
J. Cent. South Univ. (2018) 25: 1642-1650
DOI: https://doi.org/10.1007/s11771-018-3856-y

Photo oxidation of DBT using carbon nanotube titania composite as visible light active photo catalyst
BARMALA Molood1, BEHNOOD Mohammad2, OMIDKHAH Mohammad Reza3
1. Chemical Engineering Department, Dezful Branch, Islamic Azad University, Azadegan Highway,P.O. Box : 313, Dezful, Iran;
2. Department of Petroleum and Chemical Engineering, Science and Research Branch, Islamic Azad University, Tehran, Iran;
3. Chemical Engineering Department, Tarbiat Modares University, Jalal Ale Ahmad Highway,P.O. Box: 14155-143, Tehran, Iran
Central South University Press and Springer-Verlag GmbH Germany, part of Springer Nature 2018
Abstract: Sulfur removal from liquid fuels has increased in importance in recent years. Although hydrodesulfurization is the usual method for removing sulfur, the elimination of thiophene compounds using this process is difficult. Photocatalysis is an alternative method being developed for thiophene removal at ambient conditions. Among semiconductors, titania has shown good potential as a photo-catalyst; however, quick recombination of electron holes hinders its commercial use. One way to decrease the recombination rate is to combine carbon nanotubes with a semiconductor. In this work, multiwall carbon nanotube (MWCNT) / titania composites were prepared with different mass ratios of MWCNT to titania using tetraethyl orthotitanate (TEOT) and titanium tetra isopropoxide (TTIP) as precursors of titania. Dibenzothiophene (DBT) photocatalytic removal from n-hexane was measured in both the presence and absence of oxygen. The results indicated that the best removal occurred when the MWCNT to titania ratio was 1. When the ratio exceeded this number, DBT removal efficiency decreased due to light scattering. Also, the composites prepared by TEOT exhibited better efficiency in DBT removal. The research findings suggested that the obtained composite was a visible light active photocatalyst and exhibited better performance in the presence of oxygen. Kinetics of photocatalytic DBT removal was a first-order reaction with removal rate constant 0.7 h–1 obtained at optimum conditions.
Key words: advanced oxidation processes; carbon nanotube; photocatalysis; UV; kinetics; semiconductor; sol-gel process
Cite this article as: BARMALA Molood, BEHNOOD Mohammad, OMIDKHAH Mohammad Reza. Photo oxidation of DBT using carbon nanotube titania composite as visible light active photo catalyst [J]. Journal of Central South University, 2018, 25(6): 1642–1650. DOI: https://doi.org/10.1007/s11771-018-3856-y.
1 Introduction
The United States Environmental Protection Agency has imposed new rules since 2006 for allowable concentration of sulfur in fuels. Accordingly, the permitted sulfur level of 1.5×10–5 became effective for diesel fuels [1]. Sulfur content in fuels leads to poisoning of the catalysts of desulfurization units. Fuels with sulfur level >0.1% cause emissions of sulfur dioxide (SO2) into the environment, which is associated with acidity upon precipitation and aggravates conditions such as asthma in humans [1–7].
Most sulfuric compounds of diesel get converted to H2S during hydrodesulfurization reaction in the presence of cobalt/molybdenum (CoMo) or nickel/molybdenum (NiMo) catalysts [8, 9]. Benzothiophene and its derivatives naturally exist in crude oil compounds and are the most resistant sulfuric compounds to sulfur removal procedures. Unfortunately, conventional hydro processes are not capable of removing them[6, 8, 10].
To remove thiophenes in such processes, reactors that are 5–15 times greater than the current ones are required [11]. An increase in the process temperature could result in reduced catalyst life [2, 7]. Therefore, hydrodesulfurization seems not to be a proper solution to meet the new standards.
Oxidation processes convert sulfur compounds to sulfone and sulfoxide at room temperature. The resulting compound can be simply isolated by extraction, distillation, or absorption procedures [3, 6, 12].
Researchers are increasingly focused on the use of photocatalysts in removal procedures of various pollutant particles. The photocatalyst is a catalyst that must be exposed to light to alter the rate of a chemical reaction [13]. If a semiconductor (such as TiO2, ZnO and WO3) is exposed to a light beam with band gap energy (the energy gap between the valence band and the conduction band) or more, and electrons are promoted from the valence band to the conduction band forming electron-hole pairs. Therefore, reduction-oxidation (redox) reaction may occur through electron-hole pairs [13, 14].
Titania is one of the most useful semiconductors with band gap around 3.2 eV. Its anatase phase can be activated under beam light with wavelength shorter than 387 nm. Meanwhile, this area absorbs only 4% of solar radiation that reaches the earth, whereas the visible band accounts for 45% [13, 15]. That is why many efforts have been directed to developing visible-light-active (VLA) photocatalysts using substrates such as nanotubes, activated carbon, or doping compounds such as nitrogen [10, 14].
There are different ways of producing multiwall carbon nanotube (MWCNT)/titania composites. One of these methods is known as the sol-gel procedure in which nanotube particles are dispersed in titania sol and increase electron transfer area by increasing the wettability of nanotube [16]. The ultimate features of particles can be affected by titania precursor. JITIANU et al [17] found that different titania precursors in combination with nanotubes can be applied to forming film-coating or titania particles on nanotube surfaces. As they did not mention the difference between the photocatalytic features of these composites, the current study was conducted to compare the photocatalytic power of the composite made by various precursors containing different ratios of MWCNT: titania for Dibenzothiophene (DBT) removal from n-hexane. The effect of ambient oxygen on the reaction was also measured.
MWCNTs were coated with titania using a sol-gel method, as shown in Figure 1. This composite was used as a VLA photo catalyst and the ability of DBT photooxidation by this composite was investigated under different conditions.
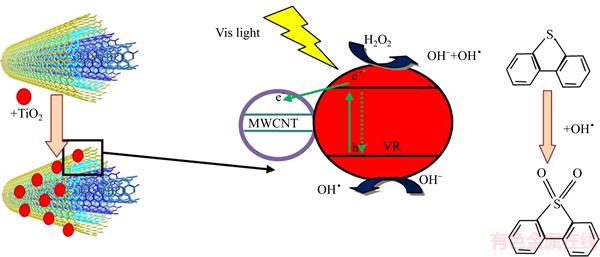
Figure 1 MWCNT/titania composite as a visible light active photo catalyst for DBT photo oxidation
2 Materials and methods
2.1 Preparation and characterization of photocatalysts
MWCNT/titania composite was prepared by the sol-gel method and the molar ratio was as used by JITIANU et al [17]. Tetraethyl orthotitanate (TEOT) and titanium tetra isopropoxide (TTIP) (with 98% purity produced by Merck Co.) were used as titania precursors. To synthesize the samples, titania precursors were dissolved in isopropanol (obtained from Chemical Industry Co. of Dr. Mojalali) with molar ratio 1 to 70. To stabilize the pH level, required amount of nitric acid solution with pH=3 was added and the mixture was stirred for 1 h using a magnetic stirrer during sol preparation. Thereafter, MWCNT was added to the mixture and was stirred for another 3 h. The nanotube ratio was selected in such a way that the mass ratios of MWCNT to titania in the resultant composite were equal to 2.6, 1.3, 1, 0.65, 0.33, 0.2, and 0. The samples were then labeled samples 2–8. The raw MWCNT was considered as Sample 1.
After this step, until the gel formed and dried, the obtained mixture was kept at room temperature. Then, the samples were placed in a furnace and heated at 5 °C/min to reach 450 °C. The samples were calcined for 1 h at this temperature. They were then cooled at room temperature with the same pace.
2.2 Photocatalytic oxidation test
To assess the photocatalytic powers of the composites, 0.5 g of catalyst was added to 100 mL n-hexane solution with initial DBT concentration of 2×10–4. The liquid was then stirred for 2 h in a dark box until adsorption equilibrium was established.
In the next step, the mixture was exposed to a 9 W UV lamp in a mirror box. DBT concentration changes with time were measured using a spectrophotometer. To understand the catalyst structure, BET and SEM tests were conducted.
3 Results
3.1 Influence of titania precursor and MWCNT: titania ratio on adsorption ability
In the first part, the effects of titania precursor and MWCNT: titania ratio on DBT adsorption capacity were measured using the composites.
As indicated in Figure 2, with increasing ratio of MWCNT: titania, the composite’s adsorption power increased (ultimate concentration decreased). This occurred due to the high specific surface area of nanotubes. As reported by other researchers [3, 10, 16, 18], BET test results (Table 1) also indicated that increasing the amount of MWCNT in the composite led to higher specific surface area and increased adsorption power. According to T-test, changing the precursor in a fixed proportion of MWCNT to titania made no significant difference to the composite’s adsorption capacity.
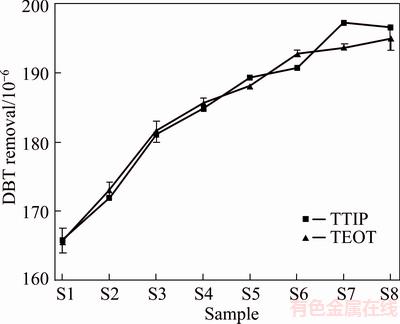
Figure 2 DBT concentration reduction by adsorption
Table 1 SBET of sample with TEOT as precursor
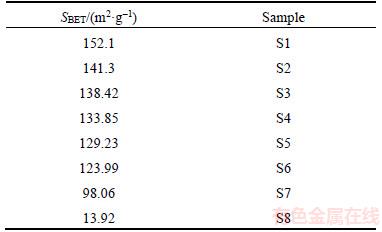
3.2 Influence of titania precursor and MWCNT: titania ratio on photooxidation of DBT
Figure 3 indicates the reduced concentration of DBT caused by UV radiation for 2 h. The figure indicates that:
1) Due to high absorption capacity of the electron, MWCNT had photocatalytic potential [12, 19, 20].
2) Adding MWCNT to the composite increased the photocatalytic power, which reduced the possibility of electron and hole recombination.
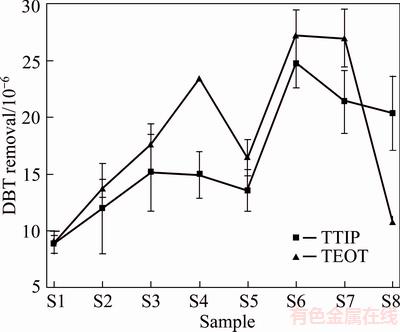
Figure 3 Open cup photooxidation of DBT using composites with different precursors
The higher ratios resulted in light scattering in the solution; though, it inversely affected the photocatalytic removal rate [3, 10, 16, 19–22].
3) In both graphs (esp. TEOT), there are two maxima. This proves that during photocatalytic removal reaction two factors affected the removal rate: adsorption of the pollutant on the surface of the composite and its oxidation rate. With large quantities of MWCNT (sample 4), the removal capacity increased due to high adsorption capacity of the composite, whereas with lower amounts of MWCNT (sample 6), a local maximum appeared as a result of the composite’s high potential for oxidation. The results suggested that there was a significant difference between the photocatalytic powers of composites containing two different precursors (Sample 4: P-value=0.03, Sample 6: P-value=0.06).
Despite the higher removal capacity of TEOT, no significant difference was witnessed between the two maxima that could be attributed to the higher specific area (Sample 4 and Sample 6, P-value= 0.33).
4) In the absence of MWCNT (Sample 8), the removal capacity of the sample produced with TTIP was twice that of the sample produced with TEOT and the difference between the two samples was significant (P-value=0.04). SIMONSEN et al [23] obtained similar results in his research; the result that he attributed to the higher specific surface area of the sample was produced with TTIP.
5) As reported by BERKI et al [24], adding MWCNT to the composite affected the photocatalytic ability of TEOT far more because the longer alkyl chain of the structure reduced the hydrolysis rate. Therefore, larger particles with lower specific surface area would be produced.
JITINAU’s [17] investigation on the effect of initiator on the morphology of MWCNT/titania composite indicated that TEOT as titania precursor coated the MWCNT surface with a thin film, whereas TTIP produced titania particles on the MWCNT surface. Accordingly, it might be the root cause for the difference in photocatalytic removal rate observed in this study. Because film coating increased the contact area between MWCNT and titania, it made electron transfer between them easier and reduced the recombination rate of electron-hole pairs.
According to the results of previous sections, TEOT was a better precursor than TTIP. SEM images of three different samples produced by TEOT are indicated in Figure 4. YAO et al [25] and TASI et al [26] divided nanotube composites to three groups and considered the homogeneous mixture of titania and MWCNT with approximately equal particle sizes as the best composite. In Figure 4, Sample 4 may represent such a composite. Sample 6 is a type-3 composite (aggregation of titania around nanotube), and Sample 2 is a type-2 composite, i.e., small titania particles embedded in a large number of nanotubes.
3.3 Effect of ambient oxygen
In the following step, the effect of ambient oxygen on the amount of DBT removal was measured. The tests were carried out again in a closed container (with no air) to measure the difference between the open and closed containers. The results are depicted in Figure 5.
Figure 5 clearly indicates that higher photocatalytic power of the composite (sample 4 and 6) showed greater difference between the open and closed states as well as the effect of oxygen on DBT removal. Sample 6, which had the maximum photocatalytic power due to favorable oxidation capacity, showed the greatest difference between the open and closed states.
3.4 Influence of MWCNT: titania ratio on light absorption
DRS test results and Kubelka-Munk charts for Samples 4 and 6 produced with TEOT are depicted in Figures 6(a) and (b). To compare the results, MWCNT graph and p-25 (Titanium (IV) dioxide),titania reference, are indicated in this figure. As the figure shows, the nanotube absorbed less light at wavelength <400 nm, i.e., UV range. However, with increase in wavelength reaching the visible region (between 400 and 700 nm), the adsorption level would increase. Considering that nanotubes were black with known features, these results have been proved [10, 16, 20, 22, 27, 28].
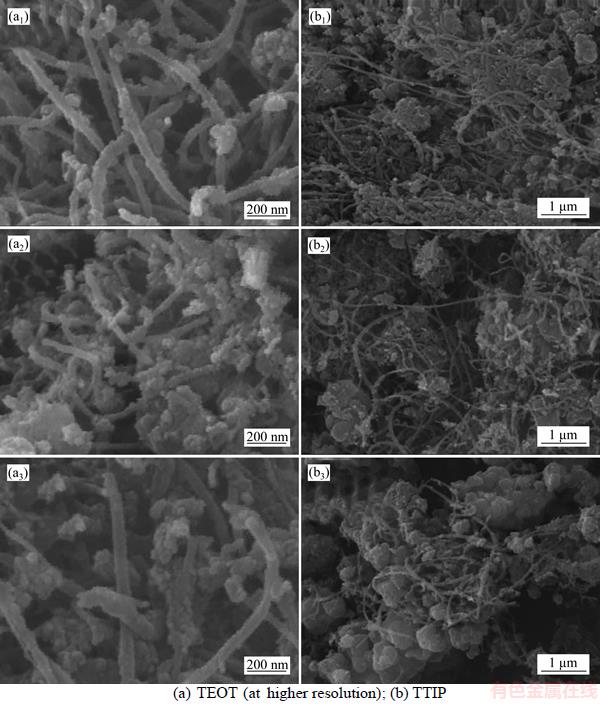
Figure 4 SEM micrographs S1 (a1, b1), S4 (a2, b2) and S6 (a3, b3) with different MWNT: titania ratios:
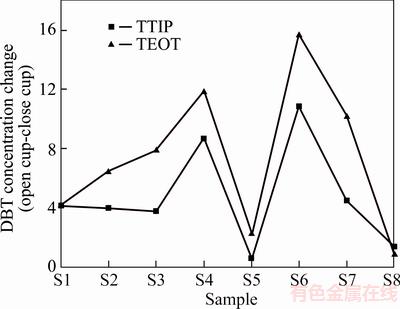
Figure 5 Effect of ambient oxygen (difference between open cup and close cup removal amount) on DBT photooxidation using composites with different precursors
The p-25 sample absorbed light very well at wavelength <400 nm (i.e., UV range) whereas its light absorption in the visible region was very low. This has been clearly indicated by other researchers as well [16, 18, 22].
Light adsorption levels of the other two samples were in between. In this case, due to the presence of nanotube in the particle structure, the light adsorption of the composites increased and reached the visible region while UV adsorption still existed. By combining nanotube and titania, the nanotube produced energy levels above the valence band of titania, which was indicated by a tail in the visible region [10, 16, 20, 22, 27, 28].
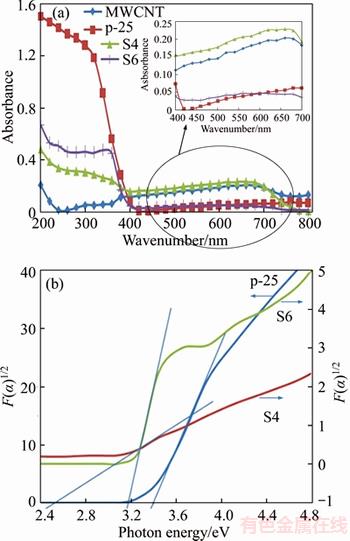
Figure 6 UV-Vis spectra (a) and Kubelka-Munk plots (b) of composites
Comparison of the two samples showed that an increase in the MWCNT: titania ratio in the composite increased the adsorption level in the visible region and decreased UV adsorption. Since the nanotube was black, some parts that were not coated with titania scattered the radiated light and prevented titania particles from adsorbing UV light [16]. The adsorption power of Sample 4 in the visible region was higher than that of the nanotube. This synergistic effect of combining two substances has also been reported by other researchers (titania and silica) [28, 29].
The extrapolation of the linear part of [F(α)]1/2=(αhv)1/2 versus energy of a photon to the abscissa at zero F(α) provides the band gap energies of the samples, where α is the absorbance, hν is the photon energy.
According to Figure 6, the obtained band gap energy of p-25, Samples 4, and 6 were 3.4, 2.53, and 3.2 eV, respectively. The effect of carbon nanotubes: titania ratio on photocatalytic power has been studied by other researchers but usually low numbers of composites or close ratios have been compared with the raw titania and carbon nanotubes. Table 2 shows a summary of this study’s results and those of other researchers.
Table 2 Optimum CNT: titania ratios in other research
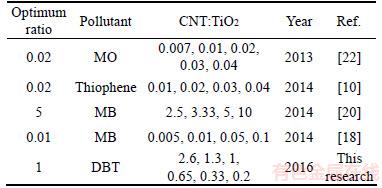
3.5 Kinetics of photo oxidation of DBT
Considering that the obtained composite was a visible light active photocatalyst, the kinetics of the DBT removal reaction under visible light radiation was measured. An adequate amount of hydrogen peroxide (oxidizing agent) was added to the mixture and changes in DBT concentration were observed for 1 h. The results are indicated in Figure 7. DBT removal was a first-order reaction and the composite could remove 48% of DBT in 1 h with reaction rate constant 0.7 h–1.
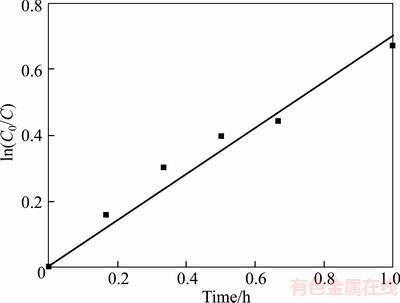
Figure 7 Photooxidation of DBT with composite and H2O2 under visible light irradiation
Based on the data reported in Table 3, the coefficient obtained was 20 times higher than that reported by MATSUZAWA et al [4] for UV radiation on p-25. Other researchers obtained the same order for the reaction rate constant. However, the main difference between this study and previous reports is that in the others UV radiation was investigated while visible light radiation was examined in this case.
Table 3 Reaction rate constant for DBT photooxidation
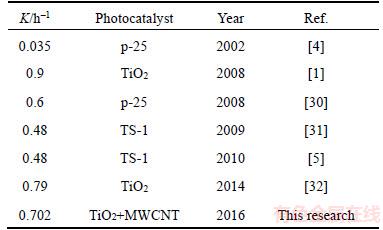
4 Conclusions
MWCNT/titania composites as visible light active photocatalysts were produced by the sol-gel method and DBT photooxidation with visible light was done for the first time. The findings suggest that composites produced with TEOT were more active than those with TTIP and the best MWCNT:titania ratio was 1, which resulted in a significant amount of DBT removal and proper absorption of visible light. It was also concluded that ambient oxygen could participate as an oxidizing agent and increase the removal rate of the reaction. DBT removal was a first-order reaction; the concentration of DBZT decreased to 52% of the initial value after being under visible light of a 9 W lamp for 1 h.
References
[1] LI F T, LIU R H, SUN Z M, ZHAO D S. Photocatalytic oxidation kinetics of thiophene with Nano-TiO2 as photocatalyst [C]// 2nd International Conference on Bioinformatics and Biomedical Engineering. Shanghai, 2008: 3887–3890.
[2] KOWSARI E. New aspects for the future [M]// Recent advances in the science and technology of desulfurization of diesel fuel using ionic liquid, ionic liquid. In TechOpen, 2013.
[3] THI V T H, NGUYEN T T T, NGUYEN Ph H T, DO M H, AU H T, NGUYEN T B, NGUYEN D L, PARK J S. Fabrication of photocatalytic composite of multi-walled carbon nanotubes/TiO2 and its application for desulfurization of diesel [J]. Materials Research Bulletin, 2012, 47(2): 308–314. DOI: 10.1016/j.materresbull.2011. 11.016.
[4] MATSUZAWA S, TANAKA J, SATO S, IBUSUKI T. Photocatalytic oxidation of dibenzothiophenes in acetonitrile using TiO2: Effect of hydrogen peroxide and ultrasound irradiation [J]. Journal of Photochemistry and Photobiology A: Chemistry, 2002, 149(1–3): 183–189. DOI: 10.1016/ S1010-6030(02)00004-7.
[5] ZHANG J, ZHAO D S, YANG L Y, LI Y B. Photocatalytic oxidation dibenzothiophene using TS-1 [J]. Chemical Engineering Journal, 2010, 156(3): 528–531. DOI: 10.1016/ j.cej.2009.04.032.
[6] CAMPOS-MARTIN J M, CAPEL-SANCHEZ M C, PEREZ-PRESAS P, FIERRO J L G. Oxidative processes of desulfurization of liquid fuels [J]. Journal of Chemical Technolology and Biotechnology, 2010, 85(7): 879–890. DOI:10.1002/jctb.2371.
[7] ZENG X Y, WANG G, MO H, ZHOU R C. Oxidation mechanism of dibenzothiophene compounds: A computational study [J]. Computational and Theoretical Chemistry, 2014, 1037: 22–27. DOI: 10.1016/j.comptc. 2014.03.023.
[8] MOOSAVI E S, DASTGHEIB S A, KARIMZADEH R. Adsorption of thiophenic compounds from model diesel fuel using copper and nickel impregnated activated carbons [J]. Energies, 2012, 5(10): 4233–4250. DOI: 10.3390/ en5104233.
[9] MEHDIZADEH A, AHMADI A N, FATEMINASSAB F. Deep desulfurization of fuel diesels using alkyl sulfate and nitrate containing imidazolium as ionic liquids [J]. Journal of Applied Chemical Research, 2013, 7 (1): 75–85. http://www. sid.ir/En/Journal/ViewPaper.aspx?ID=357421.
[10] AAZAM E S. Visible light photocatalytic degradation of thiophene using Ag–TiO2/multi-walled carbon nanotubes nanocomposite [J]. Ceramics International, 2014, 40(5): 6705–6711. DOI: 10.1016/j.ceramint.2013.11.132.
[11] HERNANDEZ-MALDONADO A J, STAMATIS S D, YANG R T. New sorbents for desulfurization of diesel fuels via π-complexation [J]. Separations, 2004, 50(4): 791–801. DOI:10.1021/ie034108.
[12] TAO H, NAKAZATO T, SATO S. Energy-efficient ultra- deep desulfurization of kerosene based on selective photooxidation and adsorption [J]. Fuel, 2009, 88(10): 1961–1969. DOI: https://doi.org/10.1016/j.fuel.2009.03.020.
[13] MUNTER R. Advanced oxidation processes–Current status and prospects. [J]. Proceedings of the Estonian Academy of Sciences: Chemistry, 2001, 50(2): 59–80. http://www.kirj.ee/public/va_ke/k50-2-1.pdf.
[14] PELAEZ M, NOLAN N T, PILLAI S C, SEERY M K, FALARAS P, KONTOS A G, DUNLOP P S M, HAMILTON J W J, BYRNE J A, O’SHEA K, ENTEZARI M H, DIONYSIOU D D. A review on the visible light active titanium dioxide photocatalysts for environmental applications [J]. Applied Catalysis B: Environmental, 2012, 125: 331–349. DOI: 10.1016/j.apcatb. 2012.05.036.
[15] REDDY K R, HASSAN M, GOMES V G. Hybrid nanostructures based on titanium dioxide for enhanced photocatalysis [J]. Applied Catalysis A: General, 2015, 489: 1–16. DOI: 10.1016/j.apcata.2014.10.001.
[16] LI Y J, Li L, Li C, CHEN W, ZENG M. Carbon nanotube/ titania composites prepared by a micro-emulsion method exhibiting improved photocatalytic activity [J]. Applied Catalysis A: General, 2012, 427–428: 1–7. DOI: 10.1016/j.apcata.2012.03.004.
[17] JITIANU A, CACCIAGUERRA T, BENOIT R, DELPEUX S, BEGUIN F, BONNAMY S. Synthesis and characterization of carbon nanotubes–TiO2 nanocomposites [J]. Carbon, 2004, 42 (5, 6): 1147–1151. DOI: 10.1016/ j.carbon.2003.12.041.
[18] KOO Y M, LITTLEJOHN G, COLLINS B, YUN Y, SHANOV V N, SCHULZ M, PAI D, SANKAR J. Synthesis and characterization of Ag-TiO2-CNT nanoparticle composites with high photocatalytic activity under artificial light [J]. Composites, 2014, 57: 105–111. DOI: 10.1016/ j.compositesb.2013.09.004.
[19] SALEH T A. Synthesis and application of carbon nanotubes and their composites [M]. Winchester: InTech Open, 2013: 479–493.
[20] HINTSHO N, PETRIK L, NECHAEV A, TITINCHI S, NDUNGU P. Photo-catalytic activity of titanium dioxide carbon nanotube nano-composites modified with silver and palladium nanoparticles [J]. Applied Catalysis B: Environmental, 2014, 156–157: 273–283. DOI: 10.1016/ j.apcatb.2014.03.021.
[21] ZHU L P, LIAO G H, HUANG W Y, MA L L, YANG Y, YU Y, FU S Y. Preparation, characterization and photocatalytic properties of ZnO-coated multi-walled carbon nanotubes [J]. Materials Science and Engineering B, 2009, 163(3): 194–198. DOI: 10.1016/j.mseb.2009.05.021.
[22] OUYANG K, XIE S, MA X. Photocatalytic activity of TiO2 supported on multi-walled carbon nanotubes under simulated solar irradiation [J]. Ceramics International, 2013, 39(7): 7531–7536. DOI: 10.1016/j.ceramint.2013.03.004.
[23] SIMONSEN M E, SOGAARD E G. Sol-gel reactions of titanium alkoxides and water: Influence of pH and alkoxy group on cluster formation and properties of the resulting products [J]. Journal of Sol-Gel Science and Technology, 2010, 53(3): 485–497. DOI: 10.1007/s10971-009-2121-0.
[24] BERKI P, RETI B, TERZI K, BOUNTAS I, HORVATH E, FEJES D, MAGREZ A, TSAKIROGLU CH, FORRO L, HERNADI K. The effect of titania precursor on the morphology of prepared TiO2/MWCNT nanocomposite materials [J]. Physica Status Solidi B, 2014, 251(12): 2384–2388. DOI:10.1002/pssb.201451161.
[25] YAO Y, LI G, CISTON S, LUEPTOW R M, GRAY K A. Photoreactive TiO2/carbon nanotube composites: Synthesis and reactivity [J]. Environmental Science and Technology, 2008, 42: 4952– 4957.
[26] TSAI Y P, DOONG R, YANG J C, WU Y J. Photo-reduction and adsorption in aqueous Cr(VI) solution by titanium dioxide, carbon nanotubes and their composite [J]. Journal of Chemical Technology and Biotechnology, 2011, 86(7): 949–956. DOI:10.1002/jctb.2605.
[27] LIU H, YU X, YANG H. The integrated photocatalytic removal of SO2 and NO using CU doped titanium dioxide supported by multi-walled carbon nanotubes [J]. Chemical Engineering Journal, 2014, 243: 465–472. DOI: 10.1016/ j.cej.2014.01.020.
[28] LIU H, ZHANG H, YANG H. Photocatalytic removal of nitric oxide by multi-walled carbon nanotubes-supported TiO2 [J]. Chinese Journal of Catalysis, 2014, 35(1): 66–77. DOI: 10.1016/S1872-2067(12)60705-0.
[29] MALI S S, BETTY C A, BHOSALE P, PATIL P S. Synthesis, characterization of hydrothermally grown MWCNT–TiO2 photoelectrodes and their visible light absorption properties [J]. ECS Journal of Solid State Science and Technology, 2012, 1(2): M15–M23. DOI:10.1149/2.004202jss.
[30] VARGAS R, NUNEZ O. The photocatalytic oxidation of dibenzothiophene (DBT) [J]. Journal of Molecular Catalysis A: Chemical, 2008, 294(1, 2): 74–81. DOI: 10.1016/ j.molcata.2008.08.001.
[31] ZHAO D, ZHANG J, WANG J, LIANG W, LI H. Photocatalytic oxidation desulfurization of diesel oil using Ti-containing zeolite [J]. Petroleum Science and Technology, 2009, 27(1): 1–11. DOI: 10.1080/10916460802108314.
[32] DEDUAL G, MACDONALD M J, ALSHAREEF A, WU Z, TSANG D C W, YIP A C K. Requirements for effective photocatalytic oxidative desulfurization of a thiophene- containing solution using TiO2 [J]. Journal of Environmental Chemical Engineering, 2014, 2(4): 1947–1955. DOI: 10.1016/j.jece.2014.08.012.
(Edited by FANG Jing-hua)
中文导读
碳纳米管二氧化钛复合材料作为可见光活性光催化剂对DBT的光氧化
摘要:近年来液体燃料中硫的脱除越来越重要。加氢脱硫是脱除硫的常用方法,但用这种方法消除噻吩类化合物比较困难。光催化法是目前正在开发的一种环境条件下脱除噻吩的替代方法。在半导体中,二氧化钛作为光催化剂显示出了良好的潜力;然而,电子空穴的快速复合阻碍了它的工业应用。降低复合率的一种方法是将碳纳米管与半导体结合起来。本文以正丁酸四乙酯(TEOT)和四异丙醇钛(TTIP)为前驱体,制备了不同质量比的纳米碳管(MWCNT)/二氧化钛复合材料。研究了在有氧和无氧条件下,二苯并噻吩(DBT)对正己烷的光催化去除作用。结果表明,当氧化钛与氧化钛之比为1时,去除效果最好。当比值超过此数时,由于光散射而使DBT去除率降低。TEOT制备的复合材料在DBT去除率方面表现出较好的效果。结果表明该复合材料是一种可见光活性光催化剂,在含氧条件下表现出较好的性能。光催化去除DBT的动力学为一级反应,在最佳条件下得到的去除速率常数为0.7 h–1。
关键词:高级氧化工艺;碳纳米管;光催化;UV;动力学;半导体;溶胶-凝胶法
Received date: 2016-02-26; Accepted date: 2017-10-11
Corresponding author: BARMALA Molood, PhD, Lecturer; Tel: +98–9163024704; E-mail: m.barmala@iaud.ac.ir; ORCID: 0000-0001- 9172-1900