
Preparation of nano-platinum and its catalytic activity toward methanol oxidation and oxygen reduction
HUANG You-ju(黄幼菊), DAI Hong-hai(戴宏海), LI Wei(李 伟),
ZHANG Qing-long(张庆龙), ZHAO Ling-zhi(赵灵智), LI Wei-shan(李伟善)
Department of Chemistry, South China Normal University, Guangzhou 510006, China
Received 15 July 2007; accepted 10 September 2007
Abstract: Nano-scale platinum catalysts were prepared on a glass carbon electrode by cyclic voltammetry. The surface morphology and active area of the catalysts, and their catalytic activity toward methanol oxidation and oxygen reduction were studied by SEM, linear and cyclic voltammetry. The result shows that the diameters of global Pt particles are affected by the scan rate of cyclic voltammetry: the faster the scan rate is, the smaller the diameters of Pt particles are. The size of the nano-scale platinum catalysts has different effects on their catalytic activity toward oxygen reduction and methanol oxidation: the catalyst with a size of 100 nm shows its best activity toward methanol oxidation, but the catalyst with a size of 65 nm shows its best activity toward oxygen reduction.
Key words: nano; platinum; catalysis; methanol oxidation; oxygen reduction
1 Introduction
There has been an increasing interest in the development of proton exchange membrane fuel cells (PEMFC) and the direct methanol fuel cell(DMFC) in the recent years, since they have a lot of advantages as a tool for energy use[1-2]. Highly dispersive platinum supported on carbon has been widely used in various electrochemical systems, particularly in oxygen reduction electrocatalysis[3-4]. However, the oxygen reduction proceeds at a very slow kinetic rate in both PEMFC and DMFC. The exchange current density of the oxygen reduction reaction at the Pt catalyst electrode is as low as 10-10 A/cm2. Obtaining a cathodic catalyst with a high electrocatalytic activity for oxygen reduction is important for the development of both PEMFCs and DMFCs[5-6].
In general, two ways have been developed to improve the electrocatalytic activity of the Pt catalyst for oxygen reduction. First, Pt-based complex catalysts were developed[7-10], such as Pt-Co, Pt-Fe, Pt-Cr, Pt-HxMoO3. Second, the Pt catalysts with an optimum structure were prepared by a suitable preparation method because structural factors, such as the average size and the relative crystallinity of the metal particles, the electrochemically active area and the alloying content of the complex catalysts. can significantly affect the final electrocatalytic activity of the catalysts[11]. Moreover, the preparation method has an important influence on the structure of the catalysts. The two aspects that have special meanings in numerous investigations on supported Pt catalysts are particle size effect and metal-support interaction[12]. The particle size effect is closely connected with the structure sensitivity of oxygen reduction on platinum. The experimental results of oxygen reduction on Pt single crystal planes in acid media show a relatively small structure sensitivity [13-15].
Poirier and Stoner found that the oxygen reduction activity on thin film Pt depends on the grain size, specific surface area and lattice parameter[16]. The experimental data in 0.5 mol/L H2SO4 showed that the specific activity of O2-reduction decreases with increasing grain size. The activity decreases was not in agreement with the previous observations in oxygen reduction electro- catalysis on highly dispersive platinum[12], indicating that the examination of thin-film Pt catalysts can give novel insights into platinum electrocatalysis. Therefore, the preparation methods for the Pt-based catalysts for DMFC are attracting much attention of investigators [17-18]. The objective of the present work is to study the effect of the size of nano-meter Pt on its catalytic activity toward oxygen reduction and methanol oxidation.
2 Experimental
The preparation and electrochemical measurement of the catalysts were conducted in a cell with an Ag/AgCl/saturated KCl electrode as the reference electrode and a platinum sheet electrode as the counter electrode. The potentials in the present paper are with respect to this reference electrode. In the preparation of the catalysts, a glassy carbon disk electrode with a diameter of 3 mm was used as the working electrode. Before the preparation, the working electrode was polished with 0.05 μm alumina, and then cleaned by ultrasonator in acetone and distilled water successively and by cycling in 0.5 mol/L H2SO4 between 0.2 V and 0.9 V at 50 mV/s. Five catalysts was prepared by cycling the cleaned glass carbon disk electrode for 2 cycles between -0.3 V and 0.6 V at 10, 20, 30, 40 and 50 mV/s in the quiescent solutions of 5 mmol/L H2PtCl6+0.5 mol/L H2SO4, respectively. Before the electrochemical measurement, the working electrode with catalyst was placed in 0.5 mol/L H2SO4 and the cell was deaerated for half an hour under open circuit potential, and then the electrode was cycled for 6 cycles between -0.3 V and 1.1 V at 50 mV/s. In the study of oxygen reduction, the solution was saturated with O2.
H2PtCl6 was spectrum grade and other chemicals used were analytical grade. Solutions were prepared with distilled water. Highly purified nitrogen was used to deaerate the solutions and highly purified oxygen was used for oxygen reduction. All the electrochemical experiments were carried out under room temperature.
The electrochemical experiments were performed using the cyclic and linear voltammetry with an electrochemical instrument (Eco Echemine BV) and a rotating disk electrode (RDE, model ATA-1B). The morphology of the prepared catalysts was observed with a scan electron microscopy (Thermal field emission environmental SEM-EDS-EBSD, model Quanta 400F, FEI/ OXFORD/ HKL).
3 Results and discussion
3.1 Cyclic voltammograms of Pt catalysts
Five Pt catalysts were prepared by cycling the cleaned glass carbon disk electrode for 2 cycles between -0.3 V and 0.6 V at 10, 20, 30, 40 and 50 mV/s in the quiescent solutions of 5 mmo/L H2PtCl6+0.5 mol/L H2SO4, respectively. Fig.1 presents the voltammograms of prepared catalysts Pt(a), Pt (b), Pt(c), Pt(d), Pt (e) on glassy carbon disk electrode in deaerated 0.5 mol/L H2SO4 solution. It can be seen from Fig.1 that the slower the electrodeposited scan rate is, the larger the surface area of the catalyst Pt is. The hydrogen adsorption/ disadsorption current peak appears between the potential -0.2-0 V. Platinum oxide is formed at the potential more positive than 0.6 V and is reduced between the potential 0.3-0.8 V. Estimated by integrating the oxidation peak area of adsorbed hydrogen, the real surface area of these five catalyst are 0.036 82, 0.025 19, 0.020 34, 0.019 22 and 0.010 73 cm2, for the preparation at 10, 20,30, 40 and 50 mV/s, respectively.
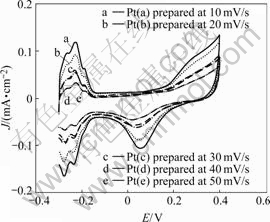
Fig.1 Cyclic voltammograms of five Pt catalysts prepared in 0.5 mol/L H2SO4 solution with scan rate of 50 mV/s
3.2 Surface morphology of Pt catalysts
The surface morphology of the catalysts Pt (Pt(a), Pt(b), Pt(d), Pt(e)) were observed by scan electron spectroscopy. The results obtained are shown in Fig.2. It can be seen that there is little difference in the surface morphology between these four Pt catalysts. All of them are global and the average diameters of the global grains are 130, 100, 80 and 65 nm for catalysts Pt(a), Pt(b), Pt(d) and Pt(e), respectively. That is to say, the larger the scanning rate is, the smaller the average diameters of the Pt global grains are.
3.3 Activity of Pt toward methanol oxidation
The cyclic voltammograms of the Pt catalysts on rotating glassy carbon disk electrode were obtained in 0.2 mol/L CH3OH+0.5 mol/L H2SO4 solution, as shown in Fig.3. It can be seen that the methanol oxidation current density on catalyst Pt(b) is the largest among these five Pt catalysts. The average diameter of catalyst Pt(b) global grains is about 100 nm. This indicates that the catalytic ability of nano-meter Pt toward methanol oxidation depends on its grain size. The experiment results show that the size of the 100 nm nano-scale platinum catalysts has the best catalytic ability toward methanol oxidation.
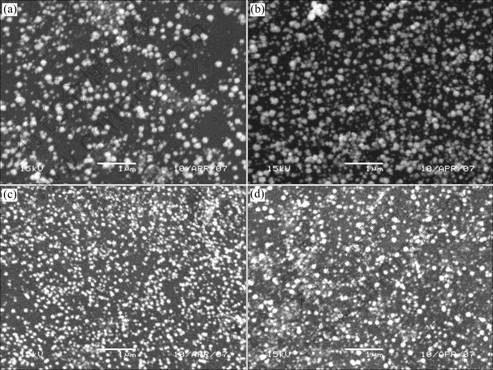
Fig.2 Scanning electron micrographs of Pt catalysts (Pt(a) in Fig.2(a), Pt(b) in Fig.2(b), Pt(d) in Fig.2(c) and Pt(e) in Fig.2(d)) on glass carbon electrode
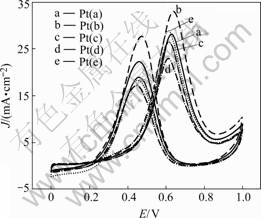
Fig.3 Voltammograms of Pt catalysts in 0.2 mol/L+0.5 mol/L H2SO4 with scan rate of 50 mV/s
3.4 Activity of Pt toward oxygen reduction
The voltammograms of the catalyst Pt(b) were obtained in the solution with and without methanol. Fig.4 shows that oxygen reduction on catalyst Pt(b) in O2-saturated 0.5 mol/L H2SO4 solutions with and without 0.8 mmol/L methanol. Oxygen reduction current density on Pt(b) in the solution without methanol is larger than on that in methanol containing solution, indicating that the activity of the catalyst Pt(b) decreases when the solution contains methanol. Thus catalyst Pt(b) is not suitable for catalytic oxygen reduction.
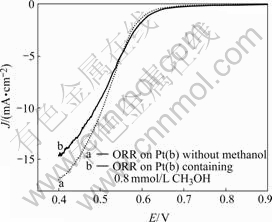
Fig.4 Voltammograms of Pt(b) in 0.5 mol/L O2-saturated H2SO4 without (curve a) and with (curve b) 0.8 mmol/L methanol with scan rate of 5 mV/s (ORR represents oxygen reduction reaction.)
The linear voltammograms of five catalysts on rotating glassy carbon disk electrode were obtained in O2-saturated 0.5 mol/L H2SO4 solutions, as shown in Fig.5. It can be seen that the oxygen reduction current density on catalysts increases as the grain size of the catalysts becomes smaller and the catalyst Pt(e) has the largest activity among these five Pt catalysts. The average diameter of catalyst Pt(e) global particles is 65 nm.
Fig.6 shows the linear voltammograms of Pt(e) in O2-saturated 0.5 mol/L H2SO4 solutions with and without methanol. It should be noted that the oxygen reduction current on Pt(e) is not reduced but improved by the existence of methanol. This is important for the application of DMFC, which faces the serious problem that methanol in anode penetrates through membrane to cathode.
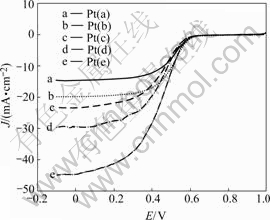
Fig.5 Voltammograms of catalysts in 0.5 mol/L oxygen saturated H2SO4 with scan rate of 5 mV/s
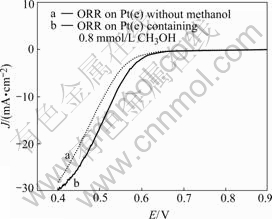
Fig.6 Voltammograms of Pt(e) in 0.5 mol/L O2-saturated H2SO4 without (curve a) and with (curve b) 0.8 mmol/L methanol with scan rate of 5 mV/s
4 Conclusions
1) The particle size of the platinum catalyst related to potential scan rate when it is prepared by the cyclic voltammetry. The faster the scan rate is, the smaller the diameters of Pt particles are.
2) The activity of nano-meter platinum catalysts toward oxygen reduction and methanol oxidation depends to a great extent on particle size of the catalysts. The catalyst with a size of 100 nm shows its best activity toward methanol oxidation, but the catalyst with a size of 65 nm shows its best activity toward oxygen reduction.
References
[1] WASMUS S, KUVER A, Methanol oxidation and direct methanol fuel cells: A selective review [J]. J Electroanal Chem 1999, 461: 14-31.
[2] MC NICOL B D, RAND D A J, WISSIAMS K R. Direct methanol-air fuel cells for road transportation [J]. J Power Sources, 1999, 83: 15-31.
[3] KINOSHITA K. Particle size effects for oxygen reduction on highly dispersed platinum in acid electrolytes [J]. J Electrochem Soc, 1990, 137: 845-848.
[4] PAULUS U A, SCHMIDT T J, GASTEIGER H A, BEHM R J. Oxygen reduction on a high-surface area Pt/Vulcan carbon catalyst:a thin-film rotating ring-disk electrodes study [J]. J Electronal Chem, 2001, 495: 134-145.
[5] ZHOU Zhen-hua, ZHOU Wei-jiang, WANG Su-li, WANG Guo-xiong, JIANG Lu-hua, LI Gong-qian, XIN Qin. Preparation of highly active 40wt.% Pt/C cathode electrocatalysts for DMFC via different routes [J]. Catal Today, 2004, 93/95: 523-528.
[6] APPLEBY A J, FOULKES F R. Fuel cell handbook [M]. New York: VanNostrand Reinhold, 1989.
[7] TODA T, IGARASHI H, UCHIDA H. Enhancement of the electroreduction of oxygen on Pt alloys with Fe, Ni, and Co [J]. J Electrochem Soc, 1999, 146: 3750-3760.
[8] MUKERJEE S, SRINIVASAN S, SORIAGA M P. Effect of activities for oxygen reduction XRD, XAS, and electrochemical studies [J]. J Phys Chem, 1995, 99: 4577-4589.
[9] XU Y, RUBAN A V, MAVRIKAKIS M. The adsorption and dissociation of O2 on Pt-Co and Pt-Fe alloys [J]. J Am Chem Soc, 2004, 126: 4717-4725.
[10] HUANG You-ju, LI Wei-shan, HUANG Qing-dan, LI Wei, ZHANG Qing-long, JIANG La-sheng. Catalytic activity improvement of platinum toward oxygen reduction reaction by hydrogen molybdenum bronze [J]. Chem J Chinese University, 2007, 28(5): 921-924.
[11] ATTWOOD P A, MC NICOL B D, SHORT R T. The electrocatalytic oxidation of methanol in acid electrolyte preparation and characterization of noble metal electrocatalysts supported on pre-treated carbon-fiber papers [J]. J Appl Electrochem, 1980, 10(2): 213-222.
[12] MUKERJEE S. Particle size and structural effects in platinum electrocatalysis [J]. J Appl Electrochem, 1990, 20: 537-548.
[13] MARKOVIC N M, GASTEIGER H A, ROSS P N. Oxygen reduction on platinum low-index single-crystal surfaces in sulphuric acid solution: Rotating ring-Pt (hlk) disk studies [J]. J Phys Chem, 1995, 99: 3411-3415.
[14] KITA H, LEI H W, GAO Y Z. Oxygen reduction on platinum single-crystal electrodes in acidic solutions [J]. J Electroanal Chem, 1994, 379: 407-414.
[15] EI KADIRI F, FAURE R, DURAND R. Electrochemical reduction of molecular oxygen on platinum single crystals [J]. J Electroanal Chem, 1991, 301: 177-188.
[16] POIRIER J A, STONER G E. Microstructural effects on electrocatalytic oxygen reduction activity of nano-grained thin-film platinum in acid-media [J]. J Electrochem Soc, 1994, 141: 425-430.
[17] BONNEMANN H, BRIJOUX W, BRINKMANN R. Formation of colloidal transition. metals in organic phases and their application in catalysis [J]. Angew Chem Int Ed Eng, 1991, 30: 1312-1324.
[18] SCHMIDT T J, NOESKE M, GASTEIGER H A, BEHM R J. Electrocatalytic activity of PtRu alloy colloids for CO and CO/H2 electrooxidation: Stripping voltammetry and rotating disk measurements [J]. Langmuir, 1997, 13: 2591-2595.
(Edited by LAI Hai-hui)
Foundation item: Project(20573039) supported by the National Natural Science Foundation of China; Project(2005DFA60580) supported by CISTC; Project(2005B50101003) supported by Guangdong Province, China
Corresponding author: LI Wei-Shan; Tel: +86-20-39310256; E-mail: liwsh@scnu.edu.cn