
FTIR spectroscopic study of electrochemical flotation of jamesonite-diethyldithiocarbamate system
ZHANG Qin(张 芹)1, HU Yue-hua(胡岳华)2, XU Jing(徐 兢)2, CHEN Tie-jun(陈铁军)1
1. College of Chemical Engineering, Resources and Environment,
Wuhan University of Science and Technology, Wuhan 430081, China;
2. School of Minerals Processing and Bio-engineering, Central South University, Changsha 410083, China
Received 27 June 2005; accepted 31 October 2004
Abstract: The flotation behavior of jamesonite was investigated with sodium diethyldithiocarbamate(DDTC) as a collector. The results show that jamesonite has good floatability from pH 2 to pH 13. The flotation of jamesonite is also dependent on the pulp potential. The potential-pH range for jamesonite flotation is established. The FTIR spectroscopy analysis shows that the major adsorption product of sodium diethyldithiocarbamate on jamesonite is lead diethyldithiocarbamate. The intensity of the FTIR signals of metal diethyldithiocarbamate adsorption on jamesonite and the flotation response of jamesonite are correlated with the pulp potential.
Key words: jamesonite; flotation behavior; floatability diethyldithiocarbamate; electrochemical flotation; FTIR spectroscopy
1 Introduction
Flotation electrochemistry and modern surface analytical techniques have enhanced our understanding of reagent-mineral interaction mechanism. Great progress has been made by applying various electrochemical techniques to investigate the interaction of flotation reagents on sulfide minerals such as galena, pyrite, chalcopyrite and pyrrhotite[1-5]. Leppinen et al[5, 6] studied thionocarbamate adsorption on sulfide minerals (chalcocite, chalcopyrite and pyrite) and ethyl xanthate adsorption on sulfide minerals (chalcocite, chalcopyrite, pyrite and galena) under the conditions of controlled potential by in-situ FTIR. The role of dixanthogen on pyrite flotation has also been revealed by FTIR measurements[7]. The XPS, ARXPS, SIMS , TOP-SIMS and other analytical techniques have been used to study the surface composition of arsenopyrite exposed to oxidizing environments[8], the Cu(Ⅱ)adsorption mechanism on pyrite[9], the interaction of xanthate with galena[10], the adsorption of collectors on pyrite[11], and the hydrophobic and hydrophilic species on galena and pyrite particles in flotation concentrates and tails[12]. Some flotation behaviors of jamesonite were reported by ZHANG et al[13, 14]. However, few reports have been published on the mechanism of jamesonite with a collector. In this paper, the electrochemical flotation behavior of jamesonite was investigated by using DDTC as a collector. The interaction mechanism for the jamesonite and DDTC was studied by FTIR reflection spectroscopic analysis.
2 Experimental
2.1 Materials
The jamesonite mineral used in this investigation was obtained from the number 100 ore body of Dachang Mine, Guangxi, China. The mineral lump was crushed and the jamesonite grains of several millimeters in diameter were handpicked. The sample was ground in a ceramic ball mill and sieved to under 0.1 mm and stored under nitrogen. This sample was analyzed to contain 34.5% Pb, 30.7% Sb, 6.3% Fe, and 21.2% S. The process water for flotation was made of the distilled water and the reagents[16] and is shown in Table 1 with the corresponding pH values.
The reagents for adjusting potential were ammonium persulfate ((NH4)2S2O8) and sodiumdithionite (Na2S2O4). All reagents for tests were analytical grade except the frother butyl ether alcohol being industrial grade. The dosage of the frother was 10 mg/L.
Table 1 Stock solution for Flotation
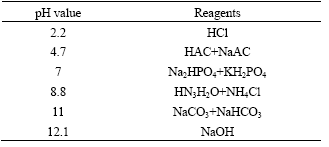
2.2 Flotation tests
The flotation tests were carried out in a micro flotation cell with a 25 mL effective volume. The amount of sample used for each experiment was 2.2 g, which was ultrasonically washed for 5 min to remove any possible oxides on the mineral surface. The washing solution was decanted and a fresh solution of a given pH was added before flotation. The pulp potential in each experiment was adjusted by the reagents and measured before frother addition. The electrodes to measure pulp potential were a saturated calomel electrode(SCE, 0.245 V vs SHE) and a platinum electrode with geometric surface area of 0.28 cm2. The SCE was checked periodically to ensure accurate measurement. Before each test, the platinum electrode was cleaned. The flotation time was 4 min. The flotation recovery(R) was calculated according to the equation as follows: R=[m1/(m1+m2)]×100%, where m1 and m2 were the floated and un-floated fractions, respectively.
2.3 FTIR reflection spectroscopic measurements
The FTIR reflection spectra were obtained using the infrared spectrophotometer (type NEXUS 470). The experimental procedure with powdered samples was as follows. The jamesonite sample of 0.7 g was suspended in a 25 mL buffer solution at the corresponding pH and desired reagent concentrations. After the addition of each reagent, the suspension was stirred for 15 min and then settled for 15 min. The solution was filtered. The treated sample was first dried in vacuum and then used for FTIR reflection spectroscopic measurement.
3 Results and discussion
3.1 Potential-pH region of jamesonite flotation by using DDTC as a collector
Fig.1 Flotation recovery of jamesonite and pulp potential as a function of pH
c (DDTC)=1.0×10-4 mol/L
The effect of pulp pH on the flotation recovery of jamesonite is given in Fig.1. It is shown that jamesonite exhibits good floatability from pH 2 to pH 13 with sodium diethyldithiocarbamate (DDTC) as a collector. The jamesonite flotation recovery was about 90% in all pH value pulp. The pulp potential decreases with increasing pH value.
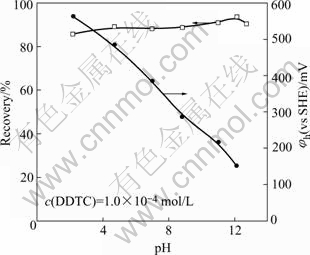
Fig.1 Flotation recovery of jamesonite and pulp potential as function of pH
With fixed pH at 4.7, 8.8 and 12.1 by different buffer solutions respectively, the dependence of jamesonite flotation recovery on pulp potential is shown in Fig.2. It can be shown that the flotation of jamesonite may be possible only in a suitable rang of the pulp potential at corresponding pH. If the upper and lower potential limits for flotation were defined at 50% flotation recovery, a relationship of jamesonite flotation upper(φh(u)) and lower (φh(l)) potential limits can be established and is presented in Fig.3. It can be seen that the flotation of jamesonite may occur only in a range of pulp potentialφh(l)< φh<φh(u) at different pH values. The potential range for flotation increases with increasing pH. In alkali solution, there was a wider range of pulp potential for flotation. For example, at pH 8.8, the optimal potential range for flotation of jamesonite was about 270-570 mV. The maximum flotation occurred at about potential 300 mV.
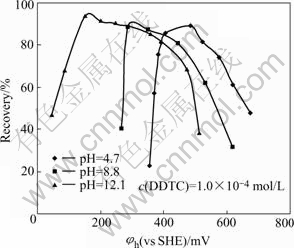
Fig.2 Dependence of jamesonite flotation recovery on pulp potential
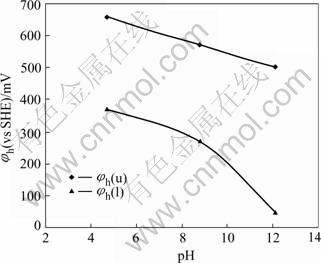
Fig.3 Flotation potential upper and lower limit of jamesonite at different pH values
3.2 FTIR reflection spectra analysis of DDTC adsorption on jamesonite surface
The FTIR reflection spectra of lead diethyldithiocarbamate were reported and shown in Fig.4[16]. Only the region of 750-600 cm-1 is shown, where most of the important vibrations of xanthate functional groups are observed. The FTIR reflection spectra of sodium diethyldithiocarbamate(DDTC) adsorption on jamesonite at pulp pH 7.0 are shown in Fig.5. From Fig.5, it can be seen that the characteristic absorption bands of lead diethyldithiocarbamatehich at about 775, 839, 900, 981, 1 071, 1 138, 1 200, 1 270, 1 351, 1 421 and 1 460 cm-1, indicating that the domi- nant hydrophobic species on jamesonite surface was lead diethyldithiocarbamate. Furthermore, the effect of pulp potential on the adsorption of DDTC on jamesonite was examined and the results are shown in Fig.6. At pH 8.8 the DDTC adsorption on jamesonite is mainly of lead diethyldithiocarbamate independent of the potential in the range of 485-680 mV due to the occurrence of almost the same as lead diethyldithiocarbamate characteristic band. However, the intensity of IR signals was changed at various potential values. The IR signals of the characteristic lead diethyldithiocarbamate peaks (hence lead diethyldithiocarbamate adsorption on jamesonite) decrease with decreasing pulp potential from 485-680mV, accounting for the results of flotation in Figs.2 and 3.
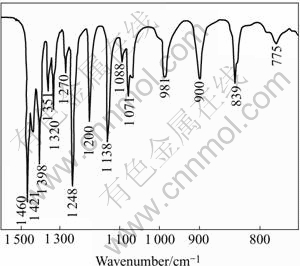
Fig.4 FTIR reflection spectra of lead diethyldithiocarbamate [16]
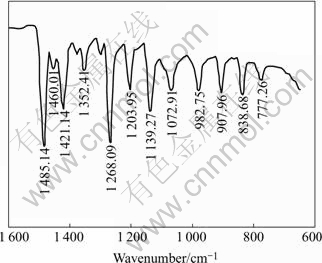
Fig.5 FTIR reflection spectra of jamesonite adsorption DDTC (pH=7.0; c(DDTC)=5×10-3 mol/L; φh=385 mV)
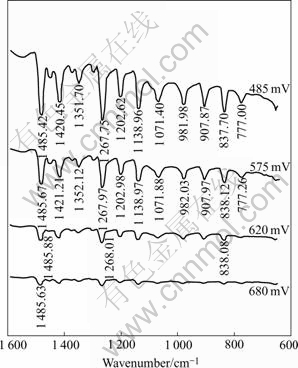
Fig.6 FTIR reflection spectra of DDTC adsorption on jamesonite at different pulp potentials (pH=4.7; c(DDTC)=5×10-3 mol/L)
4 Conclusions
1) Jamesonite has good floatability from pH 2 to pH 13 with sodium diethyldithiocarbamate(DDTC) as a collector.
2) The flotation of jamesonite is dependent on pulp pH and pulp potential. The pulp potential–pH area for flotation of jamesonite is established.
3) Through FTIR reflection spectra, it can be concluded that the major hydrophobic entity of DDTC reaction with jamesonite is lead diethyldithiocarbamate, accounting for the flotation of jamesonite.
References
[1] Buckley A N, Woods R. Appl X-ray photoelectron spectroscopy of oxidized pyrrhotite surfaces(Ⅰ): Exposure to air[J]. Appl Surf Sci, 1985, 22-23: 280-287.
[2] Buckley A N, Woods R. Appl X-ray photoelectron spectroscopy of oxidized pyrrhotite surfaces(Ⅱ): Exposure to aqueousolutions[J]. Appl Surf Sci, 1985, 20: 472-480.
[3] Hamilton I C, Woods R. An investigation of surface oxidation of pyrite and pyrrhotite by linear potential sweep voltammetry[J]. J Electroanal Chem, 1981, 118: 327-343.
[4] Steger H F. Oxidation of sulfide minerals(VII): Effect of temperature and relative humidity on the oxidation of pyrrhotite[J]. Chem Geol, 1982, 35: 281-295.
[5] Leppinen J O, Bssilio C I, Yoon R H. In-suit FTIR study of ethyl xanthate adsorption on sulfide under conditions of controlled potential[J]. International Journal of Mineral Processing, 1989, 26: 259-274.
[6] Leppinen J O, Basilio C I, Yoon R H. FTIR study of thionocarbamate adsorption on sulfide minerals[J]. Colloids and Surface, 1988, 32: 113-125.
[7] Bulut G, Atak S. Role of dixanthogen on pyrite flotation: solubility, adsorption studies and Eh, FTIR measurements[J]. Mineral and Metallurgical Processing, 2002, 19(2): 81-86.
[8] Buckley A N, Walker G W. The surface composition of arsenopyrite exposed to oxidizing environments[J]. Applied Surface Science, 1988-1989, 35: 227-240.
[9] Weisenr C, Gerson A. An investigation of the Cu(Ⅱ) adsorption mechanism on pyrite by ARXPS and SIMS[J]. Minerals Engineering, 2000, 13(13): 1329-1340.
[10] O’Dea A R, PRINCE K E, SMART R S C, GERSON A R. Secondary ion mass spectrometry investigation of the interaction xanthate with galena[J]. International Journal of Mineral Processing, 2001, 61: 121-143.
[11] Nagaraj D R, Brinen J S. SIMS study of adsorption of collectors on pyrite[J]. International Journal of Mineral Processing, 2001, 63: 45-57.
[12] Piantadosi C, Smart R S C. Statistical comparison of hydrophobic and hydrophilic species on galena and pyrite particles in flotation concentrates and tails[J]. International Journal of Mineral Processing, 2002, 64: 43-54.
[13] ZHANG Qin, HU Yue-hua, GU Guo-hua, XU Jing. Selective flotation separation of jamesonite from pyrrhotite by potassium cynide[J]. Journal of Central South University(Science and Technology), 2004, 35(3): 372-375. (in Chinese)
[14] ZHANG Qin, HU Yue-hua, GU Guo-hua, XU Jing. Selective flotation separation of jamesonite from pyrrhotite by lime[J]. Mining and Metallurgical Engineering, 2004, 24(2): 30-32.(in Chinese)
[15] ZHOU Tong-hui. Analytical Chemical Handbook (Ⅱ) [M]. Beijing: Chemistry and Industry Press, 1997. 335-349. (in Chinese)
[16] Pouchert C J. The Aldrich Library of Infrared Spectra[M]. Chicago: Aldrich Chemical Co Inc Press, 1970.
Foundation item: Project(50234010) supported by the National Natural Science Foundation of China
Corresponding author: ZHANG Qin; Tel: +86-27-68862204; E-mail: zq81219074@163.com
(Edited by LI Xiang-qun)