
Effect of Cu2+ ions on bioleaching of marmatite
CHEN Song(陈 松), QIN Wen-qing(覃文庆), QIU Guan-zhou(邱冠周)
Key Laboratory of Biometallurgy of Ministry of Education,
School of Minerals Processing and Bioengineering, Central South University, Changsha 410083, China
Received 20 September 2008; accepted 5 November 2008
Abstract: The effect of Cu2+ ions on bioleaching of marmatite was investigated through shake leaching experiments. The bacteria inoculated are a mixed culture of Acidithiobacillus ferrooxidans, Acidithiobacillus thiooxidans and Lepthospirillum ferrooxidans. The results show that zinc is selectively leached, and the addition of appropriate content of Cu2+ ions has positive effect on the bioleaching of marmatite. SEM and EDX analyses of the leaching residue reveal that a product layer composed of iron sulfide, elemental sulfur and jarosite forms on the mineral surface. The biooxidation of elemental sulfur is catalyzed by the Cu2+ ions, which eliminate the barrier to bioleaching of marmatite and keep low pH value. With the addition of 0.5 g/L Cu2+ ions, the maximum zinc extraction rate reaches 73% after 23 d at the temperature of 30 ℃ with the pulp density of 10%, while that of iron is only about 10%.
Key words: Cu2+ ions; marmatite; bioleaching; zinc
1 Introduction
Biohydrometallurgy is a new crossed subject, which involves the microbiology, mineral engineering, chemical engineering and metallurgy. Biohydro- metallurgy is regarded as a new developing way of modern resources extraction, of which bioleaching is emphatically concerned and studied. Presently, bioleaching has been commercially applied in the extraction of copper, gold and uranium from sulfides. Bioleaching of zinc, cobalt and nickel from sulfides has been extensively studied and commercially used in some cases[1-4]. Bioleaching shows competitive advantages in the treatment of low grade ores and refractory ores attributing to friendly environment, high selectivity and low cost. However, due to its slow kinetics, the commercial application of bioleaching in metal extraction is limited relatively. Therefore, studies on bacteria culture, accession of metal ions, surfactants and electric or magnetic field, were carried out in order to enhance the bioleaching rate[5-9]. Marmatite concentrate is characterized by high content of iron. When the concentrate is subjected to the roasting- leaching process, it is easy to form ferrite (ZnO·Fe2O3), which decreases the extraction rate of zinc. Pressure oxidation is an effective way to treat marmatite concentrate, by which the extraction rate of zinc is enhanced while that of iron is decreased. Bioleaching seems to be an alternative method to process marmatite [10-11].
FOWLER and GRUNDWELL carried out experiments to study the bioleaching of sphalerite [12-13]. The results indicated that the role of bacteria was oxidizing the Fe2+ ions and elemental sulfur into Fe3+ ions and sulfuric acid, respectively. No evidence was found to prove that the bacteria had direct attack on the minerals.
ZnS+2Fe3+→Zn2++S0+2Fe2+ (1)
ZnS+2H++0.5O2→Zn2++S0+H2O (2)
2Fe2++0.5O2+2H+
2Fe3++H2O (3)
S0+1.5O2+H2O
SO42-+2H+ (4)
In this work, the selective bioleaching of marmatite was investigated. The effect of Cu2+ ions on the extraction of zinc and the function mechanisms were discussed.
2 Experimental
2.1 Minerals and bacteria
The marmatite mineral was obtained from Gaogeng Mine of Guangxi Province, China. The chemical components of the marmatite were 48% Zn, 17% Fe and 34% S. The marmatite was ground until the particle size was less than 74 ?m of 75%.
The original bacteria were isolated from certain mine waste water and incubated in 250 mL Erlenmeyer flask containing 100 mL 4.5K medium and 5 g marmatite, on an air bath rotary shaker (HZQ-C) at 75 r/min and constant temperature of 30 ℃. The bacteria were the mixed culture of Acidithiobacillus ferrooxidans, Acidithiobacillus thiooxidans and Lepthospirillum ferrooxidans. The mixed culture was kept in refrigerator at temperature of 4 ℃. It was transferred and incubated monthly. The pH value was adjusted to 2.0 by diluent sulfuric acid. The composition of 4.5K medium was the same to that of 9K medium except the content of FeSO4·7H2O was halved. The composition of sulfur medium was as follows (g/L): (NH4)2SO4 0.2; MgSO4·7H2O 0.5; FeSO4·7H2O 0.01; CaCl2·2H2O 0.25; and S 20. The initial pH value was adjusted to 2.0. All reagents were in chemical grade, and deionized water was used in all experiments.
Before bioleaching, the bacteria was transferred and incubated. When the bacteria reached an exponential growth phase, the suspended solid in the culture medium was removed by centrifugation in a centrifugal machine (LG10-2.4A) at 3 000 r/min for 10 min. Then the bacteria were harvested from the liquid medium by centrifugation at 10 000 r/min for 30 min. The cells were washed with sulfuric acid (pH=1.80) and re-suspended in iron-free 9K medium to the density of 108 cell/mL, which was ready for the inoculums of the next experiments.
2.2 Experiments
Bioleaching was performed in 250 mL flask containing 100 mL iron-free 9K medium and 10 g marmatite, on the rotary shaker at 75 r/min and constant temperature of 30 ℃. The pulp pH value was adjusted with diluent sulfuric acid to 2.0 steadily, and then 5 mL bacterial solution was inoculated into the flask. At intervals, the water lost by evaporation was compensated by the addition of deionized water, and the pH value was measured. If pH>2.0, it was adjusted to 2.0 by sulfuric acid. Periodically, 2.5 mL supernatant liquid sample was drawn from the flask to determine the content of zinc and iron. 2.5 mL sample was replaced with an equal volume of iron-free 9K nutrient medium.
3 Results and discussion
3.1 Effect of Cu2+ ion on bacterial oxidizing activity
The effect of Cu2+ ions on the biooxidation of Fe2+ ions at the concentration of 1-4 g/L is shown in Fig.1. When the bacteria were incubated in the 9K medium, the Fe2+ ions were oxidized by bacteria as the growth energy source (Reaction (3)). It can be seen that with the increase of concentration of Cu2+ ions, the biooxidation rate of Fe2+ ions decreases. It seems that the bacteria are sensitive to the Cu2+ ions. For the bacteria were isolated from the waste water of a lead and zinc mine, the toleration of some heavy metal ions such as Cu2+ was frail. When the concentration of Cu2+ ions was 1 g/L, the biooxidation rate of Fe2+ ions in 9K medium was near 100% within 54 h, while that in copper-free 9K medium was near 100% within 45 h. It is shown that the negative effect of Cu2+ ions on the biooxidation of Fe2+ ions is little at low concentration.
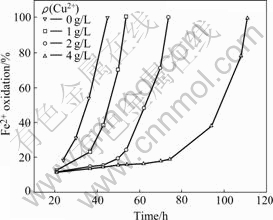
Fig.1 Effect of Cu2+ ion concentration on biooxidation of Fe2+ ions in 9K medium
The effect of Cu2+ ions on the biooxidation of elemental sulfur at the concentration of 0-2.0 g/L is shown in Fig.2. The elemental sulfur is oxidized to sulfuric acid by bacteria (Reaction (4)), so the biooxidation rate of elemental sulfur can be determined qualitatively by the pH value. The more the pH value decreases, the more the elemental sulfur is biooxidized. It can be seen that with the increase of concentration of Cu2+ ions, the biooxidation of elemental sulfur is decreased in 100 h. At 120 h, with the concentration of Cu2+ ions being 0, 0.25, 0.5, 1.0 and 2.0 g/L, the corresponding pH values are 0.88, 0.8, 0.75, 0.8 and 0.87, respectively. It seems that the Cu2+ ions enhance the biooxidation rate of elemental sulfur, especially at the content of 0.5 g/L. This phenomenon is accordant to the results of some research that Cu2+ ions catalyzed the oxidation of thiosulfate[14-15]. The Cu2+ ions show negative effect on the biooxidation of elemental sulfur at the beginning just because the bacteria inoculated are not yet adapted to Cu2+ ions.
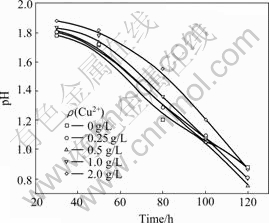
Fig.2 Effect of Cu2+ ion concentration on biooxidation of elemental S in sulfur medium
3.2 Effect of Cu2+ ion on bioleaching of marmatite
Fig.3 shows the effect of Cu2+ ions on the bioleaching rate of marmatite at the concentration of 0-1.0 g/L, which indicates that the Cu2+ ions have stimulative effect on the leaching rate at the concentration of 0.5 g/L. When the Cu2+ ion concentrations are 0, 0.25, 0.5 and 1.0 g/L, the leaching rates of marmatite in 23 d are 65%, 68%, 73% and 67%, respectively. At the beginning of bioleaching, the accession of Cu2+ ions decreases the leaching rate of marmatite. The reason is that the bacteria are sensitive to Cu2+ ions in the first phase of incubation. With increasing the concentration of Cu2+ ions, the growth and oxidation activity (especially oxidation of Fe2+ ions) are depressed. Therefore, the catalyzing effect of Cu2+ ions decreases when the concentration is above 1.0 g/L. It is observed that the extraction rate of iron is only about 10% while that of zinc is 73%, which indicates that zinc is selectively leached during the bioleaching of marmatite. This result is significant to the processing of zinc sulfide bearing high content of iron.
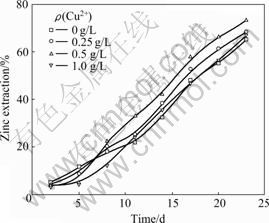
Fig.3 Zinc extraction at various Cu2+ concentrations in shake flask
The pH values were measured periodically during bioleaching. When pH>2.0, it was adjusted to 2.0 by sulfuric acid. Fig. 4 shows the variation of pH values during bioleaching of marmatite. It can be seen that the pH values are above 2.0 though it was adjusted periodically. This indicates the bioleaching of marmatite involves the consumption of acid. However, the variation of pH values is less with the addition of Cu2+ ions. With the increased concentration of Cu2+ ions at 0-1.0 g/L, the increment of pH values in bioleaching are decreased. This can be attributed to the Cu2+ ions catalyzing oxidation of elemental sulfur, which supplies sulfuric acid for the bioleaching automatically. At the beginning of the bioleaching in several days, the increase of pH values was less (especially with the increase of Cu2+ ions concentration) because the bioleaching rate is slow and the consumption of acid is limited correspondingly.
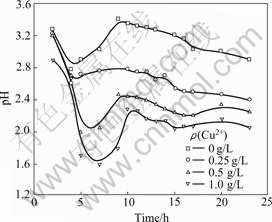
Fig.4 Variation of pH value during bioleaching at various Cu2+ concentrations in shake flask
3.3 SEM and EDX analyses of residue
The results of SEM and EDX analyses of the bioleaching residue of marmatite are shown in Fig.5 and Fig.6. In the case of copper-free medium, the clear mineral surface of the bioleaching residue is observed. The mineral surface is coated with a solid product layer (Fig.5(a)). In the case of 0.5 g/L Cu2+ ions, a relatively clear mineral surface is observed. However, there are porous and loose precipitates scattered on the mineral surface (Fig.6(a)). Fig.7 shows the analysis results of the precipitates. It can be seen that the primary elements are S and Fe. So the precipitates should be a mixture of iron sulfide or jarosite.
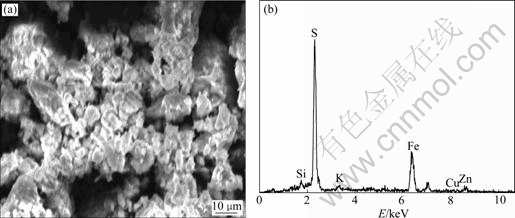
Fig.5 SEM and EDX results of bioleaching residue without addition of Cu2+
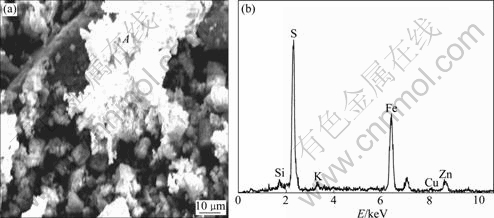
Fig.6 SEM and EDX results of bioleaching residue with addition of 0.5 g/L Cu2+
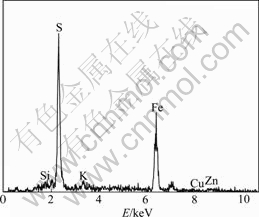
Fig.7 EDX analysis of porous product in residue surface (dot A in Fig.6(a))
The content of Zn, Fe and S on the original mineral surface and the bioleaching residue surface determined by EDX is shown in Table 1. It can be seen that there is great difference between the composition of the mineral surface and the bioleaching residue surface. The Fe content of the bioleaching residue surface increases and the Zn content decreases greatly. The mole fraction of Zn is more than twice of that of Fe in the original marmatite mineral before bioleaching. However, the mole fraction of Fe is above three times of that of Zn in the residue surface after bioleaching. This explains that zinc and iron are not leached simultaneously and proportionally, but that zinc is preferentially leached. The mole fractions of Zn, Fe and S of the original marmatite surface are 34.1%, 14.43% and 50.47%, respectively. The sum of mole fractions of Zn and Fe is nearly the same as the mole fraction of S, which accords with the chemical formula of marmatite (Zn1-xFexS) theoretically. After bioleaching, without the addition of Cu2+ ions, the mole fraction of S in the bioleaching residue surface is 63.75%, and that of Fe and Zn is 27.90% and 4.13%, respectively. The mole fraction of S exceeds the sum of Fe and Zn by 30%, which indicates the existence of elemental sulfur. While bioleaching with the addition of 0.5 g/L Cu2+ ions, the mole fraction of S in the residue surface only exceeds the sum of Fe and Zn by 5%, which indicates the Cu2+ ions catalyze the oxidation of elemental sulfur and there is only a few elemental sulfur existing. Trace of potassium is found in the bioleaching residue just because the potassium ions in the medium is precipitated as jarosite [KFe3(SO4)2· (OH) 6].
Table 1 EDX analysis results of marmatite mineral and bioleaching residue surface

It was reported that Cu2+ ion has electrochemical catalyzing effect on bioleaching of sphalerite[16]. During bioleaching, with the dissolution of zinc from the mineral crystal lattice, some Cu2+ ions substitute zinc ions and inlay the crystal lattice. The copper sulfide formed in this course shows higher potential on sphalerite and improves the conductivity of the mineral surface, so sphalerite dissolves preferentially as anode. From the results of EDX analysis shown in Fig.6, ounce of Cu2+ ions is found in the bioleaching residue of marmatite, which indicates the inbreak of Cu2+ ions into the mineral crystal lattice. Hence, an electrochemical effect of Cu2+ on bioleaching of marmatite is proposed.
4 Conclusions
1) Zinc is selectively leached during bioleaching of marmatite. With the addition of 0.5 g/L Cu2+ ions, the maximum extraction rate of zinc is 73% while that of iron is only about 10% in 23 d.
2) The iron sulfide, elemental sulfur and jarosite are formed on the mineral surface during bioleaching, and they become barrier to bioleaching of marmatite. The Cu2+ ions catalyze the biooxidation of elemental sulfur. As a result, the barrier to bioleaching is eliminated and the pH value decreases. Therefore, the bioleaching rate of marmatite is enhanced.
References
[1] WATLING H R. The bioleaching of sulphide minerals with emphasis on copper sulphides—A review [J]. Hydrometallurgy, 2006, 84: 81-108.
[2] BRIERLEY J A. A perspective on developments in biohydrometallurgy [J]. Hydrometallurgy, 2008, 94(1/4): 2-7.
[3] MOHAPATRA S, BOHIDAR S, PRADHAN N. Microbial extraction of nickel from Sukinda chromite overburden by Acidithiobacillus ferrooxidans and Aspergillus strains [J]. Hydrometallurgy, 2007, 85: 1-8.
[4] MOUSAVI S M, JAFARI A, YAGHMAEI S, VOSSOUGHI M. Bioleaching of low-grade sphalerite using a column reactor [J]. Hydrometallurgy, 2006, 82: 75-82.
[5] AKCIL A, CIFTCI H, DEVECI H. Role and contribution of pure and mixed cultures of mesophiles in bioleaching of a pyritic chalcopyrite concentrate [J]. Minerals Engineering, 2007, 20: 310-318.
[6] GOMEZ E, BALLESTER A, GONZALEZ F. Leaching capacity of a new extremely thermophilic microorganism, Sulfolobus rivotincti [J]. Hydrometallurgy, 1999, 52: 349-366.
[7] G?MEZ C, ROM?N E, BL?ZQUEZ M L. SEM and AES studies of chalcopyrite bioleaching in the presence of catalytic ions [J]. Minerals Engineering, 1999, 20(8): 825-835.
[8] SWAMY K M, NARAYANA K L, MISRA V N. Bioleaching with ultrasound [J]. Ultrasonics Sonochemistry, 2005, 12: 301-306.
[9] SELVI S C, MODAK J M, NATARAJAN K A. Electrobioleaching of sphalerite flotation concentrate [J]. Minerals Engineering, 1998, 11(8): 783-788.
[10] WANG Su-ting, ZHANG Guang-ji, YUAN Qiu-hong, FANG Zhao-heng, YANG Chao. Comparative study of external addition of Fe2+ and inoculum on bioleaching of marmatite flotation concentrate using mesophilic and moderate thermophilic bacteria [J]. Hydrometallurgy,2008, 93( 1/2): 51-56.
[11] SHI Shao-yuan, FANG Zhao-heng. Bioleaching of marmatite flotation concentrate by Acidithiobacillus ferrooxidans [J]. Hydrometallurgy,2004, 75(1/4): 1-10.
[12] FLOWLER T A, CRUNDWELL F K. Leaching of zinc sulfide by thiobacillus ferrooxidans: Experiments with a controlled redox potential indicate no direct bacterial mechanism [J]. Appl Envir Microbiol, 1998, 64(10): 3570-3375.
[13] FLOWLER T A, CRUNDWELL F K. Leaching of zinc sulfide by thiobacillus ferrooxidans: Bacterial oxidation of the sulfur product layer increases the rate of zinc sulfide dissolution at high concentrations of ferrous ion [J]. Appl Envir Microbiol, 1999, 65(12): 5285-5292.
[14] GERICKE M, MULLER H H, STADEN P J, PINCHES A. Development of a tank bioleaching process for the treatment of complex Cu-polymetallic concentrates [J]. Hydrometallurgy,2008, 94(1/4): 23-28.
[15] LUNDSTR?M M, AROMAA J, FORS?N O, HYV?RINEN O, BARKER M H. Leaching of chalcopyrite in cupric chloride solution [J]. Hydrometallurgy,2005, 77(1/2): 89-95.
[16] BALLESTER A, GONZ?LEZ F, BL?ZQUEZ M L. The use of catalytic ions in bioleaching [J]. Hydrometallurgy, 1992, 29(1/3): 145-160.
Foundation item: Project(2004CB619205) supported by the National Basic Research Program of China; Project(50674102) supported by the National Natural Science Foundation of China; Project(2007AA060902) supported by High-tech Research and Development Program of China
Corresponding author: CHEN Song; Tel: +86-731-8830545; E-mail: chensong@126.com
(Edited by YUAN Sai-qian)