J. Cent. South Univ. Technol. (2008) 15: 814-818
DOI: 10.1007/s11771-008-0150-4

Current efficiency improvement of Zn-Fe alloy electrodeposition by
hydrogen inhibitor
WANG Yun-yan(王云燕), XIAO Hai-juan(肖海娟), CHAI Li-yuan(柴立元)
(School of Metallurgical Science and Engineering, Central South University, Changsha 410083, China)
Abstract: In order to inhibit hydrogen evolution and enhance current efficiency of Zn-Fe alloy electrodeposition from alkaline zincate solution, hydrogen inhibitors composed of the sulfur group elements were optimized on the basis of atom structures analysis. The effects of hydrogen inhibitor on the current efficiency of Zn-Fe alloy electroplating and their electrochemical behaviors were studied. The results indicate that hydrogen inhibitor can increase the current efficiency of Zn-Fe alloy electroplating evidently, from 63.28% without hydrogen inhibitor up to 83.54% with a hydrogen inhibitor at a volume fraction of 2.0%, while it has a minor influence on that of pure Zn plating, which maintains at 80%. The optimum volume fraction of hydrogen inhibitor is 2.0%.
Key words: Zn-Fe alloy; hydrogen inhibitor; current efficiency; electrochemical behavior; electrodeposition
1 Introduction
Zinc coatings are recognized for providing excellent protection to steel against corrosion mainly because of their sacrificial behavior. Electrodeposition of Zn-based alloys has been investigated extensively for decades because alloys can enhance the corrosion resistance for a longer period of time[1-4].
At present, zinc-iron group alloys have been applied widely for the excellent properties of coatings. The elelctrodeposition of Zn-Fe alloy is usually conducted in acidic baths such as chloride bath, sulfate bath, sulfate-chloride bath, aqueous acetate bath; as well as in some alkaline baths, either cyanide bath or non-cyanide bath; and in methanol bath[5-9]. Among all kinds of electroplating systems, zinc alloy coatings electroplated in the alkaline zincate solution have attracted more attention throughout the world because of their beneficial properties, such as excellent corrosion resistance, good ductibility, well painted characteristics, low hydrogen embrittlement, small internal stress, and electrolyte has the advantages of simple components, good spreading and covering power, being propitious to electroplate complicated accessory, less corrosion to equipment and low cost. However, the low current efficiency (50%-80%, commonly about 65%) has limited their development and application, compared with that of weak acidic chloride system (above 95%)[10-12]. In order to improve the corrosion resistance of the Zn layer a little Fe was introduced into the Zn layer. Unfortunately, it decreases the current efficiency further, and the higher the content of Fe, the lower the current efficiency of electroplating process[13]. The reason is that, on one hand, the negative deposition potential of complex Zn(OH)42- results in hydrogen evolution inevitably, on the other hand, the smaller overpotential of hydrogen on Fe than that on Zn promotes hydrogen evolution[14-16]. So it is of significance to exploit proper technique to improve current efficiency of Zn-Fe alloy codeposition.
In this work, based on atom structure analysis of Fe, hydrogen inhibitors were optimized. And the effects and electrochemical behaviors of hydrogen inhibitor on current efficiency were investigated, which can offer theoretical guides to further application of Zn-Fe alloy codeposition.
2 Experimental
Electroplating system was constituted of copper piece served as cathode, zinc board as anode and electrolyte. During electroplating process, stable current was output by electroplating rectifier of style DZZ-4. The thickness of alloy coating was controlled to be more than 5 μm in order to make sure the accuracy. The alloy coating was dissolved by 1?1 HCl (volume ratio), and the content of Fe in coating expressed in the form of mass fraction was measured by spectrophotometer of style 721. Cathodic current efficiency was measured by copper coulometer[17-18].
Three-electrode system was adopted. H-style cell was served as electrobath; the platinum electrode with an area of 9.84×10-3 cm2 was used as working electrode in order to eliminate the effect of hydrogen evolution in alkaline solution; a platinum piece with larger area compared with working electrode was used as counter electrode; and Hg/HgO electrode dipped in 1 mol/L NaOH solution was used as reference electrode. Salt bridge with Luggin capillary was used to eliminate border potential between different solutions and to decrease Ohm resistance of the solution. The surfaces of specimens were polished by 165 μm abrasive paper, dipped successively in acetone and washed by double distilled water before immersion in electrolyte. Before polarization measurements began, the solution was purged by a stream of purified N2 for at least 15 min in order to remove the dissolved oxygen. The cell was maintained in a thermostated water bath to keep a constant temperature. The steady-state polarization curves were measured with a CHI660a electrochemical workstation monitored by computer.
The electrolyte for depositing Zn was freshly prepared from double distilled demineralised water and analytical grade reagents. The general electrolyte composition for electrodepositing Zn was 18 g/L ZnO and 180 g/L NaOH; and for electrodepositing Zn-Fe alloy, the electrolyte was composed of 18 g/L ZnO, 180 g/L NaOH, 0.16 g/L Fe2+, 0.6 g/L ascorbic acid, 15 mL/L triethanolamine.
The electrodeposition operating conditions for Zn and Zn-Fe alloys from zincate baths were as follows: cathodic current density 2 A/dm2, temperature 30 ℃, electroplating time 30 min, scan rate for stable polarization 1 mV/s, and that for cyclic voltammetry 20 mV/s.
3 Effect of hydrogen inhibitor on cathodic current efficiency of Zn and Zn-Fe alloy elelctrodeposition
For zinc ion, Zn(OH)42- is the main form in the electrolyte, and correspondingly standard electrode potential moves negatively to -1.219 V (the change of potential is 0.456 V). The potential is so negative that hydrogen will occur inevitably. In order to improve the corrosion resistance of Zn layer a small amount of Fe was introduced, which will decrease the current efficiency. Additionally, the overpotentials of hydrogen evolution are different in different media or on different metals. And the overpotential of hydrogen evolution on Fe, Co and Ni is smaller than that on Zn and Cu. Therefore, how to control serious hydrogen evolution effectively during Zn-Fe alloy codeposition in alkaline zincate system was emphasized in this work.
Most of metals can deposit near their equilibrium potential in aqueous solution, while Fe group metals deposit at the great overpotential of several hundreds mV. So during the electrodeposition of Fe, outer materials easily absorb on the surface. In addition, there is non- integral electron in 3d orbit of Fe group elements (Fe 2.2, Co 1.6 and Ni 0.6), so the property of magnetism makes Fe group metals easily absorb hydrogen atom, compound with sulfur and nitrogen[19-20].
Based on this property, hydrogen inhibitor with excellent performance and composed of the sulfur group elements was chosen.
3.1 Effect of hydrogen inhibitor on current efficiency of Zn deposition
Fig.1 shows the effect of hydrogen inhibitor concentration on current efficiency of Zn electro- deposition. Obviously, there is a minor influence of hydrogen inhibitor on current efficiency, which maintains at about 80%. Current efficiency cannot be enhanced further by hydrogen inhibitor because there is a higher overpotential of hydrogen evolution from zinc electrode. Lower current efficiency of zinc deposition is caused by negative potential of zinc complex discharging on cathode.
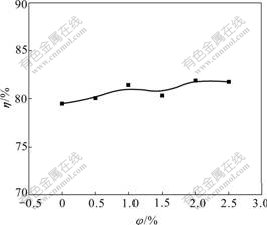
Fig.1 Effect of volume fraction of hydrogen inhibitor (φ) on cathodic current efficiency (η) of Zn electrodeposition
3.2 Effect of hydrogen inhibitor on current efficiency and Fe content of Zn-Fe alloy codeposition
The effects of volume fraction of hydrogen inhibitor on current efficiency and mass fraction of Fe in alloy layer of Zn-Fe alloy codeposition are shown in Fig.2. There is an evident influence of hydrogen inhibitor on current efficiency, while a little influence on Fe content in alloy layer. Current efficiency increases from 63.28% up to the maximum value of 83.54% as the volume fraction of hydrogen inhibitor in electrolyte changes from 0 to 2.0%, and then decreases with the increase of hydrogen inhibitor because too many active points are occupied. The mass fraction of Fe in alloy layer increases within the range from 0.45% to 0.58% with the variation of hydrogen inhibitor content.
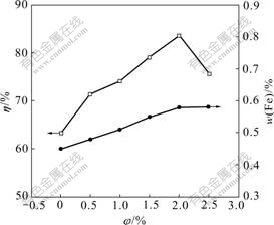
Fig.2 Relationship between volume fraction of hydrogen inhibitor and current efficiency and mass fraction of Fe in coating
By comparing Fig.1 with Fig.2, it can be concluded that for Zn deposition there is a minor influence of hydrogen inhibitor on current efficiency, while for Zn-Fe alloy codeposition there is an obvious influence. This indicates that hydrogen inhibitor enhances current efficiency of Zn-Fe alloy codeposition through improving the overpotential of hydrogen evolution on Fe. According to the property of impure substances being easily adsorbed on the surface of Fe group metals, the improvement of current efficiency can be explained by the fact that hydrogen inhibitor can be adsorbed on Fe surface more effectively than hydrogen atom.
4 Electrochemical behaviors of current efficiency improvement by hydrogen inhibitor
4.1 Effect of hydrogen inhibitor on stable polarization curves of Zn and Fe deposition
Fig.3 shows the effect of hydrogen inhibitor on stable polarization curves of Zn and Fe deposition. It is clearly indicated that there is a great effect of hydrogen inhibitor on Fe deposition, and hydrogen evolution potential on Fe negatively moves from -1.14 to -1.19 V with the addition of hydrogen inhibitor. While there is a little effect of hydrogen inhibitor on Zn deposition, and hydrogen evolution potential on Zn moves only from -1.41 to -1.42 V. These results are consistent with those of technical studies.
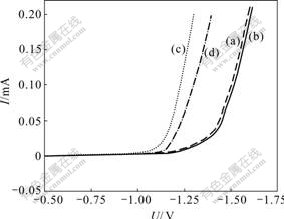
Fig.3 Effect of hydrogen inhibitor on stable polarization curves of Zn and Fe deposition: (a) Zn; (b) Zn and hydrogen inhibitor; (c) Fe; (d) Fe and hydrogen inhibitor
4.2 Effect of hydrogen inhibitor on stable polarization curves of Zn-Fe alloy codeposition
The effect of hydrogen inhibitor on stable polarization curves of Zn-Fe alloy codeposition is shown in Fig.4. And hydrogen evolution potential, peak current and peak potential are listed in Table 1.
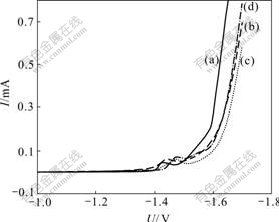
Fig.4 Effect of volume fraction of hydrogen inhibitor on stable polarization curves of Zn-Fe alloy codeposition: (a) 0; (b) 1.0%; (c) 2.0%; (d) 3.0%
Table 1 Hydrogen evolution potential, peak current and potential of Zn-Fe alloy codeposition
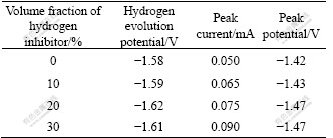
From Fig.4 and Table 1 it can be induced that hydrogen evolution overpotential during alloy codeposition is enhanced obviously with the addition of hydrogen inhibitor. Hydrogen evolution potential negatively moves until the volume fraction of hydrogen inhibitor up to 2.0%, and then the effect begins to decrease. The peak current and peak potential of Zn-Fe alloy codeposition are all changed when hydrogen inhibitor is added into the electrolyte. The former is improved slightly and the latter moves negatively, which illustrates that hydrogen evolution is inhibited when the overpotential is enhanced, and at the same time the cathodic polarization degree of alloy is improved and codeposition is accelerated.
4.3 Effect of hydrogen inhibitor on cyclic voltamme- tric curves of Zn and Fe deposition
Fig.5 shows the effect of hydrogen inhibitor on cyclic voltammetric curves of Zn and Fe deposition. There is minor effect on curve of Zn by comparison with that of Fe. When hydrogen inhibitor is added into electrolyte, hydrogen evolution overpotential in the process of Fe2+ cathodic reduction moves negatively and peak current increases evidently from 0.044 to 0.085 mA, which implies that current efficiency is improved through restraining hydrogen evolution and promoting Fe deposition.
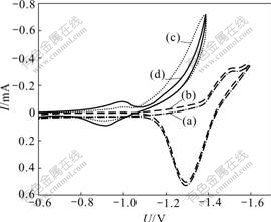
Fig.5 Effect of hydrogen inhibitor on cyclic voltammetric curves of Zn and Fe deposition: (a) Zn; (b) Zn and hydrogen inhibitor; (c) Fe; (d) Fe and hydrogen inhibitor
4.4 Effect of hydrogen inhibitor on cyclic voltamme- tric curves of Zn-Fe alloy codeposition
The effect of hydrogen inhibitor on cyclic voltammetric curves of Zn-Fe codeposition is shown in Fig.6. There exists a maximum value of negative movement of hydrogen evolution potential, and an optimum volume fraction of hydrogen inhibitor. There is a minor effect of hydrogen inhibitor on cathodic and anodic peak potentials. However, when the volume fraction of hydrogen inhibitor is added from 0 to 3.0%, anodic peak current of Zn-Fe alloy codeposition increases from 0.54 to 0.57, 0.65 and 0.66 mA, respectively, which illustrates that the alloy codeposition is promoted through restraining hydrogen evolution.
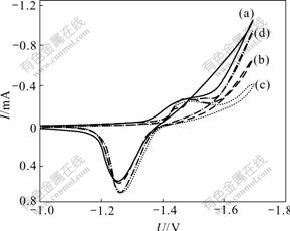
Fig.6 Effect of volume fraction of hydrogen inhibitor on cyclic voltammetric curves of Zn-Fe alloy codeposition: (a) 0; (b) 1.0%; (c) 2.0%; (d) 3.0%
4.5 Effect of hydrogen inhibitor on hydrogen evolu- tion overpotential
According to the above results it can be concluded that the deposition process of Zn-Fe alloy is accompanied by hydrogen evolution inevitably and then cathodic current efficiency cannot reach up to 100%, but it can be improved as much as possible through controlling the optimum technical conditions or choosing moderate hydrogen inhibitor to restrain hydrogen evolution. The research results confirm that hydrogen inhibitor composed of sulfur group compound can distinctly improve the current efficiency. The effect of hydrogen inhibitor on overpotential of hydrogen evolution, which is obtained from the stable polarization curves under different concentrations, is shown in Fig.7.
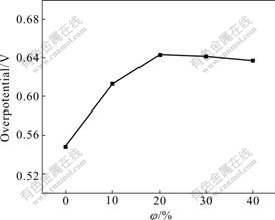
Fig.7 Effect of volume fraction of hydrogen inhibitor on overpotential of hydrogen evolution
When the volume fraction of hydrogen inhibitor is less than 2.0%, hydrogen evolution overpotential increases from 0.548 to 0.642 V with increasing concentration of hydrogen inhibitor, however, it decreases slightly when hydrogen inhibitor is further increased, which are identical to the experimental results. The optimum volume fraction of hydrogen inhibitor is 2.0%.
5 Conclusions
1) Hydrogen inhibitor is composed of the sulfur group compound. Studies on current efficiency illustrate that there is a minor effect of hydrogen inhibitor on current efficiency of Zn electroplating, which is caused by negative discharging potential of Zn(OH)42- and hydrogen evolution. Hydrogen inhibitor can increase the current efficiency of Zn-Fe alloy electroplating evidently, from 63.28% without hydrogen inhibitor up to 83.54% with hydrogen inhibitor at volume fraction of 2.0%. At the same time the content of Fe in coating increases slightly from 0.45% to 0.58%, which indicates that current efficiency is improved through enhancing the overpotential of hydrogen evolution, restraining hydrogen evolution and promoting Fe deposition.
2) Research results on electrochemical behaviors also indicate that there is a minor effect of hydrogen inhibitor on the process of Zn deposition, while great effect on that of Fe. When hydrogen inhibitor is added into the electrolyte, current efficiency is improved through promoting overpotential of hydrogen evolution and alloy codeposition. The optimum volume fraction of hydrogen inhibitor is 2.0%.
References
[1] LODHI Z F, MOL J M C, HOVESTAD A, TERRYN H, de WIT J H W. Electrodeposition of Zn-Co and Zn-Co-Fe alloys from acidic chloride electrolytes [J]. Surface and Coating Technology, 2007, 202(1): 84-90.
[2] LAN C J, LIU W Y, KE S T, CHIN T S. Potassium salt based alkaline bath for deposition of Zn-Fe alloys [J]. Surface and Coating Technology, 2006, 201(6): 3103-3108.
[3] BARBOSA L L, CARLOS I A. Development of a novel alkaline zinc-iron plating bath containing sorbitol and the chemical, physical and morphological characterization of the Zn-Fe films [J]. Surface and Coating Technology, 2006, 201(3/4): 1695-1703.
[4] YANG Z N, ZHANG Z, ZHANG J Q. Electrodeposition of decorative and protective Zn-Fe coating onto low-carbon steel substrate [J]. Surface and Coating Technology, 2006, 200(16/17): 4810-4815.
[5] ORDINE A P, DIAZ S L, MARGARIT I C P, MATTOS O R. Zn-Ni and Zn-Fe alloy deposits modified by P incorporation anticorrosion properties [J]. Electrochimica Acta, 2004, 49(26): 2815-2823.
[6] DIAZ S L, MATTOS O R, BARCIA O E, FABRI MIRANDA F J. Zn-Fe anomalous electrodeposition: Stationeries and local pH measurements [J]. Electrochimica Acta, 2002, 47(25): 4091-4100.
[7] GOMEZ E, PELAEZ E, VALLES E. Electrodeposition of zinc-iron alloys (I): Analysis of the initial stages of the anolaous codeposition [J]. Journal of Electroanalytical Chemistry, 1999, 469(2): 139-149.
[8] GOMEZ E, PELAEZ E, VALLES E. Electrodeposition of zinc-iron alloys (II): Relation between the stripping results and ex-situ characterization [J]. Journal of Electroanalytical Chemistry, 1999, 475(1): 66-72.
[9] FAN Yun-ying, ZHANG Ying-jie, DONG Peng. Preparation and property of electrodeposited Zn-Fe-SiO2 composite coating [J]. Key Engineering Materials, 2008, 373/374: 212-215.
[10] KARAHAN I H, CETINKARA H A, GUDER H S. Electrodeposition and characterisation of Zn, Zn-Fe and Zn-Fe-Ni coatings in presence of gelatin as additive [J]. Transactions of the Institute of Metal Finishing, 2008, 86(3): 157-161.
[11] ORDINE A P, DIAZ S L, MARGARIT I C P, MATTOS O R. Zn-Ni and Zn-Fe alloy deposits modified by P incorporation: Anticorrosion properties [J]. Electrochimica Acta, 2004, 49(17/18): 2815-2823.
[12] RAMANAUSKAS R. GUDAVICIUTE L, SCIT O, BUCINSKIENE D, JUSKENAS R. Pulse plating effect on composition and corrosion properties of zinc alloy coatings [J]. Transactions of the Institute of Metal Finishing, 2008, 86(2): 103-108.
[13] WANG Yun-yan, PENG Wei-jie. Study on cathodic current efficiency of Zn electroplating in alkaline zincate solution [J]. Electroplating and Environmental Protection, 2003, 23(6): 12-16. (in Chinese)
[14] WANG Yun-yan, PENG Wei-jie. Study on cathodic current efficiency of Zn-Fe alloy electroplating in alkaline zincate solution [J]. Material Protection, 2004, 37(5): 16-18.(in Chinese)
[15] WANG Yun-yan, PENG Wen-jie, CHAI Li-yuan, SHU Yu-de. Study on technology of Zn-Fe alloy electroplating in alkaline zincate solution [J]. Electroplating and Environmental Protection, 2003, 23(2): 11-14. (in Chinese)
[16] WANG Yun-yan, PENG Wen-jie, CHAI Li-yuan, SHU Yu-de. Electrochemical behaviors of Zn-Fe alloy and Zn-Fe-TiO2 composite electroplating [J]. Journal of Central South University of Technology, 2003, 10(3): 183-189.
[17] ZHANG Yun-cheng, HU Ru-nan, XIANG Rong. Electroplating handbook [M]. Beijing: National Defense Industry Press, 1997: 95-154. (in Chinese)
[18] TU Zhen-mi. Principle and technology of alloy electroplating [M]. Beijing: National Defense Industry Press, 1993: 231-243. (in Chinese)
[19] SHU Yu-de, CHEN Bai-zhen. Research methods of metallurgical chemistry [M]. Changsha: Central South University of Technology Press, 1990: 173-205. (in Chinese)
[20] ZHA Quan-xing. Introduction to dynamics of electrode process [M]. 2nd ed. Beijing: Science Press, 1987: 407-415. (in Chinese)
Foundation item: Project(50274073) supported by the National Natural Science Foundation of China
Received date: 2008-03-02; Accepted date: 2008-05-08
Corresponding author: CHAI Li-yuan, Professor, PhD; Tel: +86-731-8836921; E-mail: lychai@mail.csu.edu.cn
(Edited by CHEN Wei-ping)