-S163.
[8] RYU J H, YOON J W, LIM C S, OH W C, SHIM K B. Microwave-assisted synthesis of CaMoO4 nano-powders by a citrate complex method and its photoluminescence property [J]. Journal of Alloys and Compounds, 2005, 390: 245-249.
[9] SHI S, GAO J, ZHOU J. Effect of charge compensation on the luminescence behavior of Eu3+ activated CaWO4 phosphor [J]. Optical Materials, 2008, 30: 1616-1620.
[10] BAEK Y, YONG K. Controlled growth and characterization of tungsten oxide nanowires using thermal evaporation of WO3 powder [J]. The Journal of Physical Chemistry C, 2007, 111: 1213-1218.
[11] KIM K M, RYU J H, MHIN S W, PARK G S, SHIM K B. Luminescence of nanocrystalline Tb3Al5O12:Ce3+ phosphors synthesized by nitrate-citrate gel combustion method [J]. Journal of the Electrochemical Society, 2008, 155(10): J293-J296.
[12] BAGHBANZADEH M, CARBONE L, COZZOLI P D, KAPPE C O. Microwave-assisted synthesis of colloidal inorganic nanocrystals [J]. Angewandte Chemie International Edition, 2011, 50: 11312-11359.
[13] RYU J H, YOON J W, SHIM K B. Blue luminescence of nanocrystalline PbWO4 phosphor synthesized via a citrate complex route assisted by microwave irradiation [J]. Solid State Communications, 2005, 133: 657-661.
[14] CHEN Z, BU W, ZHANG N, SHI J. Controlled construction of monodisperse La2(MoO4)3:Yb,Tm microarchitectures with upconversion luminescent property [J]. The Journal of Physical Chemistry C, 2008, 112: 4378-4383.
[15] STOUWDAM J W, VEGGEL F C J M. Near-infrared emission of redispersible Er3+,Nd3+, and Ho3+ doped LaF3 nanoparticles [J]. Nano Letters, 2002, 2(7): 733-737.
[16] WANG J, TANNER P A. Upconversion for white light generation by a single compound [J]. Journal of the American Chemical Society, 2010, 132: 947-949.
[17] POLLNAU M, GAMELIN D R,
H U, HEHLEN M P. Power dependence of upconversion luminescence in lanthanide and transition-metal-ion systems [J]. Physical Review B, 2000, 61(5): 3337-3346.
[18] WANG G, PENG Q, LI Y. Luminescence tuning of upconversion nanocrystals [J]. Chemistry-A European Journal, 2010, 16: 4923-4931.
[19] XU H L,
S. Upconversion dynamics in Er3+-doped YAG [J]. Journal of Luminescence, 2005, 111: 191-198.
[20] HEER S, LEHMANN O, HASSE M,
H U. Blue, green, and red upconversion emission from lanthanide-doped LuPO4 and YbPO4 nanocrystals in a transparent colloidal solution [J]. Angewandte Chemie International Edition, 2003, 42: 3179-3182.
[21] LI L, CAO X Q, ZHANG Y, GUO C X. Synthesis and upconversion luminescence of Lu2O3:Yb3+,Tm3+ nanocrystals [J]. Transactions of Nonferrous Metals Society of China, 2012, 22: 373-379.
微波辐射辅助柠檬酸络合法制备的Ho3+/Tm3+/Yb3+共掺杂CaWO4的白光性能
Hyun CHO1, Seok Min HWANG2, Jae Bin LEE2, Dong Hyun KA2, Tae Wan KIM2, Bo Seul LEE2, Ji Young LEE2, Jung-Il LEE2, Jeong Ho RYU2
1. Department of Nanomechatronics Engineering, Pusan National University, 50 Cheonghak-ri, Miryang, Gyeongnam 627-706, Korea;
2. Department of Materials Science and Engineering, Korea National University of Transportation, Chungju, Chungbuk 380-702, Korea
摘 要:采用微波辐射辅助柠檬酸络合法制备Ho3+/Tm3+/Yb3+共掺杂CaWO4纳米晶升频转换荧光粉。将柠檬酸络合物前驱体在300~700 °C热处理3 h。Ho3+/Tm3+/Yb3+共掺杂CaWO4C在400 °C时开始结晶, 在600 °C时结晶完成。经600 °C热处理的Ho3+/Tm3+/Yb3+共掺杂CaWO4主要呈球形,且形态均匀。在980 nm的激光激发下, Ho3+/Tm3+/Yb3+共掺杂CaWO4纳米晶出现肉眼可见的明亮的白色升频转换发射, 这种现象来自Tm3+的475 nm蓝光发射以及Ho3+的543 nm绿光和651 nm红光发射。通过调整Tm3+和Ho3+的浓度可以控制Ho3+/Tm3+/Yb3+共掺杂CaWO4的CIE色度图从冷到暖白色之间变化。讨论了Tm3+和Ho3+浓度对升频转换光性能的影响以及与激光泵功率相关的影响机制。
关键词:升频转换;CaWO4;波辐射;白色发光
(Edited by Hua YANG)
Corresponding author: Jeong Ho RYU; Tel: +82-43-841-5384; E-mail: jhryu@ut.ac.kr
DOI: 10.1016/S1003-6326(14)63300-8