
Development of aluminum rheo-extrusion process using semi-solid slurry at low solid fraction
T. RATTANOCHAIKUL1, S. JANUDOM1, N. MEMONGKOL2, J. WANNASIN1
1. Department of Mining and Materials Engineering, Faculty of Engineering, Prince of Songkla University,
Hat Yai, Songkhla, 90112, Thailand;
2. Department of Industrial Engineering, Faculty of Engineering, Prince of Songkla University,
Hat Yai, Songkhla, 90112, Thailand
Received 13 May 2010; accepted 20 June 2010
Abstract: An aluminum extrusion process is mainly used to fabricate long tubes, beams and rods for various applications. However, this process has a high production cost due to the need for investment of high-pressure machinery. The objective of this work is to develop a new semi-solid extrusion process using semi-solid slurry at low solid fractions. A laboratory extrusion system was used to fabricate aluminum rods with the diameter of 12 mm. The semi-solid metal process used in this study was the gas induced semi-solid (GISS) technique. To study the feasibility of the GISS extrusion process, the effects of extrusion parameters such as plunger speed and solid fraction on the extrudability, microstructure, and mechanical properties of extruded samples were investigated. The results show that the plunger speed and solid fraction of the semi-solid metal need to be carefully controlled to produce complete extruded parts.
Key words: aluminum alloys; extrusion; semi-solid metal; rheo-extrusion; gas induced semi-solid (GISS)
1 Introduction
Extrusion is one of the various forming processes that is used to produce long and straight metal products with constant cross section, such as bars, solid and hollow sections, tubes, and wires[1]. In extrusion process, a billet is heated and forced through a die orifice. The products from this extrusion process are in a near net shape. However, the extrusion process requires a high-pressure machine to force the metal in the solid state. In addition, defects such as surface cracking, oxide inclusion and piping defect can be found in the products of an extrusion process[2].
An alternative way to reduce these process limitations is to apply a semi-solid extrusion process because it has several advantages such as the requirements of low extrusion force, good flowability, and less friction between the dies and the materials[3].
During the past several years, thixo-extrusion has been continuously developed since it is found to be able to achieve high quality products, for example, good surface finish and high ultimate tensile strength[4-10]. However, in a thixo-extrusion process, it is necessary to use a billet so only certain types of alloys can be processed and the scrap cannot be recycled on site[11]. From these restrictions, many research teams are focusing on the rheo-extrusion approach[12-16].
In previous studies on a rheo-extrusion process, FAN et al[12-13] performed experiments with twin screw rheo extrusion (TSRE) for magnesium alloys. The solid fractions produced in their work were more than 30% and the sleeve was kept at a high temperature of 630 °C and plunger speed was about 1 cm/s. The samples had uniform microstructure and quite high tensile strengths. LEE et al[14] investigated the behavior of melt extrusion for Al-Cu alloys. In the process, the molten Al-Cu alloy was held in a shot sleeve until the solid fraction was 78%. The slurry with the high solid fraction was then forced through the opening die at the plunger speed between 0.2 and 1.1 cm/s. The extrusion die was also kept at high temperatures of 500-520 °C. They reported that the high temperature of the die caused segregation of liquid phase. The way to solve this problem was to use lower die temperatures or to add some grain refiners.
In summary, from Refs.[12-14], most of the rheo-extrusions used high solid fractions to produce the extruded products, and therefore they needed to use low ram speed and high temperature of the dies because of the high friction between the die and the materials.
The goal of this research is to develop a new rheo-extrusion process that can be used with lower temperatures of the die and the sleeve. In addition, it should be able to be used with higher speeds than conventional extrusion. Therefore, the process focuses to use semi-solid slurry with low solid fractions. A recent work in rheocasting using low solid fractions is published by WANNASIN et al[15]. In their work, semi-solid die casting at low solid fractions was developed using the gas induce semi-solid (GISS) process. Good properties such as low porosity and improved tensile properties were reported in their work. Thus, in this present work, the GISS technique is selected to be used with the rheo-extrusion process. And a preliminary research and development work of a new rheo-extrusion process using semi-solid slurry with low solid fractions employing the GISS technique is reported.
2 Experimental
2.1 Semi-solid rheo-extrusion experiments
The raw material used in this work was aluminum 356 alloy or JIS AC4C. The chemical composition of the alloy is shown in Table 1.
Table 1 Chemical composition of aluminum 356 alloy (mass fraction, %)

In the experiments, the aluminum alloy was melted in an electric furnace at about 650 °C. Approximately 250 g of the molten aluminum was taken from the crucible by a ladle. When the temperature of the molten aluminum was about 620 °C, a graphite diffuser was immersed to produce the semi-solid slurry with the solid fractions of 5%, 10%, and 20%, respectively. A schematic drawing of the GISS technique is shown in Fig.1.
The semi-solid slurry from the GISS machine was then poured into a shot sleeve with the inner diameter of 40 mm. The shot sleeve was preheated to about 250 °C. Next, the slurry was forced by a plunger at various speeds of 2, 4, and 6 cm/s through a die, a graphite support and a water-cooled tube. The inner diameter of the die was 12 mm. The parameters used in this study are summarized in Table 2. The schematic drawing of this rheo-extrusion process is shown in Fig.2. The machine used in this study has a 80 t capacity with a hydraulic system.
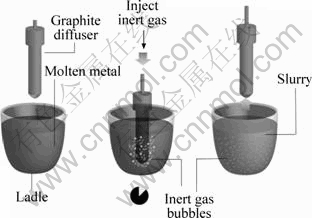
Fig.1 Schematic drawing of GISS technique
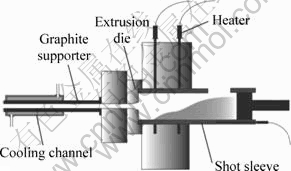
Fig.2 Schematic drawing of rheo-extrusion system
Table 2 Summary of parameters used in this study
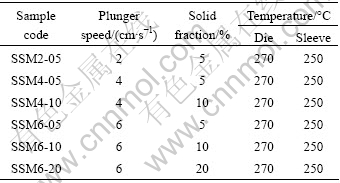
2.2 Extrudability evaluation
The extruded samples were analyzed by the measurement of the length to determine the extrudability. The length of the samples was measured after the extrusion test. In this work, the criterion for the required length was 20 cm. Shorter samples were rejected.
2.3 Microstructure observation
The microstructure of the samples was observed using an optical microscope. The samples were cut and obtained from three positions as shown in Fig.3. The samples were then prepared for metallographic analysis using the standard grinding, polishing and etching procedure. Good extruded parts should have uniform microstructure throughout the length and along the radius.
2.4 Mechanical properties measurement
The samples that pass the criterion of extrudability were tested for the ultimate tensile strength and elongation using the universal testing machine (UTM). The sample was prepared using the ASTM B557M-02a standard[17].
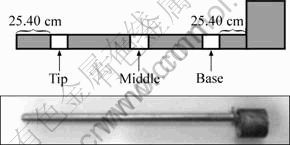
Fig.3 Sampling positions
3 Results and discussion
A representative extruded sample from the experiments is given in Fig.4. The results show that all the samples consist of unextruded, extruded and squeezed liquid sections. The samples produced by a lower plunger speed and at a higher solid fraction yield lower amount of squeezed liquid. These conditions tend to have less liquid segregation.
Observation of the surface shows that all the samples have a good surface finish in the extruded section as shown in Fig.5.
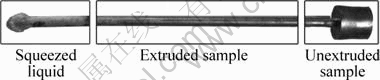
Fig.4 Components of representative rheo-extrusion sample
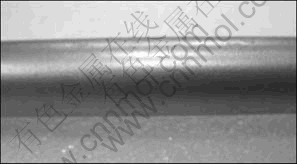
Fig.5 Surface finish of a representative sample
3.1 Extrudability
The samples produced by the conditions of 2, 4, and 6 cm/s plunger speed and 5% solid fraction have the lengths of 14, 23, and 24 cm, respectively. For 10% solid fraction, the samples produced by 4 and 6 cm/s plunger speed have the lengths of 18 and 23 cm, respectively. The sample produced by 20% solid fraction and 6 cm/s plunger speed has the length of 17 cm. The representative extruded samples from rheo-extrusion at different plunger speeds are shown in Fig.6.
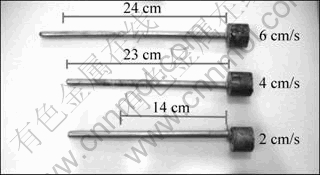
Fig.6 Samples from rheo-extrusion at different plunger speeds
The results suggest that conducting the rheo- extrusion with a higher speed (6 cm/s) and with the lower solid fraction (5%) gives the longest length as expected since the slurry can flow easier and faster. In addition, at a higher solid fraction, the plunger speed needs to be higher to yield the same extruded length because of the increased viscosity of the slurry. When the solid fraction was too high (20%), the extruded sample was short since the solidification time was shorter and the viscosity was higher.
In summary, only the samples produced by the conditions of 5% solid fraction at 4 and 6 cm/s plunger speeds and 10% solid fraction at 6 cm/s plunger speed pass the requirement of the length, as shown in Table 3.
Table 3 Semi-solid extrudability of A356 Al-alloy
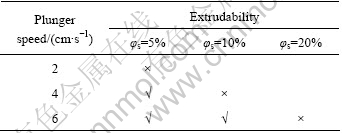
3.2 Microstructure uniformity
The microstructures at the center of the cross section of the three samples which pass the length criterion are shown in Fig.7. The micrographs show that the primary α structure (white structure) of all samples is quite similar and uniform in all the positions throughout the length. However, observation shows non-uniform microstructures in the radius direction in these samples. The edge of the samples (Fig.8) shows fine dendritic structure whereas the center of the samples shows semi-solid structure. The schematic drawing in Fig.8 illustrates the different structures at the edge and the center. The samples produced by the solid fractions of 5% and 10% have the length of liquid segregation zone of about 1.14 and 0.57 mm, respectively, as shown in Fig.9. It can be concluded that if the solid fraction increases, the liquid segregation around the outer perimeter will decrease.
The main result of using the starting slurry with low solid fractions is the lubricating liquid segregation zone near the surface of the samples. The amount of the liquid segregation can be controlled by the amount of the starting solid fractions. Although the higher liquid segregation helps to lubricate the extrusion process, it should not be too much because it will lead to non-uniform microstructure which may affect the mechanical properties.
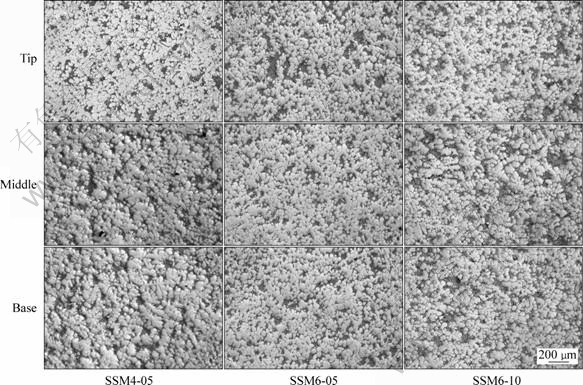
Fig.7 Representative microstructures of samples passing requirement of extrudability
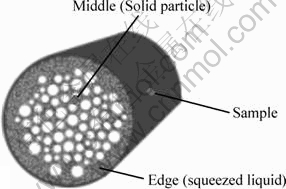
Fig.8 Schematic drawing showing liquid segregation in radius direction
From the microstructure uniformity study, it can be concluded that the microstructures are uniform in the length direction for all the conditions of the plunger speeds and solid fractions of the slurry. In the radius direction, higher solid fraction results in the reduction of the liquid layer, which helps to increase the uniformity of the microstructure, as shown in Fig.9.
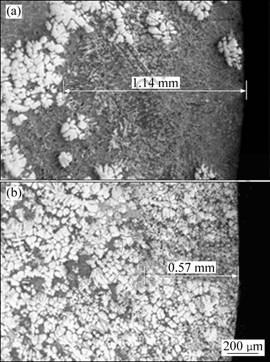
Fig.9 Representative microstructures at outer edge of samples at solid fractions of 5% (a) and 10% (b)
3.3 Mechanical Properties
The results of the mechanical property analysis report that the ultimate tensile strength and elongation of the as-extruded samples coded SSM4-05, SSM6-05 and SSM6-10 are 191.9 MPa, 190.3 MPa, 208.4 MPa and 7.5%, 6.5%, 11.2%, respectively. After T6 heat treatment, the ultimate tensile strength of samples is increased to 274.6 MPa, 255.3 MPa, 276.5 MPa and the elongation is increased to 8.3%, 7.3% and 12.4%, respectively. Fig.10 shows the ultimate tensile strength and elongation of the extruded samples before and after T6 heat treatment. The results show that the solid fraction and plunger speed do not significantly affect the tensile strength. In contrast, the results of the elongation indicate that the solid fraction affects the elongation. The elongation of the samples produced at 6 cm/s of plunger speed with 10% of solid fraction is higher than that of the samples produced at the same speed but at a lower solid fraction of 5%.
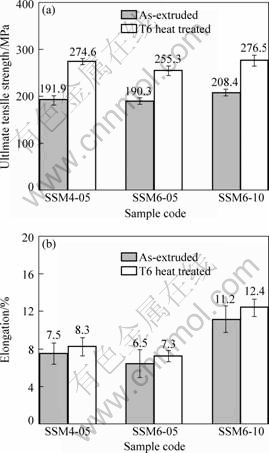
Fig.10 Ultimate tensile strength (a) and elongation (b) of extruded samples before and after T6 heat treatment
The higher elongation of the samples produced at a higher solid fraction may be the result of the lower liquid segregation in the radius direction as shown in Fig. 8 and Fig.9.
The comparison of the mechanical properties of the rheo-extruded 356 samples from this research and the typical extruded aluminum rod grade 6063 from ASM standard[16] is shown in Fig.11.
The results show that the rheo-extruded 356 samples have higher tensile strength than the 6063 alloy, and similar elongation property. Therefore, it can be concluded that the new rheo-extrusion process applying low solid fractions is a feasible process.
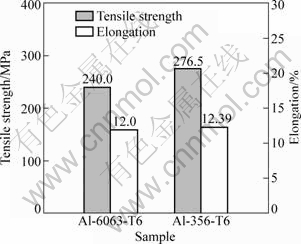
Fig.11 Comparison of mechanical properties of rheo-extruded 356 samples after T6 heat treatment from this research and typical extruded aluminum rod grade 6063 from ASM standard
4 Conclusions
1) This preliminary study shows the feasibility of a new rheo-extrusion process using low starting solid fractions processed by the GISS technique. Good extruded products can be achieved with the appropriate conditions of solid fractions and plunger speeds.
2) In this rheo-extrusion using low solid fractions of the slurry, there is a liquid layer at the outer perimeter of the samples. This phenomenon helps to lubricate the flow of the extruded part. Therefore, the process can be used with higher plunger speeds in the semi-solid state compared with conventional hot extrusion.
3) Uniform microstructure in the length direction of the samples can be achieved in all conditions of the solid fractions and plunger speeds.
4) The ultimate tensile strength (UTS) does not depend on the solid fractions of the slurry and the plunger speeds. The samples from this rheo-extrusion of 356 aluminum alloy have higher strengths compared with commercial 6063 aluminum alloy.
5) The elongation of the samples depends on the solid fraction of the slurry. The higher solid fraction yields more uniform microstructure in the radius direction so that the elongation is improved.
Acknowledgements
The authors gratefully acknowledge the financial supports from Prince of Songkla University (Contract number AGR530031M) and the Thai Research Fund (Contract number MRG5280215). We also thank the Department of Mining and Materials Engineering, Faculty of Engineering, Prince of Songkla University for the facilities and the Innovative Metal Technology (IMT) team for all the kind supports.
References
[1] PEARSON C, PARKINS R. The extrusion of metal [M]. London: Chapman & Hall Ltd, 1960: 223-270.
[2] KALPAKJIAN S, SCHMID S R. Manufacturing processes for engineering materials [M]. New Jersey: Prentise Hall, 2008: 307-319.
[3] FLEMINGS M C. Behavior of metal alloys in the semi-solid [J]. Metallurgical Transactions A, 1991, 22A: 957-981.
[4] BAYOUMI M A, NEGM M I, EL-GOHRY A M. Microstructure and mechanical properties of extruded Al-Si alloy (A356) in the semi-solid state [J]. Materials and Design, 2009, 30: 4469-4477.
[5] JANG D I, YOON Y O, KIM S K. Thixoextrusion for 7075 Al wrought alloy tube [J]. Solid State Phenomena, 2008, 141/142/143: 267-270.
[6] MORADI M, NILI-AHMADABADI M, HEIDARIAN B, ASHOURI S. Investigation of thin wall casting made of semi-solid A356 using back extrusion and die cast [J]. Solid State Phenomena, 2008, 141/142/143: 67-72.
[7] WANG K, ZHANG P, DU Y, ZENG P, LI H. Basic study on thixo-co-extrusion of multi-layer tube with Al/Mg alloys [J]. Solid State Phenomena, 2008, 141/142/143: 73-78.
[8] SUGIYAMA S, LI J, YANAGIMOTO J. Semi-solid state extrusion of plain carbon steel [C]//The 8th International Conference on Semi-solid processing of Alloys and Composites. Limassol, Cyprus, 2004.
[9] MIWA K, KAWAMURA S. Semi-solid extrusion forming process of stainless steel [C]//The 6th International Conference on Semi-solid processing of Alloys and Composites. Turin, Italy, 2000: 279-281.
[10] KOPP R, KALLWEIT J, MOLLER T H. Thixoforming of steel- experiments with a vacuum chamber based tool concept [C]//The 6th International Conference on Semi-solid processing of Alloys and Composites. Turin, Italy, 2000: 599-604.
[11] JORSTAD J L, THIEMAN M, KAMM R. Fundamental requirement for slurry generation in the sub liquidus casting process and the economics of SLCTM Processing [C]//The 8th International Conference on Semi-solid processing of Alloys and Composites. Limassol, Cyprus, 2004.
[12] XU J, ZHANG S, YANG B, SHI S, FAN Z. Microstructure and mechanical properties of rheo-extruded AZ31Mg-alloy [J]. Solid State Phenomena, 2008, 141/142/143: 713-718.
[13] JI S, FAN Z, BEVIS M J. Semi-solid processing of engineering alloys by a twin screw rheomoulding process [J]. Materials Science and Engineering A, 2001, 402: 170-176.
[14] LEE B S, JOO D H, KIM M H. Extrusion behavior of Al-Cu alloys in the semi-solid state [J]. Materials Science and Engineering A, 2005, 402: 170-176.
[15] WANNASIN J, JANUDOM S, RATTANOCHAIKUL R, FLEMINGS M C. Development of the gas induced semi-solid metal process for aluminum die casting application [J]. Solid State Phenomena, 2008, 141/142/143: 97-102.
[16] HATCH J E. Aluminum properties and physical metallurgy [M]. Ohio: American Society for Metals(ASM), 1984: 363.
[17] ASTM B557M–02a. Standard test methods of tension testing wrought and cast aluminum-and magnesium-alloy product[Metric] [M]. Pennsylvania: ASTM International, 2003: 1-15.
(Edited by YANG Bing)
Corresponding author: J. WANNASIN; Tel: +66-74-287-312; E-mail: jessada.w@psu.ac.th, jessada@alum.mit.edu
DOI: 10.1016/S1003-6326(09)60371-X