Trans. Nonferrous Met. Soc. China 23(2013) 2307-2311
Preparation of LaMgNi4-xCox alloys and hydrogen storage properties
Jian-bo TAN1, Xiao-qin ZENG1,2, Jian-xin ZOU1, Xiao-mei WU1, Wen-jiang DING1
1. Shanghai Engineering Research Center of Magnesium Materials and Applications,
School of Materials Science and Engineering, Shanghai Jiao Tong University, Shanghai 200240, China;
2. State Key Laboratory of Metal Matrix Composites, School of Materials Science and Engineering, Shanghai Jiao Tong University, Shanghai 200240, China
Received 2 July 2012; accepted 28 September 2012
Abstract: The LaMgNi4-xCox (x=0, 0.3, 0.5) compounds were prepared by the method of levitation melting and a subsequent heat treatment at 1073 K for 10 h. XRD analysis shows that the obtained LaMgNi4-xCox alloys consist of a single phase with the structure of cubic SnMgCu4 (AuBe5 type). The hydrogen absorption/desorption properties of LaMgNi4 were investigated by PCI measurement at various temperatures (T=373, 398, 423 K) and the results show that the maximum absorbed hydrogen capacity reaches 1.45% (5.79H/M) under a hydrogen pressure of 4.3 MPa at 373 K. The XRD patterns during absorbing procedure at 373 K indicate the phase structure changing from cubic (α-LaMgNi4) to orthorhombic (β-LaMgNi4H3.41) and after hydrogenation finally back to cubic (γ-LaMgNi4H4.87), and a partial desorption was also observed under this condition. With increasing temperature, a slight decrease of the absorbed hydrogen content was observed and the number of plateaus reduces from two to one, but the hydrogen absorption kinetics improves. The electrochemical properties of the LaMgNi4-xCox were measured by simulated battery test, which shows that the discharge capacity of the alloys significantly improves with the increase of Co content.
Key words: hydrogen storage alloy; electrochemical properties; LaMgNi4-xCox
1 Introduction
Hydrogen storage alloy has aroused great interest in view of its potential as a new energy storage material. During the last years, magnesium based alloys showed large potential for hydrogen storage applications due to their high hydrogen capacity and low costs [1,2], but because of the high temperature of dehydrogenation and poor kinetics, the applications of these alloys are still limited [3,4]. Recently, a lot of investigations have been done on the RE-Mg-Ni (RE=rare earth) system [5-8], and it has been shown that the ternary compounds RE1-xMgxNi2 based on Laves structure are new promising in hydrogen-absorbing capacity and electrochemical properties [9-12].
In previous works, BOBET et al [13] reported that LaMgNi4 compound absorbs 3.8H/M at room temperature but no desorption has been observed even at temperature up to 473 K under primary vacuum. Nevertheless CHOTARD et al [14] reported that LaMgNi4H4.85 formed at a pressure of more than 2 MPa and a subsequent decrease of hydrogen pressure to ambient conditions led to hydrogen desorption of 1.5H/M hydrogen at 373 K. WANG et al [15] also reported about electrochemical properties of LaMgNi4 that the discharge capacity reaches 400 mA·h/g which is much higher than that of the commercial AB5 alloys (330 mA·h/g).
To the best of our knowledge, there are neither related investigations on the reversible properties of LaMgNi4 hydrogen absorption/desorption at different temperatures, nor complete pressure-composition isotherm (PCI) curves about the compound available. Also a lot of contradictory conclusions were made whether LaMgNi4 can desorb hydrogen or not. For these reasons, the compounds LaMgNi4-xCox (x=0, 0.3, 0.5) were synthesized by levitation melting first and then the thermodynamic and kinetic hydrogen storage properties of LaMgNi4 were investigated systematically at different temperatures. Finally, the effects of Co addition on the electrochemical properties of LaMgNi4-xCox by partial substitution of Ni with Co were studied.
2 Experimental
LaMgNi4-xCox (x=0, 0.3, 0.5) alloys were synthesized by levitation melting and a subsequent heat treatment at 1073 K for 10 h under argon atmosphere and crushed to powders with average diameter of 75 μm. X-ray analysis was employed to identify the phase by a Rigaku D/max-2550VL/PC diffractometer with Cu Kα radiation (λ=0.15405 nm), and the experimental parameters were 150 mA, 35 kV, and 4 (°)/min. The hydrogen absorption/desorption properties were investigated by pressure composition isotherms with the use of an automatic Sievert-type volumetric apparatus at different temperatures (373, 398, 423 K).
The electrode properties were determined by conducting a simulated battery test through the following procedures. Firstly, the alloy powder was mixed with hydroxyl nickel powder in the mass ratio of 1:4 with a total mass of 1.5 g. All the powders with a round nickel foam (diameter 10 mm) were compressed in a tablet press (inner diameter 10 mm) under a pressure of 15 MPa for 3 min. Secondly, a small nickel bar as the negative connecting line was welded on the pellet. After that, the pellet was interposed by a piece of diaphragm paper, and then fixed by Ni(OH)2/NiOOH positive electrode. The negative electrode and counter electrode were placed into 6 mol/L KOH aqueous solution. The test was carried out at 298 K using a computer-controlled battery testing instrument (DC-5). During the activation test, the charge was conducted using the current rate of 60 mA/g for 8 h and discharged at 60 mA/g to the cut-off potential of 1 V. In the charge-discharge recycle test, negative electrodes were charged at 120 mA/g for 4 h, and discharged at 120 mA/g to the cut-off potential of 0.8 V.
3 Results and discussion
3.1 Preparation of LaMgNi4-xCox
The phase identification of LaMgNi4-xCox (x=0, 0.3, 0.5) was carried out by XRD and analyzed by Jade 5.0 software. Figure 1 reveals that only the diffraction peaks of LaMgNi4 were observed, which means the single- component alloys are synthesized. With the addition of Co content, there are almost no changes in the phase structure, which has been reported by KADIR et al [16], the cubic structure of SnMgCu4, where the La atom occupies the position of 4a sites and Mg atom occupies the position of 4c sites. It has been reported that the order/disorder between RE and Mg on both 4a and 4c sites can influence the hydrogen absorption properties [17,18].
The crystallographic data for LaMgNi4-xCox were calculated with Jade 5.0 software (See Table 1). The value of lattice constant for LaMgNi4 is 7.1443
, which is slightly smaller than that reported by KADIR et al [16] (a=7.1794
), and increases to 7.1681
after addition of Co content. The unit cell volume per formula unit was also calculated and increased from 364.7
3 to 368.3
3.
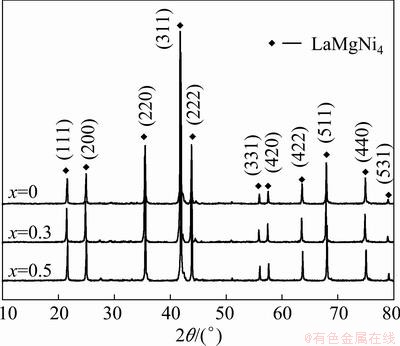
Fig. 1 XRD patterns of LaMgNi4-xCox
Table 1 Crystallographic data for LaMgNi4-xCox
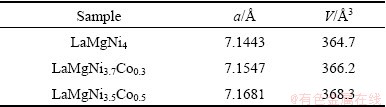
3.2 Hydrogen absorption and desorption properties
The PCI curves of LaMgNi4 at various temperatures (373, 398 and 423 K) are shown in Fig. 2, where the horizontal axis presents the hydrogen content. The maximum hydrogen content is 1.45% (5.79H/M) under a hydrogen pressure of 4.3 MPa at 373 K. It can be clearly seen that there are two well defined plateaus at the temperature of 373 K. With increasing temperature, the high plateau becomes narrow and steep, and finally shifts to be just one long plateau when the temperature reaches 423 K. It is not common to see this phenomenon in other REMgNi4 compounds such as YMgNi4 [18] and NdMgNi4 [19]. In order to analyze this transformation, the XRD analysis was carried out at each plateau at different temperatures. Figure 3 shows the XRD patterns of those hydride samples at two plateaus at 373 K. It is observed that hydride phase at a lower plateau can be explained with the hydrogenation of NdMgNi4 [20], and the transformation from the original cubic to orthorhombic after hydrogenation. From the PCI curves, it is obvious that the first plateau corresponds to the transformation from α phase (LaMgNi4) to β-LaMgNi4H3.41, and the second plateau is related to the transformation of β-LaMgNi4H3.41 to γ-LaMgNi4H4.87, which has been reported by CHOTARD et al [14] that it has got the cubic symmetry again. Figure 4 shows the XRD patterns of plateaus at 398 K and 423 K. It is obviously to see that the phase structure at 398 K has the same transformation at the temperature of 373 K, and the plateau at 423 K corresponds to β-LaMgNi4H3.20.
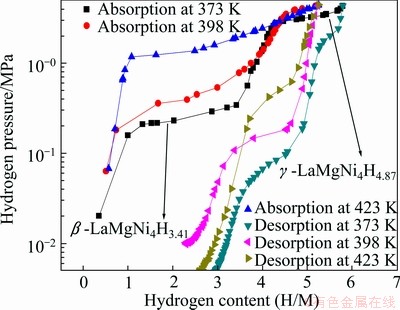
Fig. 2 Pressure—composition isotherm curves of LaMgNi4at 373, 398 and 423 K
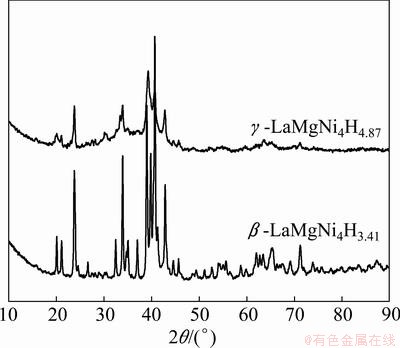
Fig. 3 XRD patterns of two plateaus at temperature of 373 K
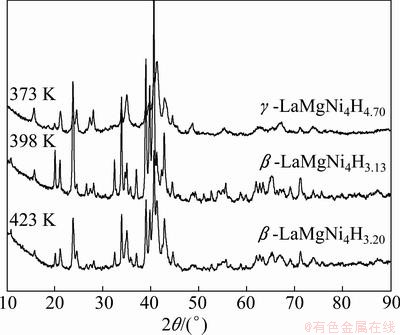
Fig. 4 XRD patterns of plateaus at temperatures of 373, 398 and 423 K
Table 2 presents the PCI characteristics of LaMgNi4 at different temperatures. The hydrogen absorption contents are 5.79H/M (1.45%), 5.24H/M (1.30%), and 5.25H/M (1.31%) at 373, 398, and 423 K, respectively. The hydrogen desorption contents are 2.90H/M (0.73%), 2.90H/M (0.73%), and 2.70H/M (0.68%), respectively. The absorption hydrogen plateau pressures are approximately 0.23 MPa and 2.83 MPa at 373 K, 0.38 MPa at 398 K and 1.26 MPa at 423 K. The desorption hydrogen plateaus of LaMgNi4 are not so well defined, so they are not listed here. However, the hydrogen can be partially desorbed at all three temperatures (see Fig. 3). This is slightly different from BoBet’s work [13], who reported that no desorption has been observed even at temperature up to 473 K under primary vacuum.
Table 2 PCI characteristics of LaMgNi4 at different temperatures (373, 398 and 423 K)
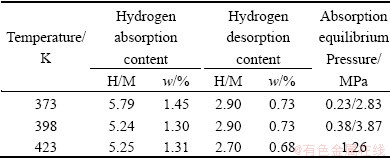
In order to analyze the hydrogen absorption kinetics of LaMgNi4, the samples were placed into hydrogen gas under a pressure of 5 MPa at various temperatures (373, 398 and 423 K). The data are recorded every second for a total time of 2 h. Figure 5 presents the hydrogen absorption kinetics of LaMgNi4, from which it can be seen that the hydrogen absorption rate becomes higher with increasing temperature. The hydrogen content reaches its stable values of 1.14% and 1.21% after about 1.62 ks at 398 K and 423 K, respectively, but it takes much longer (about 3.6 ks) to reach the stable value of 1.25% at 373 K. From Fig. 5, it is obviously to see that the plateau at 373 K is higher than that at 398 K, which might be due to the fact that the hydrogen absorbing driving force depends on the pressure and temperature, at low temperature like 373 K, the equilibrium pressure is much lower which allows it easier to absorb more hydrogen. Figure 2 obviously shows that the maximum hydrogen absorption is higher than the stable value at each temperature, which indicates the relatively slow hydrogen absorption kinetics.
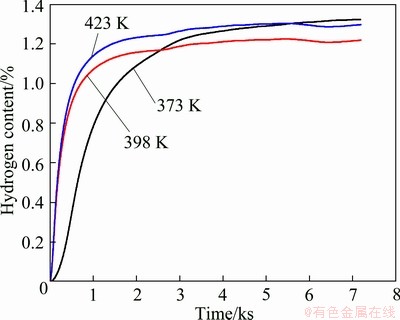
Fig. 5 Absorption kinetics of LaMgNi4 under 5 MPa hydrogen pressure at different temperatures
3.3 Electrochemical properties
The activation capabilities of the LaMgNi4-xCox alloys measured at a charging/discharging current density of 60 mA/g are shown in Fig. 6. The alloys exhibit good activation capabilities, and can be completely activated only after 4 cycles. The discharge capacities increase from 290.2 mA·h/g (x=0) to 328.5 mA·h/g (x=0.5) with the variety of Co content.
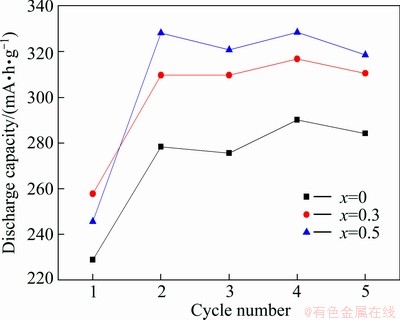
Fig. 6 Evolution of activation capabilities of LaMgNi4-xCox alloys (x=0, 0.3 0.5)
The cycle stabilities of LaMgNi4-xCox alloys measured at a charging/discharging current density of 120 mA/g are shown in Fig. 7. It can be seen clearly that when x rises from 0 to 0.5, the maximum discharge capacity of the alloys increases from 252.6 mA·h/g to 288.4 mA·h/g. This discharge capacity is lower than that reported by WANG et al [15], who measured the maximum capacity of 400 mA·h/g for LaMgNi4. The difference can be ascribed to the difference in the preparation method (melting here and ball milling for Wang), and the different charging/discharging conditions. The cycle stability is characterized by the discharge conservation rate defined as k=(C100/Cmax)×100%, where Cmax is the maximum discharge capacity and C100 is the discharge capacity at the 100th charging/discharging cycle. The value of k, C100 and Cmax of LaMgNi4-xCox alloys are listed in Table 3. It can be concluded that the addition of Co content can improve the discharge capacity of the alloys, which may be due to the increase of unit cell volumes caused by the addition of Co, which can let more hydrogen come into the unit cell. The k is not obviously changed, indicating that the addition of Co has no contribution to the improvement of cycle stabilities.
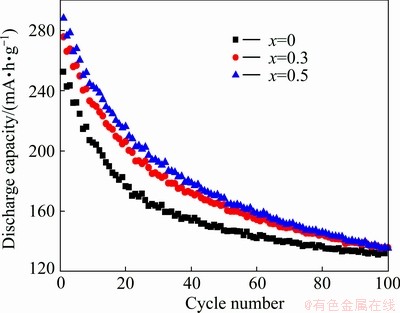
Fig. 7 Evolution of discharge capacities of LaMgNi4-xCox alloys (x=0, 0.3 0.5)
Table 3 Values of k, C100 and Cmax
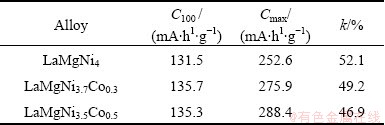
4 Conclusions
1) The single-phase LaMgNi4-xCox (x=0, 0.3 0.5) alloys are synthesized by the method of levitation melting and a subsequent heat treatment at 1073 K for 10 h under argon atmosphere. The unit cell volume per formula unit increases with Co addition.
2) The maximum hydrogen absorption content is 1.45% (5.79H/M) under a hydrogen pressure of 4.3 MPa at 373 K. Hydrogenation leads to a phase structure transformation from original cubic SnMgCu4 type to orthorhombic and finally back to the cubic structure. With increasing temperature, the hydrogen absorption content decreases and the hydride phase reduces from two to one, but the hydrogen absorption kinetics improves.
3) The discharge capacities of the alloys improve from 252.6 mA·h/g (x=0) to 288.6 mA·h/g (x=0.5) with the addition of Co content, but it has no contribution to the improvement of cycle stabilities.
References
[1] ORIMO S, FUJII H. Materials science of Mg-Ni-based new hydrides [J]. Applied Physics A, 2001, 72(2): 167-186.
[2] ORIMO S, FUJII H. Effects of nanometer-scale structure on hydriding properties of Mg-Ni alloys: A review [J]. Intermetallics, 1998, 6(3): 185-192.
[3] ZALUSKA A, ZALUSKI L, STROM-OLSEN J O. Nanocrystalline magnesium for hydrogen storage [J]. J Alloys Compd, 1999, 288(1-2): 217-225.
[4] BARKHORDARIAN G, KLASSEN T, BORMANN R. Effect of Nb2O5 content on hydrogen reaction kinetics of Mg [J]. J Alloys Compd, 2004, 364(1-2): 242-246.
[5] OZAKI T, KANEMOTO M, KAKEYA T, KITANO Y, KUZUHARA M, WATADA M, TANASE S. Stacking structures and electrode performances of rare earth–Mg–Ni-based alloys for advanced nickel–metal hydride battery [J]. J Alloys Compd, 2007, 446-447: 620-624.
[6] REN Hui-ping, ZHANG Yang-huan, LI Bao-wei, ZHAO Dong-liang, GUO Shi-hai, WANG Xin-lin. Influence of the substitution of La for Mg on the microstructure and hydrogen storage characteristics of Mg20-xLaxNi10 (x=0-6) alloys [J]. Int J Hydrogen Energy, 2009, 34(3): 1429-1436.
[7] DONG Xiao-ping, LU Fan-xiu, ZHANG Yang-huan, YANG Li-ying, WANG Xin-lin. Effect of La/Mg on the structure and electrochemical performance of La–Mg–Ni system hydrogen storage electrode alloy [J]. Mater Chem Phys, 2008, 108(2-3): 251-256.
[8] ZHANG Yang-huan, ZHAO Dong-liang, SHI Yan-chun, QI Yan, GUO Shi-hai, WANG Xin-lin. Structures and electrochemical performances of La0.75-xZrxMg0.25Ni3.2Co0.2Al0.1 (x=0-0.2) electrode alloys prepared by melt spinning [J]. Transactions of Nonferrous Metals Society of China, 2010, 20(3): 405-411.
[9] PRIGENT J, GUPTA M. Ab initio study of the hydrogenation properties of Mg-based binary and ternary compounds Mg2X (X=Ni, Si) and YMgNi4 [J]. J Alloys Compd, 2007, 446-447: 90-95.
[10] WANG Z M, ZHOU H Y, CHENG G, GU Z F, YU A B. Preparation and electrode properties of new ternary alloys: REMgNi4 (RE=La, Ce, Pr, Nd) [J]. J Alloys Compd, 2004, 384(1-2): 279-282.
[11] STAN C, ANDRONESCU E, PREDOI D, BOBET J L. Structural and hydrogen absorption/desorption properties of YNi4-xAlxMg compounds (with 0≤x≤1.5) [J]. J Alloys Compd, 2008, 461(1-2): 228-234.
[12] HANADA N, ORIMO S, FUJII H. Hydriding properties of ordered/disordered-Mg-based ternary Laves phase structures [J]. J Alloys Compd, 2003, 356-357: 429-432.
[13] BOBET J L, LESPORTES P, ROQUEFERE G L, CHEVALIER B, ASANO K, SAKAKI K, AKIBA E. A preliminary study of some "pseudo-AB(2)" compounds: RENi4Mg with RE=La, Ce and Gd. Structural and hydrogen sorption properties [J]. Int J Hydrogen Energy, 2007, 32(13): 2422-2428.
[14] CHOTARD J L, SHEPTYAKOV D, YVON K. Hydrogen induced site depopulation in the LaMgNi4-hydrogen system [J]. Z Kristallogr, 2008, 233(10): 690-696.
[15] WANG Z M, ZHOU H Y, GU Z F, CHENG G, YU A B. Preparation of LaMgNi4 alloy and its electrode properties [J]. J Alloys Compd, 2004, 377(1-2): L7-L9.
[16] KADIR K, NOREUS D, YAMASHITA I. Structural determination of AMgNi4 (where A=Ca, La, Ce, Pr, Nd and Y) in the AuBe5 type structure [J]. J Alloys Compd, 2002, 345(1-2): 140-143.
[17] KITANO Y, YAMADA K, MIYAMOTO M, ORIMO S, FUJII H, AONO K, TANABE E. Electron microscopy and hydriding properties of MgYNi4 synthesized by mechanical alloying [J]. J Alloys Compd, 2002, 330-332: 292-295.
[18] AONO K, ORIMO S, FUJII H. Structural and hydriding properties of MgYNi4: A new intermetallic compound with C15b-type Laves phase structure [J]. J Alloys Compd, 2000, 309(1-2): L1-L4.
[19] WANG Z M, ZHOU H Y, ZOU R P, YAO Q R. Effect of sintering conditions on the formation of single-phase NdMgNi4 compound and its hydrogen storage properties [J]. J Alloys Compd, 2007, 429(1-2): 260-263.
[20] GUENEE L, FAVRE-NICOLIN V, YVON K. Synthesis, crystal structure and hydrogenation properties of the ternary compounds LaNi4Mg and NdNi4Mg [J]. J Alloys Compd, 2003, 348(1-2): 129-137.
LaMgNi4-xCox合金的制备及储氢性能
谭剑波1,曾小勤1,2,邹建新1,吴晓梅1,丁文江1
1. 上海交通大学 材料科学与工程学院,上海市镁材料及应用工程技术研究中心,上海 200240;
2. 上海交通大学 材料科学与工程学院,金属基复合材料国家重点实验室,上海 200240
摘 要:采用悬浮熔炼加烧结的方法制备LaMgNi4-xCox(x=0, 0.3, 0.5)化合物。XRD的测试结果表明,所制得的LaMgNi4-xCox (x=0, 0.3, 0.5)均为单相,其结构为SnMgCu4 (AuBe5 型)。利用PCI 对LaMgNi4在不同温度下的吸放氢性能进行测试,结果表明在373 K、4.3 MPa氢气的条件下吸氢量达到最大(1.45%),在吸氢过程中发生了由立方结构的α-LaMgNi4到正交结构的β-LaMgNi4H3.41再到立方结构的γ-LaMgNi4H4.87的变化,而放氢过程中只能观测到部分氢气放出。随着温度的升高,合金的吸氢量有所降低,同时吸氢平台的数量由2个变为1个,但吸氢动力学性能得到提高。采用模拟电池实验测试了LaMgNi4-xCox (x=0, 0.3, 0.5)的电化学性能,结果表明合金的最大放电容量随着Co含量的增加而增大。
关键词:储氢合金;电化学性能;LaMgNi4-xCox
(Edited by Xiang-qun LI)
Foundation item: Projects (10JC407700, 11ZR1417600) supported by the Science and Technology Committee of Shanghai, China; Project (12ZZ017) supported by the Shanghai Education Commission, China
Corresponding author: Xiao-qin ZENG; Tel: +86-21-54742301; Fax: +86-21-34203730; E-mail: xqzeng@sjtu.edu.cn
DOI: 10.1016/S1003-6326(13)62733-8