
Preparation of continuous Si-Fe-C-O functional ceramic fibers
CHEN Zhi-yan(陈志彦)1, LI Xiao-dong(李效东)2, WANG Jun(王 军)2, LI Wen-fang(李文芳)1
1. School of Mechanical Engineering, South China University of Technology, Guangzhou 510640, China;
2. Key Laboratory of Ceramic Fibers and Composites, National University of Defense Technology,Changsha 410073, China
Received 16 January 2007; accepted 27 March 2007
Abstract: A new polymer named polyferrocarbosilane(PFCS) was prepared from polydimethylsilane and ferrocene. The spinnability of this polymer can be tailored by controlling the content of ferrocene in the polymer. The prepared polymer was spun into a continuous polymer fiber that was subsequently cured in air and heat-treated finally in N2 up to 1 350 ℃ for conversion into Si-Fe-C-O fibers. The resulted Si-Fe-C-O fibers display low specific resistance and magnetic property due to the existence of Fe, which also reduces the specific resistance significantly to 10-2 Ω?cm at room temperature when the amount of ferrocene in feed is as low as 3.0% (mass fraction). The resulted Si-Fe-C-O fibers, with C/Si molar ratio of about 1.3 and the maximum Fe content of about 2.0% (mole fraction), are composed of β-SiC and small amount of Fe3Si-like crystalline and have an average tensile strength of about 2.0 GPa.
Key words: continuous Si-Fe-C-O fiber; SiC fiber; polyferrocarbosilane; ferrocene; electromagnetic properties
1 Introduction
It is well known that silicon carbide fiber prepared by precursor conversion method is one of the most promising fibers for many high-technology composites because of the high tensile strength, high elastic module and good oxidation resistance at high temperature. It is interesting to study the electromagnetic properties of the fiber when it is applied as functional materials. Since SiC fiber derived from polycarbosilane(PCS) generally has rather high specific resistance and no magnetic properties [1], the modification of the electromagnetic properties of SiC fiber with hetero atoms in PCS has been very important in many applications and attracts much attention of many researchers.
Polytitanacarbosilane(PTCS) with 1.5%-4% of Ti was synthesized from polydimethylsilane and titanium tetraalkoxide by YAMAMURA et al[2], and low specific resistance (10-1 Ω?cm) Si-Ti-C-O fibers (Tyranno Lox[3] (trade name) by Ube Industries, Japan) were produced from PTCS through melt-spinning, curing and pyrolysis. With a similar procedure, Si-Zr-C-O fibers[4-5] (with about 2% Zr) were prepared with polyzirconocarbosilane (PZCS) and Si-Al-C-O fibers[5-7] (with about 1% Al) from polyaluminacarbosilane(PACS) were manufactured by Ube with trade names Tyranno-ZE and Tyranno-SA respectively. The hetero atoms in both Si-Zr-C-O and Si-Al-C-O fibers mentioned above play an important role in inhibition of crystalline grain of β-SiC at very high temperature and thus improve the high temperature resistance. It was reported that materials made from silicon carbide fibers containing Ti or Zr exhibited a reflection attenuation of 40 dB at 10 GHz and may be applied as electromagnetic wave absorbing materials[3].
In addition, SiC single fibers with small amount of iron, cobalt and nickel were prepared by physical mixing of the nano-metal particles with PCS solution by ultra-sonic processing followed by melt-spinning, curing and pyrolysis. These fibers show electromagnetic wave absorbing properties because of the existence of magnetic particles[8].
In this study, Fe contained pre-ceramic polymer, polyferrocarbosilane(PFCS), is synthesized by chemical method, which exhibits a good spinnability, and is converted to magnetic Si-Fe-C-O continuous fibers successfully by high temperature pyrolysis in inert atmosphere. The structural materials that show excellent radar-wave absorbing properties are prepared with this fibers and resin[9].
2 Experimental
Polydimethylsilane(PDMS) was synthesized by dechlorination of dimethaldichlorosilane with sodium in xylene as previous report[10-11]. The synthesized PDMS was rearranged at 420 ℃ under atmosphere of N2 to produce viscous liquid with some Si—C backbond and some Si—Si groups. Then the viscous liquid was mixed with ferrocene and reacted at 400 ℃ for 10 h. PFCS was obtained through dissolving in xylene, filtration and distillation. Continuous Si-Fe-C-O fibers (Fig.1) were prepared by melt-spinning, curing in air and pyrolysis at 1 350 ℃ in N2.
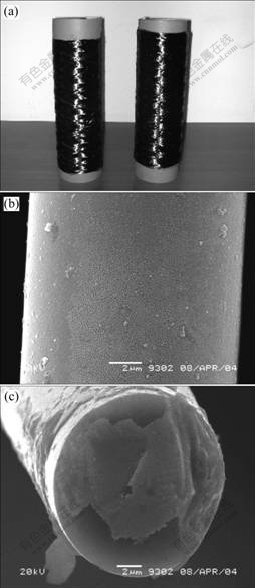
Fig.1 Appearance of continuous Si-Fe-C-O fibers (a), SEM micrographs of Si-Fe-C-O fibers (b), (c)
The relative molecular mass and distribution of PFCS were characterized with Waters-1515 liquid chromatogram machine by Gel Permeation Chromatography(GPC) and tetrahydrofuran(THF) eluent at a flow rate of 1 mL/min at room temperature using polystyrene as standard.
The XRD patterns were recorded on a D8 advance X-ray diffractometer (Cu Kα radiation, λ=0.154 18 nm). XPS semi-quantitative analysis of the surface of Si-Fe-C-O fibers was performed with an X-ray photoelectron spectroscopy apparatus (PHI-5702, American physics electron, Al Kα) under ultrahigh vacuum (4.53×10-7Pa). The tensile strength of the fibers was measured using YG001 monofilament strength electron machine (25 mm in gauge length at room temperature). The specific resistance of Si-Fe-C-O fiber was measured with 4329 high-resistance apparatus at ambient temperature. The magnetic properties were characterized by TM-VSM 2050HGC at room temperature.
3 Results and discussion
3.1 Relative molecular mass of PFCS
The relative molecular mass of PFCS is listed in Table 1, compared with PCS (ferrocene in feed is 0). The relative molecular mass, especially Mm, of PFCS increases greatly with the adding of ferrocene in feed under the same reaction conditions. When the amount of ferrocene is up to 10%(mass fraction) or more, the reaction system becomes an insoluble solid. This is evidential that ferrocene is actually a cross-linker connecting the polymer chains.
Table 1 Effect of ferrocene in feed on relative molecular mass of PFCS
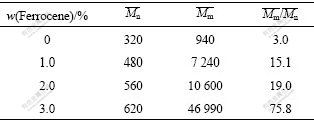
3.2 Composition and structure of PFCS
The IR spectra of PFCS with different amount of ferrocene in feed are shown in Fig.2, where PFCS-n and PCS stand for n% and 0% ferrocene respectively. All the samples show a similar structure of PCS with absorption bands of C—H stretching (2 950 and 2 900 cm-1), Si—H stretching (2 100 cm-1), CH3 deformation in Si—CH3 (1 400 cm-1), CH2 deformation in Si—CH2—Si (1 350 cm-1), CH2 wagging in Si—CH2—Si (1 020 cm-1), and Si—CH3 rocking/Si—C stretching (820 cm-1)[10,12]. However, the Si—H bond becomes lower with the increasing of ferrocene. In the case of high ferrocene specimen, such as PFCS-10, some small characteristic absorbing bands of η5-Cp in ferrocene emerge at 3 088, 1 106 and 490 cm-1[13]. Thus, PFCS can be assumed to take the following general structure with a much smaller than b.
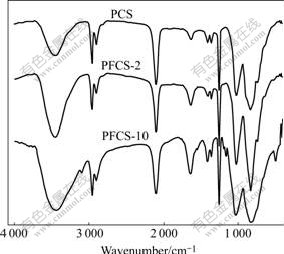
Fig.2 FT-IR spectra of PCS and PFCS
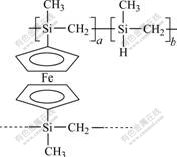
The results of the chemical analysis of PFCS are shown in Table 2, where the content of Fe gradually increases with the amount of ferrocene added, which is quantitatively in accordance with the above structure. It is interesting to find that the ratio of C/Si of PFCS is generally 2.0 or a little higher, while in the case of PCS, the ratio is significantly less than 2.0, as mentioned in Refs.[10, 12, 14]. This can be explained that in the rearrangement reaction from PDMS (C/Si=2) to PCS without ferrocene, the degradation of methyl group and hydrogen free radicals occurs simultaneously to form volatile small molecules and the evaporation of large amount of CH4 leads to a lower C/Si ratio. In the presence of ferrocene, however, the degradation reaction seems less important and the resulted PFCS has a higher C/Si ratio. In practice, one can find that ferrocene also acts as a catalyst of the rearrangement reaction because it makes the reaction time much shorter even at lower temperature.
Table 2 Elemental composition of PFCS (mass fraction, %)
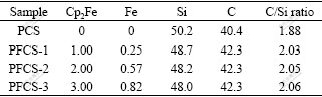
3.3 XPS analysis
The XPS depth elementary profiles of continuous Si-Fe-C-O-3 fibers (Fig.3) show that a carbon-enriched layer of about 100 nm in depth is formed on the surface, the C/Si mole ratio is about 1.3 in the fiber core, and O and Fe molar fractions are about 15% and 2% respectively, and there are some Fe3Si phases in the fibers. The fluctuation of the elementary composition may be due to the heterogenious distribution of small amount of Fe atoms.
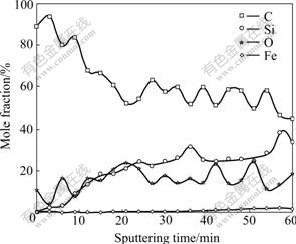
Fig.3 Distribution of elements in diametric direction of continuous Si-Fe-C-O fiber by XPS depth analysis (Etching rate 15 nm/min, etching degree 45?)
3.4 XRD analysis
From the XRD patterns of Si-Fe-C-O fibers (Fig.4), it is obvious that higher amount of ferrocene in PFCS or the content of Fe in the fibers leads to the sharper diffrac- tion peaks. The crystalline grain sizes are estimated, using Scherrer equation from the (111) peak of β-SiC at 2θ= 35.6?, to be 1.8, 3.1, 3.3, 4.2 and 93.4 nm for Si-C-O, Si-Fe-C-O-1, Si-Fe-C-O-2, Si-Fe-C-O-3 and Si-Fe-C-O- 10(powder) respectively. Therefore, Fe contained in the Si-Fe-C-O fibers seems to accelerate the growth of β-SiC crystalline grain in pyrolysis process. In addition, for the high Fe content sample (Fig.4(a)), some new diffraction peaks at 2θ=45.34?, 66.05? and 83.75? emerge, which may possibly be assigned to Fe3Si as (220), (400) and (422) respectively.
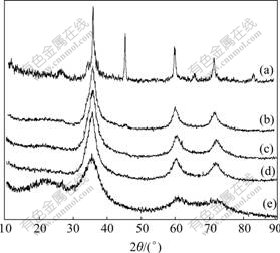
Fig.4 XRD patterns of Si-C-O, Si-Fe-C-O fibers and powder: (a) Si-Fe-C-O-10 (powder); (b) Si-Fe-C-O-3; (c) Si-Fe-C-O-2; (d) Si-Fe-C-O-1; (e) Si-C-O
3.5 Electromagnetic properties of fibers
The specific resistances of Si-Fe-C-O fibers at ambient temperature, listed in Table 3, are much lower than those of the PCS derived SiC fiber (without ferrocene) and Nicalon NL202 (104Ω·cm[15]). Even very small amount of Fe (0.25% in PFCS for 1% ferrocene) plays an important role because of the introduced Si-Fe structure. The more the ferrocene is involved in PFCS, the lower the specific resistances of Si-Fe-C-O fibers are. Fig.5 shows the relationship between the specific resistance of Si-Fe-C-O fibers and the pyrolysis temperature. The trend and magnitude of the curve are similar to those of the PCS derived SiC fibers, demonstrating that the pyrolysis temperature does not change the structure and effect of Si-Fe fundamentally.
Table 3 Properties of continuous Si-Fe-C-O fibers
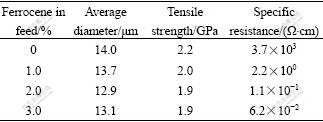
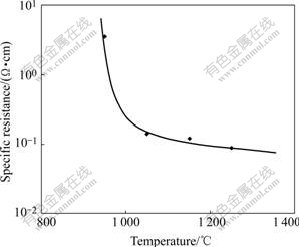
Fig.5 Specific resistance vs pyrolysis temperature of Si-Fe-C-O-2 fiber
Fig.6(a) shows the magnetic hysteresis loop of Si-Fe-C-O-3 fibers whose direction is vertical to that of magnetic field. When the fibers are in parallel direction, a similar loop is produced, but the specific saturation magnetizations are 0.109 and 0.096 A?m2·kg-1, and the coercivities are 1.2×104 and 1.8×104 A/m, for the vertical and parallel direction, respectively. Obviously, Si-Fe-C-O fibers show some magnetic properties and magnetic anisotropy. For higher Fe content of Si-Fe-C-O-10 powder, higher magnetic properties can be expected, as shown in Fig.6(b).
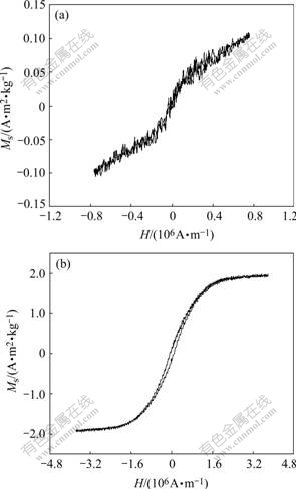
Fig.6 Magnetic hysteresis loops of Si-Fe-C-O-3 fibers (a) and Si-Fe-C-O-10 powder (b)
4 Conclusions
1) Polyferrocarbosilane(PFCS) was synthesized from polydimethylsilane and ferrocene. From PFCS, continuous Si-Fe-C-O fibers were prepared by melt-spinning, curing in air and pyrolysis above 1000℃ in N2.
2) The Si-Fe-C-O fibers show good mechanical properties and low specific resistance.
3) The element Fe in the system is considered to play important roles in cross-linking the PFCS chains, accelerating the growth of β-SiC crystalline grain in pyrolysis, reducing the specific resistance and producing magnetic properties of the Si-Fe-C-O fibers.
References
[1] Nariwa M, ITOI Y, Okamura K. Electrical resistivity of Si-Ti-C-O fibers after rapid heat treatment [J]. Journal of Materials Science, 1995, 30(10): 3401-3406.
[2] Yamamura T, Isgikawa T, Shibuya M. Development of a new Si-Ti-C-O fiber using an organometallic polymer precursor [J]. Journal of Materials Science, 1988, 23: 2589-2594.
[3] Yamamuna T, Toshikawa T, Shibuya M. Electromagnetic wave absorbing material [P]. US 5094907, 1992.
[4] Ishikawa T, Kohtoku Y, Kumagawa K. Production mechanism of polyzirconocarbosilane using zirconium (Ⅳ) acetylacetonate and its conversion of the polymer into inorganic materials [J]. Journal of Materials Science, 1998, 33: 161-166.
[5] Dong S M, Chollon G, Labrugere C. Characterization of nearly stoichiometric SiC ceramic fibres [J]. Journal of Materials Science, 2001, 36: 2371-2381.
[6] Cao F, LI X D, Peng P, Feng C X, Wang J. Structural evolution and associated properties on conversion from Si-C-O-Al ceramic fibers by sintering [J]. Journal of Materials Chemistry, 2002, 12(3): 606-610.
[7] YU Y X, LI X D, CAO F, WANG Y D, ZOU Z C, WANG J, ZHENG C M, ZHAO D F. Preparation and properties of continuous Al-containing silicon carbide fibers [J]. Trans Nonferrous Met Soc China, 2005, 15(3): 510-514.
[8] WANG J, SONG Y C, FENG C X. Pyrolysis of polycarbosilane/ nanoferrum particles [J]. Chinese Journal of Materials Research, 1998, 12(2): 195-198. (in Chinese)
[9] CHEN Z Y, WANG J, LI X D, WANG Y D. Study on preparation of continuous Si-Fe-C-O radar-wave absorbing fibers by precursor method [J]. Journal of Aeronautical Materials, 2006, 26(2): 33-36. (in Chinese)
[10] Yajima S, Hasegawa Y, Hayashi J. Synthesis of continuous silicon carbide fiber with high tensile strength and high young’s modulus (Part 1) [J]. Journal of Materials Science, 1978, 13: 2569-2576.
[11] Soraru G D, Babonneau F, Mackenzie J D. Structural evolutions from polycarbosilane to SiC ceramic [J]. Journal of Materials Science, 1990, 25: 3886-3893.
[12] Hasegawa Y. Synthesis of continuous silicon carbide fibre (Part 3) [J]. Journal of Materials Science, 1983, 18: 3633-3648.
[13] YU Z, ZHOU X G, BAI Z P, ZHOU Y, LIU R C. Mossbauer spectroscopy by using transmission integral evaluation technique: A application for the studies of polyferrocene [J]. Journal of Inorganic Chemistry, 1997, 13(1): 38-42. (in Chinese)
[14] CHENG X Z, XIAO J Y, XIE Z F, SONG Y C, WANG Y D. Composition and structure characterization of polycarbosilane synthesized from polydimethylsilane under high pressure [J]. Journal of National University of Defense Technology, 2005, 27(2): 20-23. (in Chinese)
[15] Ishikawa T. Recent developments of the SiC fiber Nicalon and its composites, including properties of the SiC fiber Hi-Nicalon for ultra-high temperature [J]. Composites Science and Technology, 1994, 51: 551-554.
Corresponding author: CHEN Zhi-yan; Tel: +86-13360034319; E-mail: spchen@163.com
(Edited by YUAN Sai-qian)