J. Cent. South Univ. Technol. (2008) 15: 295-300
DOI: 10.1007/s11771-008-0055-2

Preparation and properties of PEO/LiClO4/KH560-SiO2 composite polymerelectrolyte by sol-gel composite-in-situ method
PAN Chun-yue(潘春跃), GAO Jin-huan(高金环), ZHANG Qian(张 倩),
FENG Qing(冯 庆), CHAO meng(巢 猛)
(School of Chemistry and Chemical Engineering, Central South University, Changsha 410083)
Abstract: Composite polymer electrolytes based on polyethylene oxide(PEO) were prepared by using LiClO4 as doping salt and silane-modified SiO2 as filler. SiO2 was formed in-situ in (PEO)8LiClO4 matrix by the hydrolysis and condensation reaction of Si(OC4H9)4. The crystallinity, morphology and ionic conductivity of composite polymer electrolyte films were examined by differential scanning calorimetry, scanning electron microscopy, atom force microscopy and alternating current impedance spectroscopy, respectively. Compared with the crystallinity of the unmodified SiO2 as inert filler, that of composite polymer electrolytes is decreased. The results show that silane-modified SiO2 particles are uniformly dispersed in (PEO)8LiClO4 composite polymer electrolyte film and the addition of silane-modified SiO2 increases the ionic conductivity of the (PEO)8LiClO4 more noticeably. When the mass fraction of SiO2 is about 10%, the conductivity of (PEO)8LiClO4-modified SiO2 attains a maximum value of 4.8×10-5 S·cm-1.
Key words: polyethylene oxide(PEO); sol-gel; composite-in-situ; polymer electrolyte; ionic conductivity
1 Introduction
Solid polymer electrolytes based on polyethylene oxide(PEO) have become very important materials for solid lithium–polymer battery technology. The advantages of such polymer electrolytes are: 1) volatile solvent is not incorporated; 2) dendrite formation at the lithium electrode can be minimized. The high crystallinity of PEO at ambient temperature, however, restricts polymer chain movement, which results in a relatively low ionic conductivity at room temperature[1-2]. Now, how to improve the ionic conductivity is still a key problem. A variety of methods may be used, such as modifications of polymer structure[3-4], the incorporation of plasticizers[5-6] or ceramic fillers[7-9]. It is observed that both the ionic conductivity and the mechanical properties can be improved after the addition of ceramic fillers.
Generally, the polymer composites with ceramic fillers are made by mechanical blending, in which the aggregation of nanoparticles is an inevitable event due to the high surface energy of small particles. Sol-gel chemistry as a simple and effective method to resolve this problem has been reported recently[10-12]. This method has been widely used in the preparation of organic-inorganic nanocomposite materials[13]. However, very few studies have been published using this method for the preparation of composite polymer electrolytes[14].
The concept of preparing in-situ formed polymer- ceramic composites was further expanded in this study. Because of the property failure of composite solid polymer electrolyte(CSPE) prepared by mechanical blending, a novel preparation method, composite-in-situ method, was introduced into the preparation of CSPE. The method “composite-in-situ” here means that the inorganic particles are produced in-situ by the sol-gel process of precursors in the polymer medium, and it is called as sol-gel composite-in-situ method. Sol-gel transformation based on the hydrolysis and condensation of tetraethoxysilane (TEOS, Si(OC4H9)4) was used to prepare the inorganic phase. Silane-coupling agent KH560 was used to modify SiO2 particles in order to improve the dispersion of inert SiO2 particles in the polymer matrix. The modification by the silane molecules that were attached to the SiO2 surface could make the polar SiO2 particle surface become a much less polar-sililated surface[15]. The principal motivation of this work was to investigate the effect of interaction between the polymer and fillers by modification of surfaces of fillers. The thermal property, morphology, crystallinity and ionic conductivity of the composite polymer electrolyte films were examined by differential scanning calorimetry (DSC), scanning electron microscopy(SEM), atom force microscopy(AFM), X-ray diffraction(XRD) and alternating current(AC) impedance spectroscopy, respectively.
2 Experimental
2.1 Preparation of polymer electrolyte
Polyethylene oxide (relative molecular mass of 600 000) was obtained from Aldrich Chemicals and dried under vacuum at 50 ℃ for 24 h before use. Lithium perchlorate (LiClO4) was analytical grade and dried under vacuum at 120 ℃ for 24 h to remove crystal- water. Acetonitrile (99.5%) was used as the solvent in the film-casting process, TEOS was analytical grade and used without further purification, and silane-coupling agent KH560 was used as plasticizer.
The required amounts of PEO(Aldrich) and LiClO4 were dissolved in acetonitrile and the mixture was stirred at room temperature for 3 h until a homogeneous solution was obtained, and followed by the addition of the mixed solution composed of TEOS and ethanol with a mass ratio of 1?10. pH value of the solution was adjusted to 4 with hydrochloride. Then the solution was stirred at 55 ℃ for about 8 h and continued to be stirred at room temperature for another 12 h until a homogeneous mixture was obtained. Finally, PEO/LiClO4/SiO2 composite polymer electrolyte was formed. In the above process, KH560 was added to the system before heating (the mass ratio of KH560 and TEOS was 15?1), then the PEO/LiClO4/KH560-SiO2 composite polymer electrolyte was obtained. The molar ratio of EO to Li was fixed at 8?1 for all the samples, and the mass fraction of SiO2 in the sample for testing was fixed to be 10%.
The mixture was achieved by casting the solution on the Teflon dish in air at room temperature to allow the evaporation of acetonitrile. After evaporation of acetonitrile, the membranes were dried in a temperature controlled oven at 50 ℃ for 48 h to remove traces of residual solvent, and the composite polymer electrolyte films were formed.
2.2 Characterization of polymer electrolytes
DSC was performed with a Q-10 analyzer (TA, USA). About 10 mg of sample was sealed in standard aluminum cans and cooled in a nitrogen atmosphere from room temperature to -60 ℃. The heating curve was recorded when the sample was heated from -60 to 100 ℃ at a rate of 10 ℃/min. The morphologies of the composite polymer electrolyte films were examined by a KYKY-2800 scanning electron microscope at 20 kV. The samples were shadowed with Au before SEM measurements. The surface morphology, roughness and fractality of the films were also examined with a Dimension-3000 atom force microscope(AFM) (DI, USA). AC impedance spectra of the composite polymer electrolyte films were measured by using CHI660 electrochemical workstation (Chenhua, Shanghai) between 1 Hz and 100 kHz with 30 mV amplitude. The films were sandwiched between two stainless steel electrodes and assembled into a tightly sealed test cell.
3 Results and discussion
3.1 Morphologies of composite polymer electrolytes
SEM images of PEO/LiClO4, PEO/LiClO4/SiO2 and PEO/LiClO4/KH560-SiO2 polymer electrolyte films are shown in Fig.1.
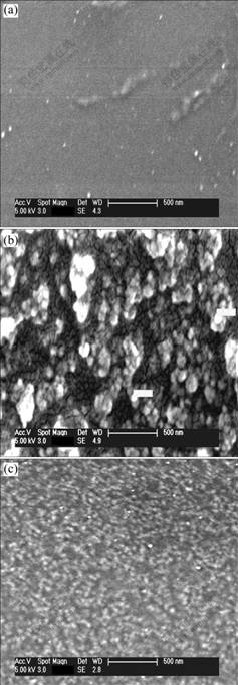
Fig.1 SEM images of PEO/LiClO4(a), PEO/LiClO4/SiO2(b) and PEO/LiClO4/KH560-SiO2(c)
The surface of PEO/LiClO4 is smooth and there are no holes detected in Fig.1(a). From the image shown in Fig.1(b), the nanoparticles cannot disperse well within the remains of the polymer matrix, and show extensive particle agglomeration even after thermal treatment. The average diameter of SiO2 particle agglomeration is about 65 nm. While in Fig.1(c), there is little particle agglo- meration, and small particles of about 30-35 nm in diameter and a narrow size distribution are found. The present preparation method is able to produce and maintain the inorganic phase with high dispersion in the polymer matrix. These dispersed SiO2 particles play an active role in the growth of microstructures, resulting in completely different morphologies. SiO2-polymer interaction may provide additional mechanism of ionic conductivity enhancement along the particles-polymer interface.
To further investigate the morphological characteristics of the PEO/LiClO4/KH560-SiO2 composite polymer electrolyte films, AFM was also used to characterize the surface morphologies of solid polymer electrolytes.
As seen from Fig.2, many SiO2 nano-particles homogeneously disperse on the surface of PEO/LiClO4/ KH560-SiO2 polymer electrolyte. The maximum perpendicular deviation is about 2 nm in Fig.2(b), and the film is plat. The interface of the SiO2 particles and the
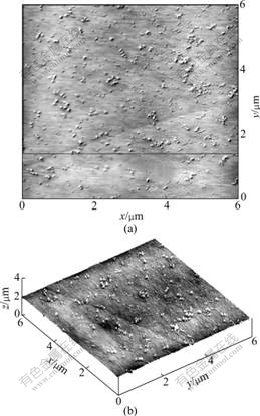
Fig.2 Top view (a) and three-dimensional surface plot (b) of AFM images of PEO/LiClO4/KH560-SiO2 polymer electrolytes
polymer matrix is very illegible, that is to say, the surface modification of these particles can improve the compatibility between the ceramic particles and the polymer matrix.
SiO2 modified by silane-coupling agent KH560 can uniformly disperse in the polymer matrix and has a smaller average diameter. The —OH groups on the SiO2 surface are active in the process of silanization reaction, therefore, the surface —OH groups of SiO2 are replaced by silyloxy, which cannot lead to the augment of the particles. On the other hand, the nanoparticles disperse well within the polymer matrix, just because of steric repulsive actions among the surfaces of the modified nanoparticles. The ether oxygen bonding exists in the surface of modified nanoparticles and in PEO, therefore there is a good compatibility between the nanoparticles and PEO.
3.2 Thermal analysis of polymer electrolyte
The DSC results of the PEO-LiClO4, PEO/LiClO4/ SiO2 and PEO/LiClO4/KH560-SiO2 composite polymer electrolyte films are shown in Fig.3 and Table 1.
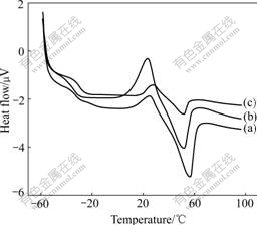
Fig.3 DSC curves for PEO/LiClO4(a), PEO/LiClO4/SiO2(b) and PEO/LiClO4/KH560-SiO2(c)
Table 1 Results of DSC curves for three samples
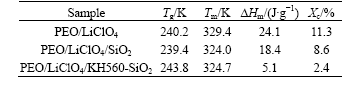
The three DSC curves in Fig.3 show obvious transition in low temperature region assigned to the glass transition temperature(Tg) of composite polymer electrolyte films. All the samples exhibit a relatively sharp endothermic peak at about 50 ℃, which can be attributed to the melting of the crystalline region of PEO. The crystallinity(Хc) of composite polymer electrolyte films can be obtained by comparing the heat of melting (?Hm) with that (213.7 J/g) of 100% crystalline PEO[16]. The important data obtained from the first heating curves are summarized in Table 1.
It can be known from Table 1 that the degree of crystallinity(Хc) of PEO in PEO/LiClO4/SiO2 is smaller than that in PEO/LiClO4, and the degree of crystallinity of PEO is reduced greatly to be only 2.4% in PEO/ LiClO4/KH560-SiO2. This observation can be explained in terms of hydrogen bonding between the surface —OH groups of SiO2 particles and the ether oxygen atoms in the polymer chains, which weakens the complexion between Li+ and the ether oxygen atoms, facilitates the migration of Li+ and perturbs the PEO chain conformation, and therefore introducing additional free space among the polymer segments and yielding a lower Хc. Meanwhile, the modified SiO2 by KH560 can uniformly disperse in the polymer matrix and has a smaller diameter, which indicates that the polymer segmental motion is increased and the degree of crystallinity of PEO is decreased. These results are beneficial to ionic conduction. Furthermore, Хc of composite polymer electrolyte films mostly decreases when SiO2 and KH560-modified SiO2 are added, which leads to the decrease of Tm. The decrease in Tm upon SiO2 addition is attributed to the appearance of smaller crystallites due to the presence of ceramic particles. Because ionic conduction takes place primarily through the amorphous phase of the polymer, high crystallinity will lead to low conductivity.
3.3 XRD analysis of polymer electrolyte
The XRD patterns of PEO/LiClO4, PEO/LiClO4/ SiO2 and PEO/LiClO4/KH560-SiO2 polymer electrolyte are shown in Fig.4.
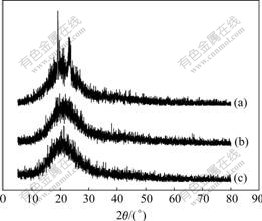
Fig.4 XRD patterns for PEO/LiClO4(a), PEO/LiClO4/SiO2(b) and PEO/LiClO4/KH560-SiO2(c)
PEO/LiClO4 sample shows much higher diffraction peaks (Fig.4(a)) than the samples of PEO/LiClO4/SiO2 (Fig.4(b)) and PEO/LiClO4/KH560-SiO2 (Fig.4(c)). The XRD patterns show that the crystallinity of PEO can be inhibited by adding inorganic particles. It can be seen that the crystallinity of PEO is in good agreement with the result of DSC.
3.4 Ionic conductivity
The conductivities of PEO/LiClO4/SiO2 and PEO/ LiClO4/KH560-SiO2 composite polymer electrolyte are shown in Fig.5.
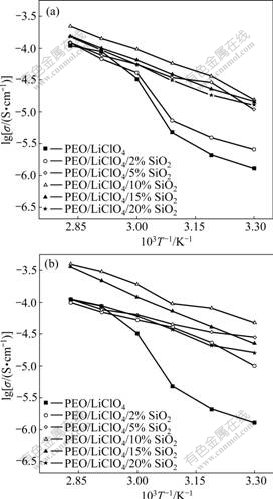
Fig.5 Temperature dependence of ionic conductivity on PEO/ LiClO4/SiO2(a) and PEO/LiClO4/KH560-SiO2(b)
Fig.5 shows temperature dependence on the ionic conductivity for the (PEO)/LiClO4/SiO2 composite electrolytes. The plot of lg σ vs 1 000/T is approximately linear, suggesting that the Arrhenius equation can be a good fit for the experimental data:
σ=σ0exp[-Ea/(kT)]
where σ is the conductivity, σ0 is the conductivity pre-exponential factor and Ea is the activation energy for conduction. It indicates that the interface between SiO2 and polymer/salt electrolyte is very important for ionic conduction. It is suggested that the weakening of the polyether-cation association induced by the ceramic particles might be important for ionic conduction. The conductivity increases with the increase in SiO2 content and then attains a maximum value when the mass fraction of SiO2 is about 10% for both SiO2 and KH560-modified SiO2 composite electrolytes. Subsequently, the conductivity decreases with further increase of SiO2 content. The enhancement in ionic conductivity due to the addition of ceramic fillers has been explained by an improved effective-medium theory (EMT)[17]. From a microscopic perspective, the composite electrolyte can be treated as a quasi two-phase system that consists of a polymeric ion-conducting matrix with dispersed composite units. The ionic conductivity could arise from the existence of a highly conducting layer at the electrolyte/filler interface[18]. This interface layer could be an amorphous polymer layer surrounding SiO2 or a space-charge layer[19]. At low ceramic filler loadings, the conductivity increases with SiO2 content basically, due to the increase in amount of the conductive layers. The conductivity does not continue to rise indefinitely with increasing concentration of SiO2 particles. This behavior is a direct consequence of the high concentration of SiO2, which tends to impede ionic movement by acting as mere insulators.
For further illustration, Fig.6 shows the ionic conductivities of PEO/LiClO4 with various mass fractions of SiO2 and KH560-modified SiO2 at 30 ℃. Fig.6 shows that the KH560-modified SiO2 composite electrolyte can produce greater enhancement in ionic conductivity than unmodified SiO2, especially at low loading levels. Thus the surface modification of SiO2 is an effective approach to prepare composite electrolytes with enhanced performance. The modified SiO2 particles are more compatible with the PEO/LiClO4 solution, because the silane molecule carries a short PEO block. As discussed above, the interaction between active silane and SiO2 leads to a monolayer coverage of the short polyether units on the SiO2 surface[15], and the oxygen atoms from the short polyether units on the SiO2 surface can compete with oxygen atoms in the PEO backbone for complexation with Li+ ions, resulting in a more relaxed coordination between oxygen atoms and Li+ ions and thereby facilitating the transport of Li+ ions through the polymer. On the other hand, the silane moieties attached on the SiO2 particle surface can effectively improve the dispersion of the SiO2 particles in the PEO matrix during blending because of steric repulsive actions. In comparison with the case of unmodified SiO2 powder, a larger electric interfacial area between the polymer and fillers can be reached. The increase of the polymer-SiO2 interfaces raises the proportion of effective media for ion conduction in the electrolyte, which leads to enhancement in ionic conductivity.
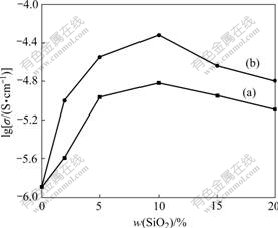
Fig.6 Conductivity of samples PEO/LiClO4/SiO2 (a) and PEO/ LiClO4/KH560-SiO2 (b) with various mass fractions of SiO2 at 30 ℃
3.5 Electrochemistry stability window
An important characteristic of polymer electrolyte for its technological application is the electrochemical stability window, which can be measured by cyclic voltammetry. Fig.7 shows the cyclic voltammograms for polymer electrolytes based on PEO/LiClO4/KH560-SiO2. It can be seen that there are two oxidation and reduction peaks at the potential of 4.50 V and 3.62 V in the cyclic voltammetry profiles, indicating that there exist two processes during both intercalation and deintercalation of Li+. With the increase of circle time, the current of oxidation and reduction peaks decrease gradually and the shape of reduction peak becomes wider, which shows that it is difficult to remove and embed for Li+ from the anode. Both cases exhibit a good electrochemical stability window of 3.70 V (vs Li+/Li) for composite complexes with KH560-modified SiO2. The stability window might be related to the excellent dispersion of SiO2 particles when they are modified with KH560 silane-coupling agent.
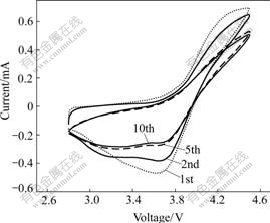
Fig.7 10 cyclic voltammograms of LiCoO2/PEO/LiClO4/ KH560-SiO2/Li
4 Conclusions
1) PEO/LiClO4/KH560-SiO2 composite polymer electrolytes are prepared by using LiClO4 as doping salt and silane-modified SiO2 as filler. DSC shows that crystallinity of PEO is 8.6% and 2.4% respectively for PEO/LiClO4/SiO2 and PEO/LiClO4/KH560-SiO2 composite polymer electrolytes. Therefore, the addition of modified SiO2 by silane-coupling agent KH560 decreases more crystallinity of composite polymer electrolyte film than unmodified SiO2 and leads to a higher ionic conductivity. XRD result also shows that the crystallinity of PEO can be inhibited by adding inorganic particles.
2) The SEM and AFM show that modified SiO2 particles are uniformly mixed at the nanometer level. SiO2 particles are about 30-35 nm in diameter, which can result in more interfacial regions between particles and polymer.
3) Modified SiO2 in PEO-based polymer electrolytes effectively leads to greater enhancement in ionic conductivity. When the mass fraction of SiO2 is about 10%, the conductivity of (PEO)8LiClO4-modified SiO2 attains a maximum value of 4.8×10-5 S/cm at 30 ℃. The enhancement can be attributed to the good dispersion of silane-modified SiO2 in polymer electrolyte.
References
[1] TOMINAG Y, ASAI S, SUMITA M, PANERO S, SCROSATI B. A novel composite polymer electrolyte: Effect of mesoporous SiO2 on ionic conduction in poly(ethylene oxide)-LiCF3SO3 complex [J]. J Power Sources, 2005, 146(1/2): 402-406.
[2] HU Yong-jun, CHEN Bai-zhen, YUAN Yan. Preparation and electrochemical properties of polymer Li-ion battery reinforced by non-woven fabric [J]. Journal of Central South University of Technology, 2007, 14(1): 47-50.
[3] BOURIDAH A, DALARD F, DEROO D, CHERADAME H, LENEST J F. Poly(dimethylsiloxane)-poly(ethylene oxide) based polyurethane networks used as electrolytes in lithium electrochemical solid state batteries [J]. Solid State Ionics, 1985, 15(3): 233-240.
[4] BLONSKYP M, SHRIVER D F, AUSTIN P, ALLCOCK H R. Polyphosphazene solid electrolytes [J]. Am Chem Soc, 1984, 106(22): 6854-6855.
[5] KIM Y T, SMOTKIN E S. The effect of plasticizers on transport and electrochemical properties of PEO-based electrolytes for lithium rechargeable batteries [J]. Solid State Ionics, 2002, 149(1/2): 29-37.
[6] YANG X Q, LEE H S, HANSON L, MCBREEN J, OKAMOTO Y. Development of a new plasticizer for poly(ethylene oxide)-based polymer electrolyte and the investigation of their ion-pair dissociation effect [J]. J Power Sources, 1995, 54(2): 198-204.
[7] CROCE F, APPETECCHI G B, PERSI L, SCROSATI B. Nanocomposite polymer electrolytes for lithium batteries [J]. Nature, 1998, 394(6692): 456-458.
[8] CROCE F, PERSI L, SCROSATI B, SERRAINO-FIORYF, PLICHTA E, HENDRICKSON M A. Role of the ceramic fillers in enhancing the transport properties of composite polymer electrolytes [J]. Electrochimica Acta, 2001, 46(16): 2457-2461.
[9] PAN Chun-yue, FENG Qing, WANG Li-jun, ZHANG Qian, CHAO Meng. Morphology and conductivity of in-situ PEO-LiClO4-TiO2 composite polymer electrolyte [J]. Journal of Central South University of Technology, 2007, 14(3): 348-352.
[10] KWEON J O, NOH S T. Thermal, thermo, mechanical, and electrochemical characterization of the organic-inorganic hybrids poly(ethylene oxide) (PEO)-silica and PEO-silica-LiClO4 [J]. Appl Polym Sci, 2001, 81(10): 2471-2479.
[11] MELLO N C, BONAGAMB T J, PANEPUCCI H, DAHMOUCHE K, JUDEINSTEIN P, AEGERTER M A. NMR study of ion conducting organic-inorganic nanocomposites poly(ethylene glycol) silica LiClO4 [J]. Macromolecules, 2000, 33(4): 1280-1288.
[12] de SOUZA P H, BIANCHI R F, DAHMOUCHE K, JUDEINSTEIN P, FARIA R M, BONAGAMBA T J. Solid-state NMR, ionic conductivity and thermal studies of lithium doped siloxane-poly (propylene glycol) organic-inorganic nanocomposites [J]. Chem Mater, 2001, 13(10): 3685-3692.
[13] FAN L Z, NAN C W, ZHAO S J. Effect of modified SiO2 on the properties of PEO-based polymer electrolytes [J]. Solid State Ionics, 2003, 164(1/2): 81-86.
[14] LIU Y, LEE J Y, HONG L. In situ preparation of poly(ethylene oxide)-SiO2 composite polymer electrolytes [J]. Power Sources, 2004, 129(2): 303-311.
[15] LIU Y, LEE J Y, HONG L. Functionalized SiO2 in poly(ethylene oxide)-based polymer electrolytes [J]. J Power Sources, 2002, 109(2): 507-514.
[16] DAI Y, GREENBAUM S, GOLODNITSKY D, ARDEL G, STRAUSS E, PELED E, ROSENBERG Y. Lithium-7NMR studies of concentrated LiI/PEO-based solid electrolytes [J]. Solid State Ionics, 1998, 106(1/2): 25-32.
[17] WIECZOREK W, ZALEWSKA A, SIEKIERSKI M, PRZYLUSKI J. Modelling the ac conductivity behaviour of composite polymeric electrolytes by the effective medium theory [J]. Solid State Ionics, 1996, 86/88(1): 357-362.
[18] NAN C W, SMITH D M. A.C. electrical properties of composite solid electrolytes [J]. Mater Sci Eng A, 1991, A10(2): 99-106.
[19] SATA N, EBERMAN K, EBERL K, MAIER J. Mesoscopic fastion conduction in nanometre-scale planar [J]. Nature, 2000, 408(6815): 946-949.
(Edited by CHEN Wei-ping)
Received date: 2007-09-08; Accepted date: 2007-10-25
Corresponding author: PAN Chun-yue, Professor; Tel: +86-731-8836961; E-mail: panchunyue@sina.com