
Rheo-diecasting of AZ91D magnesium alloy by taper barrel rheomoulding process
YANG Liu-qing(杨柳青)1, KANG Yong-lin(康永林)1, 2, ZHANG Fan(张 帆)1,
DING Rui-hua(丁瑞华)1, LI Jiong(李 炯)3
1. School of Materials Science and Engineering, University of Science and Technology Beijing, Beijing 100083, China;
2. State Key Laboratory for Advanced Metals and Materials, University of Science and Technology Beijing,
Beijing 100083, China;
3. Beijing Guangling Jinghua Science and Technology Co. Ltd., Beijing 102211, China
Received 3 August 2009; accepted 11 January 2010
Abstract: A self-developed taper barrel rheomoulding (TBR) machine was introduced, and the rheo-diecasting process was implemented by combining TBR machine with the high pressure die casting (HPDC) machine. Microstructural characteristics of the rheo-diecasting components were investigated at different rotation speeds. Flow characteristics and microstructural evolution of the semi-solid slurry during the rheo-diecasting process were analyzed and the mechanical properties of the rheo-diecasting components were studied. The experimental results show that the process is able to obtain such components in which the primary α-Mg particles are fine, nearly spherical and uniformly distributed in the matrix. When the rotation speed of internal taper barrel is 700 r/min, the primary α-Mg particles get a mean diameter of about 45 μm and a shape factor of about 0.81. The magnesium alloy melt has complex stirring-fixed flow characteristics when flowing in TBR machine. Compared with conventional die-casing process, the rheo-diecasting process can improve the mechanical properties of components; especially, the elongation is improved by 80%.
Key words: AZ91D magnesium alloy; rheo-diecasting; microstructure; flow characteristics; mechanical properties
1 Introduction
Thixoforming and rheoforming are two basic methods of semi-solid metal (SSM) forming, and each obviously has its own characteristics. Due to the widespread applications, applicability to many processing methods, short process, compact casting structure and high dimensional accuracy, rheoforming received much attention. Many scholars abroad carried out a great deal of work on theories, experiments, techniques and equipment developments and made rapid progress, which made rheoforming a new focus in SSM processing field[1-3]. However, in order to obtain uniform and fine semi-solid slurry microstructure, rheoforming need to control the solidification of the alloy melt accurately in a relatively short time, thus the processing control is obviously difficult, which hinders the large-scale industrial application of this technology. Consequently, one of the main research directions of rheoforming is to develop new semi-solid equipments and techniques with low cost and accurately-controlled slurry microstructure[4]. Combined with semi-solid slurry preparation technology, a rheo-diecasting process was developed which keeps the characteristics of rheoforming and high efficiency and low cost of die-casting, and it assuredly has a great industrial application value. The representative rheo-diecasting process mainly includes the twin-screw rheomoulding process developed by FAN[5] and JI et al[6], but so far this technology has not achieved large-scale industrial application. Rapid semi-solid slurry preparation as well as transportation is still the bottleneck that restricts the rapid development of rheo-diecasting process.
In this work, the widely applied AZ91D magnesium alloy was used as experimental material. Using self- developed taper barrel rheomoulding (TBR) machine combined with TOYO BD-900V4-T high pressure diecasting (HPDC) machine, the integrated rheo-diecasting process from semi-solid slurry preparation to die-casting is successfully realized. Microstructural characteristics of the rheo-diecasting components at different internal taper barrel rotation speeds were investigated. The flow characteristics and microstructural evolution of the semi-solid slurry of AZ91D alloy during the rheo-diecasting process were researched; and the mechanical properties of the rheo-diecasting components were studied as well.
2 Experimental
2.1 TBR process
The integrated TBR process is illustrated in Fig.1. It consists of a semi-solid preparing appliance, a driving system, an elevating system, a semi-solid conveying appliance, a gas protection system, a temperature control system and a die-casting system. The semi-solid preparing appliance is the core of the TBR process, which consists of internal and external taper barrel. The driving system mainly consists of the motor and driving bevel gears, to make the internal taper barrel rotate at a high speed by the driving bevel gears and to shear and stir the magnesium alloy melt. The function of the elevating system is to adjust the gap between the internal and external taper barrel. The objective of the semi-solid conveying appliance is to transport the prepared semi-solid magnesium alloy slurry to the die-casting machine promptly at a constant temperature. The gas
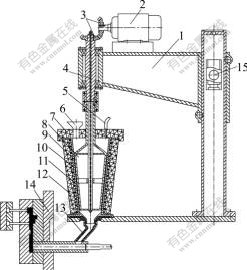
Fig.1 Schematic diagram of integrated TBR process: 1— Elevating system; 2—Motor; 3—Driving bevel gears; 4—Argon channel; 5—Argon channel; 6—Funnel; 7—Thermal cover; 8—Internal taper barrel; 9—External taper barrel; 10— Heating elements; 11—Cooling channels; 12—Muff; 13— Semi-solid conveying appliance; 14—Die-casting mould; 15— Column
protection system is to avoid oxidative burning of the semi-solid slurry during preparing and conveying. The temperature control system includes heating and cooling channels, which assures a constant temperature in the course of SSM slurry preparation. The die-casting system is composed of a HPDC machine and a die-casting mould, the purpose of which is to form the semi-solid slurry inside and finally acquire the near-net shape components. Both the preparation and transportation of the semi-solid slurry were achieved by self-gravity of the melt under the integrated TBR process.
2.2 Experimental material and procedures
The experimental material is commercial high purity AZ91D magnesium alloy ingot. Liquidus and solidus of the alloy are 595 ℃ and 470 ℃, respectively. The chemical composition of the magnesium ingot was tested by ARL3460 Direct Reading Spectrometer and the result is presented in Table 1.
Table 1 Chemical composition of AZ91D magnesium alloy ingot (mass fraction, %)
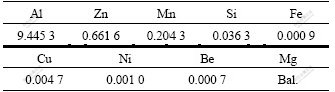
AZ91D ingot was put into a preheating furnace which was preheated at 150 ℃ for 1 h, and then it was conveyed to a melting furnace in which the temperature reached 680 ℃ and the ingot melted. Covering agent was used during the melting process to protect the magnesium alloy melt from oxidative burning. Then, the liquid alloy was poured into TBR machine the parameters of which were set specifically in advance. During passing through the gap of taper barrel, the alloy melt experienced intense shearing during solidification because of the shear stress field caused by relatively high-speed rotation between the internal and external taper barrel. The shear time was approximately 5-8 s. Finally, the prepared semi-solid slurry was transferred into a TOYO BD-900V4-T HPDC machine via slurry conveying appliance in order to carry out die-casting forming. Both the preparation and transportation of the semi-solid slurry were carried out in a closed environment and Ar was applied to prevent the magnesium alloy melt from oxidative burning. The die-casting process parameters in the experiment were as follows: clamp force 9 000 kN, casting pressure 75 MPa, casting speed 0.35-3.5 m/s and mould temperature 200 ℃. The experimental die-casting mould was selected from architecture scraper moulds with a thick wall (about 7 mm) which was fit for rheo-diecasting process. Fig.2 shows the photograph of the selected rheo-diecasting architecture scraper and sampling position. Specimens for tensile tests were cut from the scraper referring to national standard GB 6397—86.
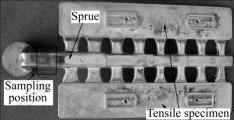
Fig.2 Photograph of rheo-diecasting architecture scraper and sampling position
The samples were cut from the sprue of the architecture scraper (illustrated in Fig.2), followed by grinding and polishing. The polished samples were then etched by 4% (volume fraction) nitric acid alcohol and flushed with hot water, and finally blew dried. A NEOPHOT 21 optical microscope and a Cambridge S-360 scanning electron microscope were utilized for microstructural observation and analysis. The tensile test was carried on a MTS810 material testing machine referring to national standard GB/T 228—2002, and the strain rate was 1.0 mm/min. The influence of processing parameters on the primary α-Mg particles size and the shape factor was investigated using Image Tool 3.0 image analysis software. The diameter of primary α-Mg particles is symbolized by the equivalent area diameter Deq, and the shape factor is recorded as F. Therefore, the primary α-Mg particles are even and round when F is close to 1. Specific calculation formulae are as follows [7-8]:
(1)
(2)
where An and Pn are the area and perimeter of the nth primary α-Mg particle, respectively; and n is the number of particles.
3 Results and discussion
3.1 Microstructure of rheo-diecasting components
The microstructure of conventional die-casting components is observed to compare with that of semi-solid rheo-diecasting components. Fig.3 shows the microstructure of the die-casting components poured at 680 ℃, which indicates that the primary α-Mg particles of conventional die-casting components mainly consist of coarse and developed dendrites. Fig.4 presents the optical microstructure of rheo-diecasting components at different rotation speeds of the internal taper barrel, while other corresponding processing parameters include pouring temperature 680 ℃, shearing temperature 590 ℃ and taper barrel gap 3 mm. It reveals that all the semi-solid microstructures consist of fine granular and spheroidal primary α-Mg particles, while dendritic primary phase is not found, which is obviously different from the morphology of the primary α-Mg particles of conventional die-casting components. The results show that the rheo-diecasting process is capable of obtaining primary α-Mg particles characterized by fine granular microstructure.
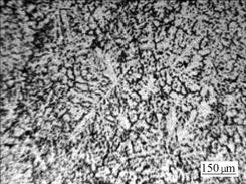
Fig.3 Optical micrographs of conventional die-casting AZ91D alloy
The corresponding processing parameters and microstructural characteristics are summarized in Table 2. When the taper barrel speed is 300 r/min, the component reflects an unevenly distributed structure where the primary α-Mg particles appear in rosette and ellipsoid. The mean particle diameter is about 63 μm and the shape factor is 0.46. When the taper barrel speed reaches 500 r/min, the primary α-Mg particles present relatively uniform distribution on the matrix where major primary α-Mg particles are ellipsoidal with a mean particle diameter of 54 μm and a shape factor of 0.62. As the taper barrel speed increases to 600 r/min, microstructure of the components turns out a great change, that is, the primary α-Mg particles become finer and nearly spherical, and the mean size is 46 μm. Moreover, the particles uniformly distribute on the matrix in a shape resembling sphere and the shape factor is 0.78. The distribution of the primary α-Mg particles gets more homogeneous at 700 r/min compared with that at 600 r/min, while the particle size and shape factor does not
change much. Based on the above analysis, it is concluded that the high rotation speed of the internal taper barrel influences the undercooling melt and makes the temperature and concentration field uniform throughout the entire volume of the melt and the primary α-Mg particles grow spherically. Meanwhile, the high rotation speed leads to the transformation of partial primary α-Mg particles from dendrite to non-dendrite. It is clear that increasing the intensity of turbulence (namely rotation speed of the internal taper barrel) makes the primary α-Mg particles finer substantially and the spherical shape more regular and uniform[9].
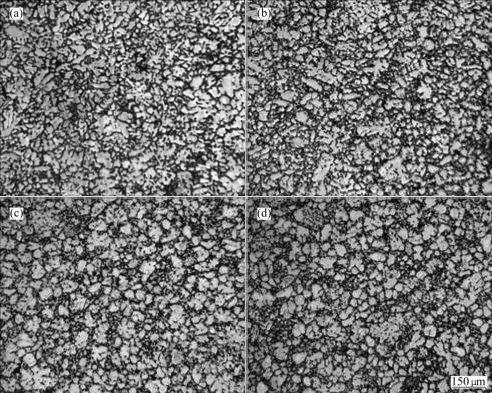
Fig.4 Optical micrographs of rheo-diecasting AZ91D alloy at different rotation speeds: (a) 300 r/min; (b) 500 r/min; (c) 600 r/min; (d) 700 r/min
Table 2 Processing parameters and microstructural characteristics of rheo-diecasting AZ91D alloy

Fig.5 presents the SEM micrograph of the rheo-diecasting components obtained at a pouring temperature of 680 ℃, shearing temperature of 590 ℃, taper barrel gap of 3 mm and internal taper barrel speed of 300 r/min. The nearly spherical gray particles are primary α-Mg phases, and the white reticular phase
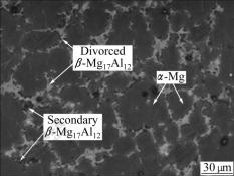
Fig.5 SEM images rheo-diecasting components
along the boundaries of the primary phase is the β-Mg17Al12 divorced eutectic resulted from non-equilibrium solidification. Besides, the black phase around the divorced β phase is the secondary β phase precipitated from primary α-Mg particles, the precipitation of which is controlled by phase transformation law[10-11].
3.2 Flow characteristics of semi-solid slurry
Fig.6 illustrates the flow characteristics of the AZ91D alloy melt inside TBR machine during the slurry preparation. The flow of the alloy melt is achieved by the rotation speed of internal taper barrel and self-gravity of the melt. Because of the high rotation speed and adhesion action between the melt and exine of the internal taper barrel, the alloy melt gets a trend which makes its rotation direction the same as the internal taper barrel when flowing downwards the gap between the internal and external taper barrel. Meanwhile, there exist some angled grooves on the exine of the internal taper barrel and the wall of the external taper barrel, which increases the friction of the melt received from TBR machine. As a result, particles carry out self-rotation at the same time as rotating along grooves on the internal taper barrel. Therefore, the alloy melt has complex stirring-mixed flow characteristics when flowing in the TBR machine. Under the high-speed rotation of the internal taper barrel, the alloy melt not only flows from above to below in the TBR machine but also rotates along the grooves on the internal taper barrel, and meanwhile the primary α-Mg particles in the melt self-rotate at a certain speed.
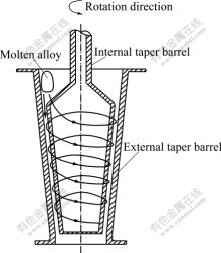
Fig.6 Schematic diagram of semi-solid slurry in TBR machine
3.3 Microstructure evolution
Based on a large number of experiments, a conclusion is obtained that the integrated device enhances the heterogeneous nucleation tremendously under the comprehensive action of taper barrel cooling and its high intensity of shearing. Many kinds of spheroidizing mechanisms cause the formation of semi-solid magnesium alloy, which mainly include the dendrite-suppressed growth mechanism and the dendrite arm fracture spheroidizing mechanism.
When the alloy melt flows through the interior of TBR machine during the semi-solid slurry preparation, high intensity of turbulence creates a relatively uniform temperature field and composition field inside. Meanwhile, under the strong cooling effect that the internal and external taper barrel take on the alloy melt, the melt with a certain superheat cools to the solidification temperature in a short time. According to the “big bang” nucleation theory[12], lots of effective nucleation sites which are potential nucleus under appropriate conditions are formed inside the melt. Besides, part of particles forming on the taper barrel wall dissociate into the melt under a high rotation speed of internal taper barrel, so in TBR process, the intense shearing and stirring increase the density of primary α-Mg particles tremendously. Since the temperature field and composition field are uniform, and the coefficient of thermal diffusion is much higher than that of solute diffusion, it is presumed that the morphological evolution dynamics of primary α-Mg particles is mainly controlled by the solute diffusion and solid-liquid interfacial energy. It is believed that the growing granular particles can self-rotate, which leads to a more uniform distribution of temperature and composition around the particles urging them to grow, and this helps to form spherical semi-solid primary particles[13-14]. In a perspective of solidification morphology, particle spheroidization is substantially the result of solid-liquid interface change during the transformation of steady- state to non-steady, while the steady-state maintaining course. In brief, under pure diffusion conditions, steadiness of the solid-liquid interface can be described with the classic Mullins-Sekerka theory[15]:
(3)
where Tm is the alloy melting point, Γ is the surface tension constant, m is the liquidus slope, ω is the vibration frequency, ω* is the fluctuation frequency of medium along the solid-liquid interface, ν is the interface velocity, D is the solute diffusion coefficient, and k0 is the solute equilibrium partition coefficient. GL and GC are the temperature gradient and concentration gradient when δ=0, respectively. Positive or negative of S(ω) corresponds with the growth or decay of interference amplitude and it decides the steadiness of solid-liquid interface. The classic Mullins-Sekerka theory is based on single particle, that is, the growth of one particle is not influenced by others. However, in TBR process, the primary α-Mg particles have a high grain density and a short grain distance, and the composition field of one particle would stack on that of adjacent particles, which makes the concentration gradient around each particle decline and further results in the decrease of the destabilizing effect caused by concentration gradient. Therefore, high particle density is helpful to the steadiness of solid-liquid interface and keep the particles growing spherically.
Besides, at the stage of semi-solid slurry preparation, part of nuclei in the undercooling alloy melt distribute on the wall of the taper barrel and grow as dendrites which would be sheared and stirred by the internal taper barrel, resulting in the primary dendrites fragmenting and dissociating into the melt. The melt flow caused by stirring creates a uniform temperature in every direction for dissociated dendrite, reduces the concentration gradient of solid-liquid interface, decreases the composition undercooling, consequently the particles grow in a spherical shape. The fragmentation and spheroidization of dendrites can be divided into two stages: the formation and spheroidization of the dissociative dendrites. That is, nucleation first occurs on the wall of the taper barrel and grows to dendrites, then the dendrites break up to dissociative dendrites under the shearing and stirring of the internal taper barrel, finally, the dissociative dendrites become spherical. Under a certain shear rate, the shearing and stirring of the internal taper barrel would promote the formation of globular particles. Yet, when the shear rate is high, the globular particles would diminish under the suppression of such shearing and stirring[16].
3.4 Mechanical properties of rheo-diecasting components
Table 3 lists the mechanical properties of AZ91D magnesium components under different processes. The ultimate tensile strength (UTS) of rheo-diecasting is higher than that of thixocasting, and both the yield strength (YS) and elongation rise. Compared with the twin-screw rheomoulding techniques, the rheo-diecasting components have a lower UTS and elongation, but the YS does not change much. Conventional die-casting was performed to compare with rheo-diecasting using the same HPDC machine and die-casting mould. The results show that the YS of the rheo-diecasting components was nearly the same as conventional die-casting, while the UTS of the former increases, and the elongation improves by 80%.
Factors that result in the degeneration of mechanical properties in die-casting process mainly involve internal porosity, micro-shrinkage, hot crack, microstructural non-uniformity and so on. In conventional die-casting process, due to the high pouring temperature, the coarse dendrite and micro-shrinkage or shrinkage would form during the solidification in the die-casting mold cavity,
Table 3 Mechanical properties of rheo-diecasting components under different processes
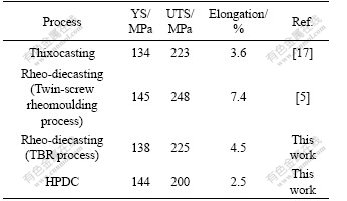
which leads to microstructural non-uniformity and inferior compactness inside the components, thus worsens the mechanical properties. While in rheo-diecasting process, pouring temperature is not much high, and the melt experiences intense shearing and stirring before pouring, which is helpful to form uniform structure and high compactness, and results in the rise of the tensile strength and elongation. The reasons why the mechanical properties of rheo-diecasting parts are inferior to those of the twin-screw rheomoulding ones may attribute to the short shearing time and slow shearing rate, and this results in the microstructural non-uniformity. It is believed that mechanical properties of die-casing components have a strong dependence on the microstructural morphology[11]. The unique microstructure feature that primary α-Mg globules uniformly distribute on the matrix may be beneficial for the components to obtain good mechanical properties.
4 Conclusions
1) A self-developed TBR machine is introduced. Combining TBR machine with HPDC machine, the integrated rheo-diecasting process from semi-solid slurry preparation to die-casting is implemented successfully. The rheo-diecasting process is able to obtain the components in which the primary α-Mg particles are fine, nearly spherical and uniformly distributed on the matrix.
2) Microstructural characteristics of the rheo-diecasting components at different rotation speeds are investigated. As the speed varies from 300 to 600 r/min, the primary α-Mg particles in the rheo-diecasting components become finer and nearly spherical, with the mean particle diameters varies from 63 to 46 μm and the shape factor from 0.46 to 0.78. Distribution of the primary α-Mg particles is more homogeneous at 700 r/min than that at 600 r/min, but the particle size and the shape factor does not change much.
3) Compared with conventional die-casing process, the rheo-diecasting process successfully improves mechanical properties of components; especially, the elongation is improved by 80%. More experiments should be taken to improve and optimize the rheo-diecasting process.
References
[1] KANG Yong-lin, MAO Wei-min, HU Zhuang-qi. Theory and technology of semi-solid metal forming processing [M]. Beijing: Science Press, 2004. (in Chinese)
[2] FLEMINGS M C. Behavior of metal alloys in the semisolid state [J]. Metallurgical Transactions B, 1991, 22B: 269-293.
[3] HAGA T, KAPRANOS P. Simple rheocasting processes [J]. Journal of Materials Processing Technology, 2002, 130/131: 594-598.
[4] MAO Wei-min. Semi-solid metal forming technology [M]. Beijing: China Machine Press, 2004. (in Chinese)
[5] FAN Z. Development of the rheo-diecasting process for magnesium alloys [J]. Materials Science and Engineering A, 2005, 413/414: 72-78.
[6] JI S, FAN Z, BEVIS M J. Semi-solid processing of engineering alloys by a twin-screw rheomoulding process [J]. Materials Science and Engineering A, 2001, 299: 210-217.
[7] TZIMAS E, ZAVALIANGOS A. A comparative characterization of near-equiaxed microstructures as produced by spray casting, magnetohydrodynamic casting and the stress induced, melt activated process [J]. Materials Science and Engineering A, 2000, 289: 217-227.
[8] LIU Zheng, MAO Wei-min, ZHAO Zhen-duo. Semi-solid A356 alloy slurry prepared by a new process [J]. Acta Metallurgica Sinica, 2009, 45(4): 507-512. (in Chinese)
[9] XU Yue, KANG Yong-lin, WANG Zhao-hui, DONG Wen-chao, LIU Jin-wei. Preparation of semi-solid slurry of AZ91D Mg alloy [J]. Special Casting and Nonferrous Alloys, 2004, (5): 12-14. (in Chinese)
[10] LIU Zheng, ZHANG Kui, ZENG Xiao-qin. Theoretical basis and application of magnesium based light alloys [M]. Beijing: China Machine Press, 2006. (in Chinese)
[11] DU X H, ZHANG E L. Microstructure and mechanical behaviour of semi-solid die-casting AZ91D magnesium alloy [J]. Materials Letters, 2007, 61: 2333-2337.
[12] CHALMERS B. Principles of solidification [M]. New York: John Wiley & Sons, 1964.
[13] GUO Hong-min, YANG Xiang-jie, LUO Xue-quan. Formation of grain refined and non-dendritic microstructure of an aluminum alloy under angular oscillation [J]. Journal of Alloys and Compounds, 2009, 482: 412-415.
[14] GUAN Ren-guo, WEN Jing-lin, WANG Shun-cheng, Liu Xiang-hua. Microstructure behavior and metal flow during continuously extending-extrusion forming of semisolid A2017 alloy [J]. Transactions of Nonferrous Metals Society of China, 2006, 16(2): 382-386.
[15] HU Han-qi. Principles of metal solidification [M]. Beijing: China Machine Press, 2000. (in Chinese)
[16] GUAN Ren-guo, MA Wei-min. Theory and technology of semi-solid metal forming [M]. Beijing: Metallurgical Industry Press, 2005. (in Chinese)
[17] CZERWINSKI F, ZIELINSKA-LIPIEC A, PINET P J, OVERBEEKE J. Correlating the microstructure and tensile properties of a thixomolded AZ91D magnesium alloy [J]. Acta Materialia, 2001, 49: 1225-1235.
Foundation item: Project(2006CB605203) supported by National Basic Research Program of China; Project(2006AA03Z115) supported by the National High-tech Research and Development Program of China; Project(2006BAE04B09-4) supported by the National Key Technologies R&D Program of China during the 11th Five-Year Plan Period
Corresponding author: KANG Yong-lin; Tel: +86-10-62332983; E-mail: kangylin@mater.ustb.edu.cn
DOI: 10.1016/S1003-6326(09)60243-0
(Edited by FANG Jing-hua)