
Properties of electric brushes made with Cu-coated graphite composites and with copper powders
HU Zhong-liang (胡忠良), CHEN Zhen-hua (陈振华), XIA Jin-tong (夏金童), JING Guo-yun (丁国芸)
College of Materials Science and Engineering, Hunan University, Changsha 410082, China
Received 15 July 2007; accepted 10 September 2007
Abstract: Cu-coated graphite composites were prepared by an electroless plating method, and the composites were examined by scanning electron microscopy (SEM). The copper content of the composites was measured to reach 73% (mass fraction). Brush samples made with composites contents of 10%, 15% and 20% and with copper powders content of 11% were obtained. The results show that the resistivity of the brush samples decreases and hardness becomes higher with increasing composites content. Copper is dispersively distributed in the brush samples made with the composites, whereas copper agglomerates in Cu-graphite mechanical mixture. For the same Cu content, Cu-coated graphite composites possess lower resistivity and higher hardness than Cu-graphite mechanical mixture. A new type of electric brush was obtained by using Cu-coated graphite composites, the wear and durability tests carried out on a HGH5435M120 AC motor indicate that its service life reaches 250 h. The electric brushes possess relatively low resistivity (275 μΩ·m) and good wear resistance, so they are very suitable for high-speed micromotors.
Key words: electric brush; Cu-coated graphite; electroless plating; wear
1 Introduction
China motors industry has developed rapidly in recent years and many new types of micromotors with a high rotational speed have been invented[1]. The brushes fixed in the motors must have excellent commutation ability. Otherwise, large commutation sparks are produced, thus brushes wear quickly and their service life is shortened. To solve the problem, high-resistivity brushes have been developed to match the motors[2], they can restrain commutation sparks due to their high contact resistance. But their resistivity is often higher than 800 mW?m[3], which induces a high contact voltage drop between the brush and commutator. This will reduce the power efficiency of motors and even affect their normal operation.
In order to develop brushes with good wear resistance and relatively low resistivity, the materials with good conductibility and wear resistance should be utilized. Copper-graphite composites possess the properties of copper, i.e. excellent thermal and electrical conductivities, and properties of graphite, i.e. solid lubricating and small thermal expansion coefficient, so they have been extensively used as materials for electric brushes[4]. However, copper poorly wets graphite, so the interface strength of copper-graphite is very weak. Some studies have showed that the copper-graphite interface cohesion has much to do with the wear and mechanical properties of composites[5-6].
Copper coated graphite composites were developed in 1947. The surface treatment for graphite improves interfacial adhesion of composites. Due to their excellent properties and potential use, numerous studies have been conducted on them[7]. Earlier literatures investigated the optimal processing parameters of preparing the composites[8]; newer research focused on their properties and their new application as functional materials[9-11]. Electroless plating has been proved to be a good method for preparing metal-coated composites[12-15].
In this study, Cu-coated graphite composites were prepared by the plating method. Due to their excellent conductibility and wear resistance, we attempted to extend their application to electric brushes for high-speed micromotors. For comparison, the brush samples were also prepared by using copper powders.
2 Experimental
2.1 Preparation and characterization of copper- coated graphite composites
Cu-coated graphite composites were prepared by an electroless plating method. First, surface hydrophilization of graphite powder (Flake graphite content is greater than 99% and its size is less than 75 mm) was boiled in 20% NaOH solution for 20 min, and then washed with water until being neutral. Second, graphite powders were oxidized by 20% HNO3 solution at boiling temperature for 20 min, and then washed with distilled water until being neutral and dried. Third, the graphite powders were added under magnetic stirring into a fresh sensitizing solution that included 20 g/L SnCl2 and 4% HCl. After 20 min stirring, the sensitized graphite powders were filtered and washed until being neutral with distilled water. Fourth, the graphite powders were activated for 30 min in an aqueous solution of 0.001 4 mol/L PdCl2-0.25 mol/L HCl. Finally, the activated graphite powders were introduced in the electroless bath for 2 h. Thus Cu-coated graphite powders were obtained. The compositions of the electroless bath and the reaction conditions were listed in Table 1.
Table 1 Bath compositions and operating condition for electroless plating
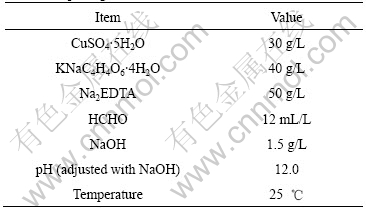
The reagents used in the experiments were of analytical grade purity.
The morphology of Cu-coated graphite composites was observed by SEM. The copper content in the composites was measured by chemical oxidation- reduction method and calculated according to Eqn.(1).
(1)
where m is the total mass of Cu-coated composite sample, m1 is the mass of copper. The value of m1 was gotten by oxidation and reduction method. The composites were heated to 700 ℃ for 6 h until the graphite grains were completely oxidized, then they were put into reduction oven at 350 ℃ in H2 atmosphere for 2 h till the copper oxide was reduced to copper. After cooling, the final mass of reaction product was m1.
2.2 Preparation of brush sample
Manufacturing process for electrical brushes is shown in Fig.1 and Fig.2. In first step, graphite powders, coke powders and modified pitch were mixed at 180 ℃, then tabletted at 180 ℃, milled and sized by <74 μm sieve. Thus crude graphite powders were obtained. In second step, crude graphite powders, Cu-coated graphite powders, MoS2 and additive were mixed, molded at 100 MPa, baked at 750 ℃ and finally machined to brushes.
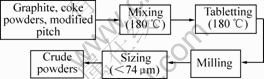
Fig.1 Preparation process for crude powders
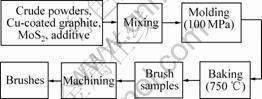
Fig.2 Manufacturing process for high-resistivity electric brushes
2.3 Wear and durability tests for carbon brushes
Wear and durability tests were carried out on a HGH5435M120 AC motor. The motor is commercially available and its main performance parameters are listed in Table 2. All tests were conducted when the motor was with free load and carried out under the same condition. The relative humidity is 50%, the ambient temperature is 25 ℃ and the spring loads are 5 N/cm2. The schematic of the test motor is shown in Fig.3.
During wear and durability tests, the bulk temperature of brushes was measured by a TM6801A digital temperature meter with precision of 0.5 ℃, and the place where the temperature was measured was within 3 mm adjacent to the worn surface of brushes. The commutation sparks were measured according to China motor standard GB—755—65 and the worn surfaces of brushes were characterized by SEM.
Table 2 Parameters of motor
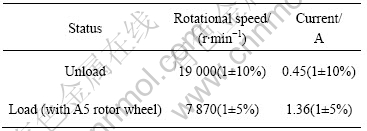
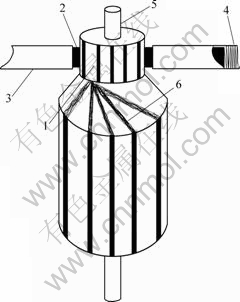
Fig.3 Scheme of test motor: 1 Commutator bar; 2 Brush; 3 Brush holder; 4 Contact force spring; 5 Bearing; 6 Winding
3 Results and discussion
3.1 Analysis of Cu-coated graphite composites
SEM images of original graphite powders and Cu-coated graphite powders are shown in Fig.4 and Fig.5. The comparison result shows clearly that the surface of graphite powder is uniformly and compactly coated by a layer of copper particles. The copper content in the composites is 73%, which is determined by the chemical oxidation-reduction method. These results indicate that it is practicable to form a complete coating layer on graphite by the above-mentioned electroless plating process.
3.2 Effects of Cu-coated graphite on properties of brush samples
Cu-coated graphite composites possess excellent friction and wear properties[5]. In this study we investigated the properties of several brush samples made with different contents of Cu-coated graphite. To compare the composites with Cu-graphite mechanical mixture, we added brush sample 4 made with Cu powders (The copper purity is greater than 99.5% and its size is less than 75 mm). All compositions of brush samples are listed in Table 3 and the properties of brush samples are listed in Table 4.
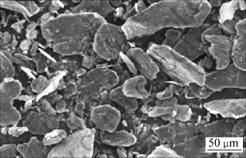
Fig.4 SEM image of original graphite powders
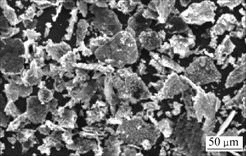
Fig.5 SEM image of Cu-coated graphite powders
Table 3 Compositions of brush samples (mass fraction, %)
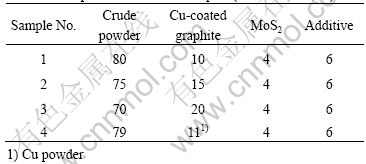
Table 4 Properties of brush samples
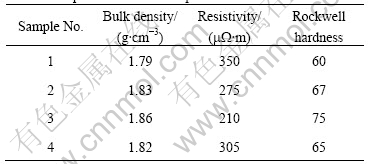
From Table 4 one can clearly see that bulk density and Rockwell hardness increase regularly with increasing Cu-coated graphite content while resistivity shows an opposite tendency. These results can be explained by that the Cu-coated graphite composites possess a good electrical conductivity and a high density.
It is worth noting that the bulk density and Rockwell hardness of brush sample 2 are higher than those of brush sample 4, that is, brush sample 2 possesses a better conductivity than brush sample 4. Because these two brush samples have almost the same element contents, the difference of properties between the two samples should be caused by the difference of bonding modes between copper and graphite matrix. Micrographs of the fracture surfaces of brush sample 4 and brush sample 2 are shown in Fig.6 and Fig.7, respectively. Fig.6 shows that copper powder agglomerates and is not evenly distributed. There is no interfacial bonding between copper and graphite particles due to the presence of gaps, and the brush sample has a higher porosity. On the other hand, SEM image of brush sample 2, as shown in Fig.7, indicates that copper powders are well distributed and there is a good contact between copper and graphite particles, which leads to a lower porosity. The better interfacial bonding for Cu-coated composites makes brush sample 2 possess a better conductivity and a higher hardness. A little higher bulk density of brush sample 2 is due to its compact structure.
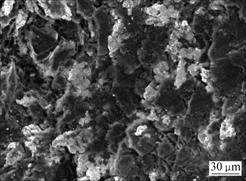
Fig.6 SEM image of fracture surface of brush sample 4
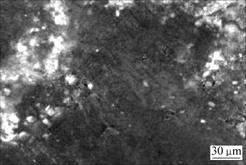
Fig.7 SEM image of fracture surface of brush sample 2
3.3 Wear and durability tests and analysis for brush samples
The wear and durability tests for four kinds of brushes were conducted on an HGH5435M120 AC motor. The wear rate and some related experimental phenomena are listed in Table 5.
From Table 5 one can see that brush sample 2 has the smallest wear rate, spark grade and the lowest bulk temperature, whereas brush sample 4 has the biggest wear rate, spark grade and the highest bulk temperature.
It is widely recognized that the wear rates of brushes are directly related to the commutation sparks, the state of the lubricant film and the bulk temperature[16-17]. For high-speed micromotors, some investigations have proved that electric wear due to commutation sparks dominates the brushes wear[16]. Sparks can damage the lubricating film and make the worn surfaces of brushes and commutators rough, thus they make the brushes wear increase much.
Because brush sample 2 and brush sample 4 have nearly the same element contents, the wear property difference between them is apparently due to the excellent properties of Cu-coated graphite. In brush sample 2, the copper encapsulates the graphite and dispersively distributes in the matrix, while the copper and graphite in brush sample 4 are just mechanical mixtures. The structure of brush sample 2 make it be of good contact performance, thus small sparks are induced. But for brush sample 4, the situation is completely opposite, worse contact between brush and commutator leads to big sparks.
From Table 5 one can also see that the content of Cu-coated graphite has much to do with the wear property of brushes. When the content of Cu-coated graphite is low, the good wear property of Cu-coated graphite cannot fully display and the oxide film can not well form, thus the brushes wear is large. With increasing the content of Cu-coated graphite, although the resistivity of brushes decreases, MoS2 in brushes can form a lubricating film that can restrain sparks, and Cu-coated graphite displays its wear property more fully,so the wear rate becomes less. But further increment of Cu-coated graphite will make the contact resistance too small to induce short-circuit current, which can increase commutator sparks, thus the wear of brushes increases. Based on the above analysis, brush sample 2 containing an appropriate content of Cu-coated graphite has the lowest sparks and wear rate.
Table 5 Wear tests results and related experimental phenomena for brush samples
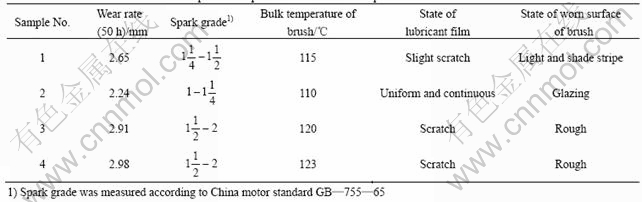
From Table 5 one should pay attention to an interesting relationship between the wear rate and the bulk temperature of brushes. The lower the bulk temperature is, the lower the wear rate and the longer the service life. According to the theories of SAVAGE and LANCASTER[18-21], the wear of brushes is closely related to the relative water vapour pressure of p/p0, p is the absolute water vapour pressure, while p0 is the water saturated pressure at the bulk temperature of brushes. Under high relative pressure, the water vapor molecules tend to cover the exposed graphite surface by forming transient monolayers. By this way, the free surface energy is lowered, which reduces the cohesion and adhesion between the contact areas and makes the friction and wear decrease. Under low relative pressure, the graphite can not obtain its saturated surface and the adsorption water film only covers a part of graphite surface. So the free surface energy is high, the contact molecules between graphite and copper may seize, which makes the friction and the wear of brushes increase. Our durability tests were carried out in a relative humidity of 50%, but in our electrical brush-rotor systems, the bulk temperature of brushes is over 100 ℃ and the value of p/p0 is low. With increasing the bulk temperature, the value of p/p0 decreases, so the wear of brushes becomes larger.
The wear and durability tests for brush sample 2 indicate that the service life reaches 250 h and the resistivity is relatively low (275 mW?m). The brushes made with Cu-coated graphite composites are very suitable for high-speed micromotors.
4 Conclusions
1) Cu-coated graphite particles were prepared by an electroless plating method. The copper is uniformly and compactly distributed on the surface of graphite. The copper content reaches 73% in the composites.
2) With increasing Cu-coated graphite composites, the resistivity of brush samples decreases, whereas the bulk density and hardness increase.
3) The copper is dispersively distributed in matrix of brush samples made with Cu-coated graphite, whereas the copper agglomerates in Cu-graphite mechanical mixture. Brushes made with 15% Cu-coated graphite content are of better wear properties.
4) The electric brushes made with Cu-coated graphite possess low resistivity and good wear resistance. The service life of the brushes reaches 250 h.
References
[1] ZHAO Ji-cun, ZHOU Wei, SUN Qiong, DUAN Jun, ZHANG Rui. Preparation of brushes for new types of micromotors[J]. Micromotors, 2000, 33(4): 43-44. (in Chinese)
[2] ROBERGE R. Carbon brush performance and application in the pulp and paper environment[J]. National Electrical Carbon Products, 2001(1): 184-191.
[3] XIA Jin-tong, LU Xue-feng, LI Yan, SHAO Hao-ming. Study on the preparation process and properties of high-resistance carbon brush[J]. Natural Science Journal of Hunan University, 2005, 32(4): 88-93. (in Chinese)
[4] WANG Wen-fang, XU Shao-fan, YING Mei-fang, WANG Cheng-fu, CHU Dao-bao. Research on the copper coating graphite-copper matrix composites[J]. Journal of Hefei University of Technology, 1999, 22(1): 35-38. (in Chinese)
[5] MOUSTAFA, S F, E1-BADRY S A, SANAD A M, KIEBACK B. Friction and wear of copper-graphite composites made with Cu-coated and uncoated graphite powders[J]. Wear, 2002, 253: 699-710.
[6] LEE P K. High-current brush material development, Part I: sintered metal-coated graphite[J]. IEEE Transactions on Components, Hybrids, and Manufacturing Technology, 1980, 3(1): 4-8.
[7] MOUSTAFA S F, El-BADRY S A, SANAD A M. Effect of graphite with and without copper coating on consolidation behaviour and sintering of copper-graphite composite[J]. Powder Metallurgy, 1997, 40(3): 201-206.
[8] PAI B C, ROHATGI P K. Copper coating on graphite particles[J]. Mater Sci Eng, 1975, 21: 161-167.
[9] LU W, DONEPUDI V S, PRAKASH J, LIU J, AMINE K. Electrochemical and thermal behaviour of copper coated type MAG-20 natural graphite[J]. Electrochimica Acta, 2002, 47: 1601-1606.
[10] GAO J, FU L J, ZHANG H P, ZHANG T, WU Y P, WU H Q. Suppression of PC decomposition at the surface of graphite carbon by Cu coating[J]. Electrochem Commun, 2006, 8: 1726-1730.
[11] GUO K, PAN Q, WANG L, FANG S. Nano-scale copper-coated graphite as anode material for lithium-ion batteries[J]. J Appl Electrochem, 2006, 32: 679-685.
[12] YUAN Hai-long, FENG Yi. Electroless plating of carbon nanotube with copper[J]. The Chinese Journal of Nonferrous Metals, 2004, 14(4): 665-669. (in Chinese)
[13] DENG Fu-ming, CHEN Xiao-hua, CHEN Wei-xiang, LI Wen-zhu. Electroless plating Ni-P matrix composite coating reinforced by carbon nanotubes[J]. Trans Nonferrous Met Soc China, 2004, 14(4): 681-685.
[14] HE Xin-kuai, CHEN Bai-zhen, HU Geng-sheng, DENG Ling-feng, ZHOU Ning-bo, TIAN Wen-zeng. Process of electroless plating Cu-Sn-Zn ternary alloy[J]. Trans Nonferrous Met Soc China, 2006, 16: 223-228.
[15] XUAN Tian-peng, ZHANG Lei, HUANG Qin-hua. Crystallization behavior of electroless Co-Ni-B alloy plated in magnetic field in presence of cerium[J]. Trans Nonferrous Met Soc China, 2006, 16: 363-367.
[16] HONBO R, SAWA K, SHIMOYAMA, T, MORITA N. Development of the lead-free brush material for the high-load starter[C]//2005 Proceedings of 51 IEEE Holm on Electrical Contacts, 2005: 298-303.
[17] HU Zhong-liang, CHEN Zhen-hua, XIA Jin-tong. Study on surface film in the wear of electrographite brushes against copper commutators for variable current and humidity[J]. Wear, 2007. (in press, dio: 10.1016/j.wear.2007.01.034)
[18] SAVAGE R H. Graphite lubrication[J]. J Appl Phys, 1948, 19(1): 1-10.
[19] SAVAGE R H, SCHAEFER D L. Vapor lubrication of graphite sliding contacts[J]. J Appl Phys, 1956, 27(2): 36-38.
[20] LANCASTER J K, PRITCHARD J R. On the ‘dusting’ wear regime of graphite sliding against carbon[J]. J Phys D: Appl Phys, 1980, 13: 1551-1564.
[21] LANCASTER J K, PRITCHARD J R. The influence of environment and pressure on the transition to dusting wear of graphite[J]. J Phys D: Appl Phys, 1981, 14: 747-762.
(Edited by HE Xue-feng)
Foundation item: Project (59972009) supported by the National Natural Science Foundation of China
Corresponding author: HU Zhong-liang; Tel: +86-13975873284; E-mail: david10103@sina.com